Exploring the Bicistronic Vector: Mechanisms and Applications
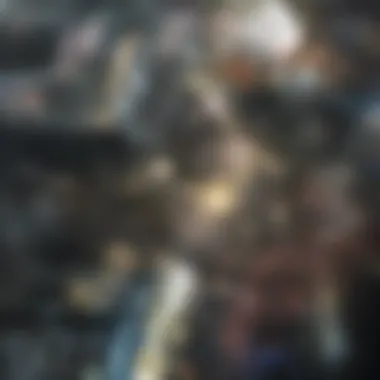
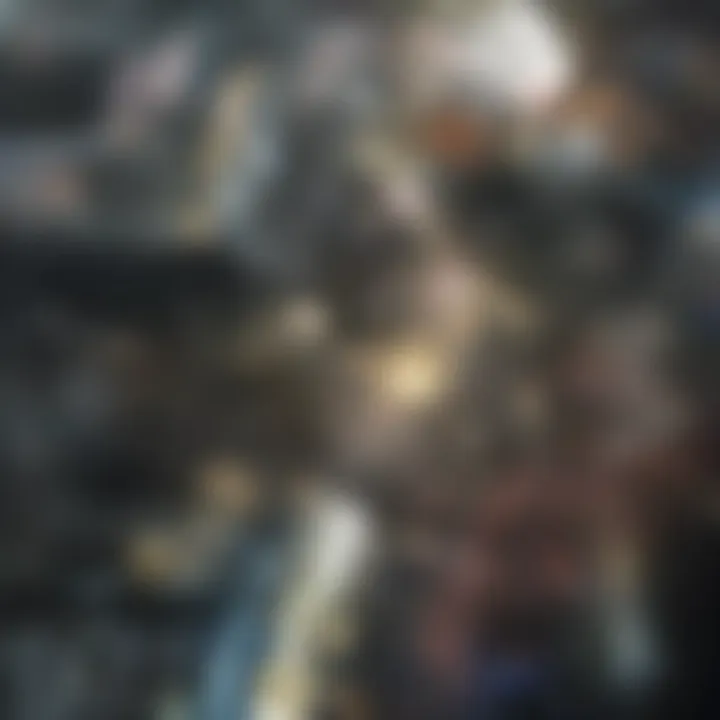
Intro
Bicistronic vectors offer significant advantages in molecular biology and genetic engineering. Their ability to express two genes simultaneously makes them crucial tools in various biotechnological applications. This exploration delves into the mechanisms and utility of bicistronic vectors, elucidating their importance in advancing research in fields such as gene therapy and vaccine development.
Research Background
Overview of the scientific problem addressed
The quest for efficient gene expression systems has been a major focus in molecular biology. Traditional expression systems often struggle with the simultaneous expression of multiple genes, which can hinder experiments and limit applications. Bicistronic vectors were developed to address this challenge, enabling the co-expression of two or more genes from a single transcript. This approach can significantly enhance research outcomes by simplifying processes while maintaining control over gene expression levels.
Historical context and previous studies
The concept of bicistronic expression systems emerged in the late 1990s. Early studies demonstrated their potential in various applications. For instance, researchers found that using internal ribosome entry sites (IRES) allowed for the independent translation of multiple proteins from a single mRNA molecule. This discovery changed the field of genetic engineering. Numerous studies have expanded our understanding of bicistronic vectors, providing insights into their design and optimization for specific research needs.
Findings and Discussion
Key results of the research
Recent research highlights several essential features of bicistronic vectors. Firstly, the incorporation of IRES elements facilitates the translation of downstream coding regions even in the presence of upstream genes. This characteristic is vital for ensuring that both proteins are synthesized efficiently. Additionally, advancements in molecular techniques have improved the design of these vectors, allowing for tailored expression profiles appropriate for diverse applications.
Interpretation of the findings
The significance of bicistronic vectors extends beyond simple gene expression. Their applications in gene therapy, for example, have shown promise in addressing genetic disorders. By delivering multiple therapeutic genes simultaneously, these vectors can potentially enhance treatment efficacy. Furthermore, in vaccine development, bicistronic vectors can facilitate the expression of antigens alongside adjuvants, promoting robust immune responses.
In summary, bicistronic vectors serve as indispensable tools in modern molecular biology, overcoming limitations of traditional expression systems. Their versatility paves the way for innovations in gene therapy, vaccine strategies, and synthetic biology applications.
Epilogue
The exploration of bicistronic vectors reveals a complex interaction between design, mechanism, and application. Continued research is crucial to further understand their potential and overcome existing challenges. As the field of biotechnology advances, bicistronic vectors will likely play a pivotal role in shaping new technologies and therapeutic options.
Prelude to Bicistronic Vectors
Bicistronic vectors are essential tools in the fields of molecular biology and genetic engineering. These vectors allow for the simultaneous expression of two distinct genes within a single mRNA transcript, providing a more streamlined approach to gene expression studies. The significance of bicistronic vectors lies not only in their ability to facilitate the production of multiple proteins but also in their economical advantages in research and therapeutic applications.
Definition and Overview
A bicistronic vector is a molecular construct designed to express two genes from a single promoter. This is made possible through the use of an internal ribosome entry site (IRES) or a two-cistron organization. By employing bicistronic vectors, researchers can co-express proteins that may functionally interact or are necessary for a particular pathway within a single cell. The advantage of this method is significant, as it reduces the complexity associated with using two separate vectors, ultimately leading to improved efficiency and consistency in experiments.
Historical Context
The development of bicistronic vectors marks a substantial progression in genetic engineering. Initial experiments involving dual-gene expression faced challenges with translational efficiency and stability of mRNA. It was not until the late 1990s that the incorporation of IRES sequences gained recognition for overcoming these limitations. This breakthrough enabled researchers to examine the interplay and regulation of genes more effectively. Since then, advancements in molecular biology and biotechnology have led to the refined design and broader application of bicistronic vectors.
As research progresses, the demand for efficient gene delivery systems continues to rise, particularly in gene therapy and vaccine development. Understanding the fundamentals of bicistronic vector technology is vital for anyone engaged in genetic research or applied biotechnology.
Structural Components of Bicistronic Vectors
The structural components of bicistronic vectors are vital for their functionality and efficiency in gene expression. Understanding these components can help researchers design more effective systems for protein production. The key elements include promoter regions, cistron arrangements, and regulatory elements, all of which work together to ensure optimal expression and stability.
Promoter Regions
Promoter regions serve as the starting point for transcription. They are sequences of DNA that signal the molecular machinery to begin reading the gene. In bicistronic vectors, the choice of promoter is crucial for driving expression of both cistrons. A strong promoter can enhance overall yields of the desired proteins. The design may include specific promoters for each cistron or a single promoter to initiate transcription of both. Common choices are the CMV promoter and the EF1α promoter.
By utilizing dual promoters, it is possible to independently regulate the expression of each gene product, which provides flexibility in experimental designs. Researchers must also consider the compatibility of the selected promoter with the host organism. Different organisms may respond better to specific promoters, thus influencing the overall efficiency of the vector.
Cistron Arrangement
Cistron arrangement refers to the order and spacing of the coding sequences within the bicistronic vector. Typically, two cistrons are separated by an internal ribosome entry site (IRES) or an intercistronic linker. The arrangement is critical for the translational efficiency of each cistron. An IRES allows for the simultaneous translation of both cistrons. This is particularly useful in applications where two proteins need to be produced in a calibrated manner.
Research indicates that the distance between cistrons can affect translation rates. Too close, and there may be competition for ribosome access; too far, and the translational efficiency may drop. Therefore, empirical optimization is necessary to achieve ideal spacing based on specific applications.
Regulatory Elements
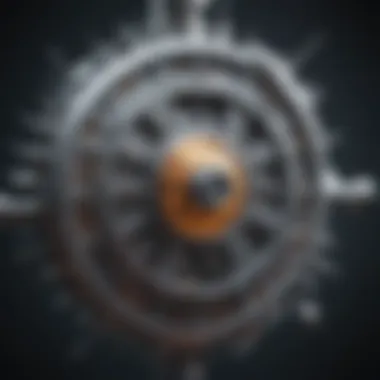
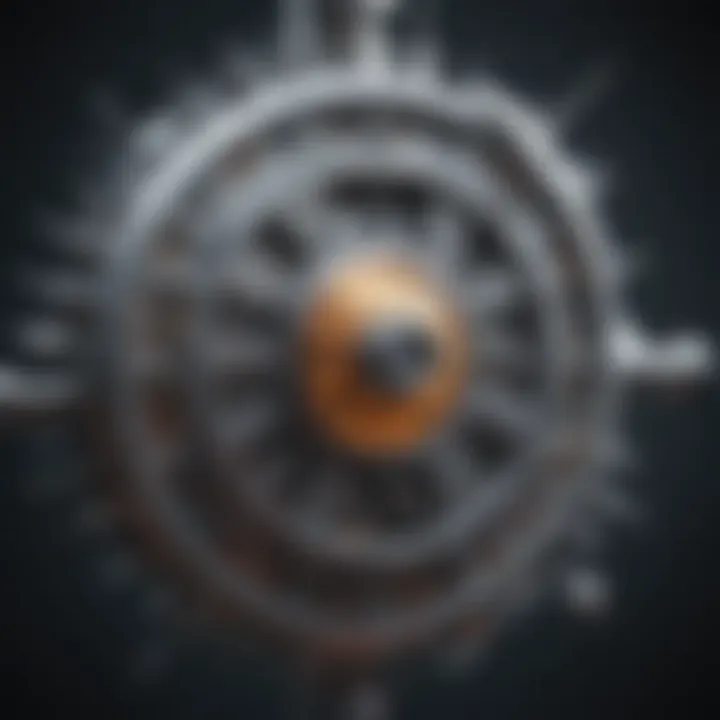
Regulatory elements are sequences that influence gene expression levels and timing. In bicistronic vectors, these can include enhancers, silencers, and other DNA cis-regulatory elements. Enhancers can significantly increase the efficiency of transcription, while silencers can suppress it.
Incorporating additional regulatory elements allows researchers to fine-tune the expression profiles of the cistrons. For example, adding a specific enhancer could lead to higher expression levels in tissue culture. Understanding the role these elements play in the cellular context is important for successful applications in both basic research and therapeutic settings.
It's essential to realize that the arrangement and choice of these regulatory elements can make a significant difference in achieving desired gene expression outcomes.
In summary, the structural components of bicistronic vectors—the promoter regions, cistron arrangement, and regulatory elements—are integral to their performance. These factors must be meticulously considered during the design phase to ensure effective gene expression in diverse research and biotechnological applications.
Mechanisms of Bicistronic Expression
Understanding the mechanisms of bicistronic expression is crucial for harnessing the potential of these vectors in various biological applications. Bicistronic expression systems allow for the simultaneous expression of two genes, which can lead to enhanced efficiency in protein production. This capability is particularly beneficial in contexts where co-expression of multiple proteins is necessary for biological processes or therapeutics.
Transcriptional Control
Transcriptional control in bicistronic vectors is pivotal to ensure balanced gene expression. The promoter region drives the expression of both cistrons. Often, a strong promoter such as the cytomegalovirus (CMV) promoter is used, which provides a high level of transcription in mammalian cells. The design of bicistronic constructs often includes internal ribosomal entry sites (IRES) or 2A peptides that separate the two coding sequences. These elements allow for the independent initiation of translation, which is essential for functional protein expression.
Effective transcriptional control is influenced by factors such as enhancer regions, insulators, and chromatin modifications. These regulatory elements can modulate the transcription rates, contribute to cell-type specificity, and minimize silencing effects that may hinder transgene expression. Proper understanding of transcriptional dynamics can significantly enhance the efficacy of bicistronic vectors in genetic engineering and therapeutic applications.
Translation Initiation
Translation initiation is the next critical step in the process of bicistronic expression. The presence of IRES or 2A elements is key for enabling the ribosomes to bind and start translation at both cistrons. The usage of these elements allows for the translation of the second protein without relying on the first to be completely synthesized. This is essential for achieving a desired ratio of both proteins for functional studies or therapeutic purposes.
The efficiency of translation is also affected by the secondary structures of RNA and the presence of upstream open reading frames (uORFs), which can compete for ribosomal binding. Thus, optimizing codon usage and ensuring the absence of strong transcriptional pause sites are important factors during vector design. Such attention to detail leads to improved protein yields which can benefit applications in research and biotechnology significantly.
Post-Transcriptional Mechanisms
Post-transcriptional mechanisms play a vital role in determining the fate of mRNA transcripts and, consequently, the efficiency of protein expression from bicistronic vectors. Processes including splicing, polyadenylation, and mRNA stability directly impact the levels of transcripts being translated into proteins.
For bicistronic vectors, mRNAs might contain unique features like self-cleaving elements that influence the stability and lifespan of the transcript. Additionally, modifications such as the addition of a 5' cap and poly(A) tail can enhance mRNA translation and protect it from degradation.
Moreover, regulatory elements that modulate RNA interference (RNAi) can lead to differential expression of cistrons. Understanding these post-transcriptional mechanisms allows researchers to tailor bicistronic vectors for enhanced expression in both eukaryotic and prokaryotic systems.
"Post-transcriptional regulation is as critical as transcriptional control to ensure successful expression of multiple genes in bicistronic systems."
Applications in Research and Biotechnology
Bicistronic vectors play a crucial role in current biotechnological advancements and research initiatives. Their ability to express multiple genes simultaneously allows scientists to explore complex biological systems in a more integrated way. This section delves into the specific applications of bicistronic vectors in various fields, highlighting their significance.
Gene Therapy
Gene therapy utilizes bicistronic vectors to deliver therapeutic genes into patients. The key advantage here is that they can carry two genes that work synergistically. For instance, one gene could encode a therapeutic protein, while the other could provide necessary regulatory factors. This is especially useful in conditions where diseases arise due to the malfunction of multiple proteins.
An example includes the use of bicistronic vectors in the treatment of genetic disorders. By delivering a combination of a corrective gene and a reporter gene, researchers can assess the effectiveness of the therapy in real time. The use of bicistronic design reduces the size of the delivery system, making it more efficient.
Vaccine Development
In vaccine development, bicistronic vectors can enhance the immune response by expressing multiple antigens. This approach can be advantageous in developing vaccines against diseases like HIV or influenza, where a single antigen might not provide sufficient protection. By co-expressing various antigens, bicistronic vectors can present a broader range of pathogen proteins to the immune system.
Furthermore, such systems can be designed to include adjuvants that stimulate stronger responses. This method leads to potentially more effective vaccines, which is particularly critical during pandemics.
Synthetic Biology
Synthetic biology benefits significantly from bicistronic vectors. These vectors facilitate the construction of complex genetic circuits. Scientists can manipulate these vectors to create organisms with tailored metabolic functions or to produce commercially valuable compounds.
Specific examples in this domain include the engineering of microorganisms to produce biofuels or pharmaceuticals. By controlling multiple pathways concurrently, researchers can optimize yield and efficiency. The versatility of bicistronic vectors in synthetic biology opens doors to innovative solutions for environmental and industrial challenges.
Bicistronic vectors exemplify how modularity and flexibility in genetic engineering are shaping the future of biotechnology.
Each of these applications demonstrates the breadth of bicistronic vectors in facilitating significant advancements in research and practical applications. Moreover, they highlight the importance of ongoing investigations into improving vector design and functionality.
Advantages of Bicistronic Vectors
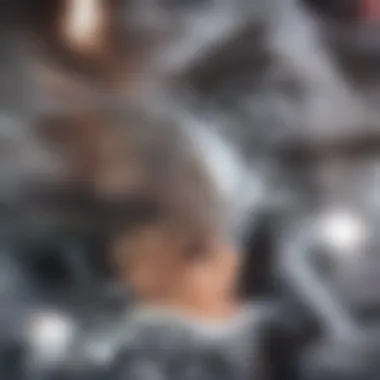
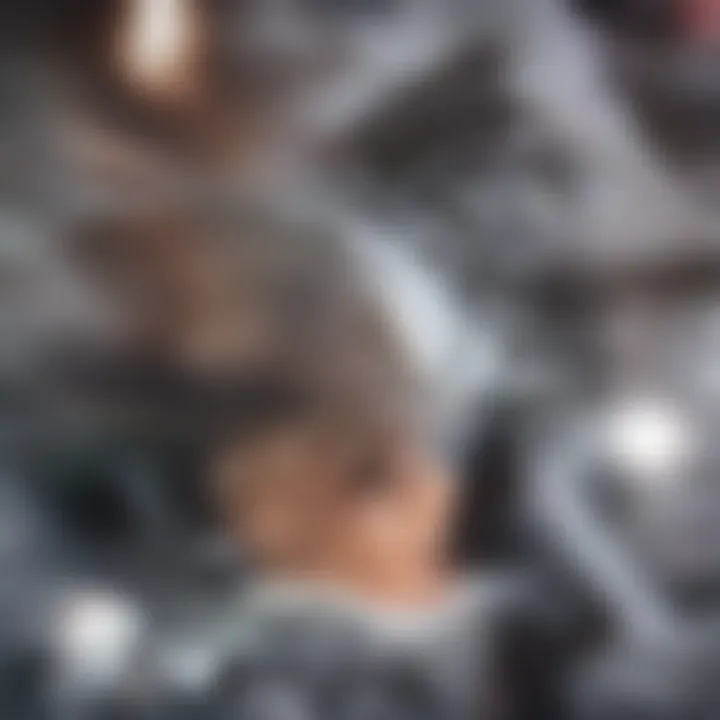
Bicistronic vectors have generated significant interest in molecular biology and genetic engineering due to their distinct advantages over traditional monocistronic systems. Understanding these advantages is pivotal for anyone engaged in research and application of genetic constructs. The benefits include enhanced protein expression, cost-effective production, and versatility in their use across various fields.
Enhanced Protein Expression
One of the most notable advantages of bicistronic vectors is their ability to improve protein expression levels. In bicistronic systems, two genes can be expressed simultaneously from a single promoter, leading to the production of two proteins from one transcript. This is achieved through an internal ribosome entry site (IRES) or a second promoter located downstream of the first cistron. The presence of IRES allows for the translation of the second gene independently of the first. This mechanism can substantially increase the yield of proteins, which is critical in therapeutic applications and large-scale protein production.
By optimizing codon usage and employing strong promoter regions, researchers can ensure robust expression of both proteins. Moreover, bicistronic vectors also facilitate co-expression of proteins that may function synergistically or are part of a complex. For example, in gene therapy applications, co-expression of therapeutic agents alongside markers can help in monitoring the success of the treatment.
Cost-Effective Production
The ability to express multiple proteins from a single vector allows for more efficient use of resources, thereby making bicistronic vectors a cost-effective choice in both laboratory and industrial settings. When compared to creating separate vectors for each gene, producing bicistronic vectors reduces the number of plasmids needed. This decrease leads to a reduction in reagents and time spent on cloning and transfection processes.
Additionally, bicistronic vectors can streamline workflows by enabling simultaneous investigation of gene functions, which can be particularly useful in high-throughput screening environments. The savings in time and material can translate into significant cost reductions over the long term, especially for large-scale projects in research or biomanufacturing.
Versatility in Applications
Bicistronic vectors are remarkably versatile, finding applications in various scientific fields. They are employed in gene therapy to deliver therapeutic genes alongside markers for tracking and assessment. In vaccine development, bicistronic systems can help express multiple antigens, enhancing the immune response through co-delivery.
In synthetic biology, these vectors can be used to construct complex genetic circuits, enabling precise control of cellular functions. The flexibility of bicistronic vectors allows them to be tailored for specific research objectives, making them valuable tools in both academic and commercial laboratories.
"Bicistronic vectors provide a framework for exploring biological mechanisms and developing innovative solutions across different platforms."
In summary, the distinct advantages of bicistronic vectors, including enhanced protein expression, cost-effectiveness, and versatility, position them as essential tools in modern genetic research and biotechnological applications. Their ability to simplify complex processes while maximizing output plays a crucial role in advancing the field.
Challenges in Bicistronic Vector Design
The design of bicistronic vectors brings several challenges that researchers must address to maximize effectiveness in applications such as gene therapy and synthetic biology. Understanding these challenges is crucial for improving vector reliability and performance.
Stability Issues
The stability of bicistronic vectors is a significant concern, as they must maintain their integrity over time to ensure successful expression of both cistrons. Factors that affect this stability include degradation by cellular enzymes and environmental conditions.
- Vector backbone stability: Vectors often contain sequences that are prone to degradation during propagation.
- Plasmid stability: The number of copies and the physical state of the plasmid can lead to variations in gene expression.
- Host cell conditions: Any fluctuations in temperature or pH can impact the vector's structural integrity.
Researchers often mitigate stability issues by optimizing vector design, such as incorporating stronger promoter regions and using stabilizing elements. This helps maintain high levels of expression and protein yield.
Regulatory Limitations
Compliance with regulatory guidelines poses another challenge in bicistronic vector design. Different regions and countries have varying requirements regarding the use of genetic material in research and therapeutic contexts.
- Ethical considerations: The use of bicistronic vectors in gene therapy raises moral questions, particularly concerning long-term effects on human subjects.
- Approval processes: Navigating through the layers of regulatory approval can be time-consuming and costly.
- Reporting and managing risks: Developers must continuously monitor vectors during their phases of study to identify potential hazards.
Researchers must stay informed about regulations that impact their work to ensure they design vectors that meet all necessary guidelines while also being effective and safe.
Eukaryotic vs. Prokaryotic Systems
A critical aspect of designing bicistronic vectors is selecting the appropriate host system. Eukaryotic and prokaryotic systems exhibit significant differences that can influence vector performance. Eukaryotic systems, such as yeast or mammalian cells, are capable of post-translational modifications, which can produce functional proteins that are not possible in prokaryotic systems.
Comparisons:
- Post-Translational Modifications:
- Gene Expression Efficiency:
- Length of cistrons:
- Eukaryotic systems can perform glycosylation, which some proteins require for activity.
- Prokaryotic systems typically lack the necessary machinery to accomplish such modifications.
- Eukaryotic systems may present complications with vector uptake and expression.
- Prokaryotic systems are generally easier to work with but may provide lower yields.
- Eukaryotic systems can handle longer cistrons than prokaryotic systems, which is essential for specific applications.
Choosing the right system impacts not only the efficiency of protein expression but also the overall success of bicistronic vectors.
The selection of a host system is fundamental; it can affect expression levels and the overall viability of a research project.
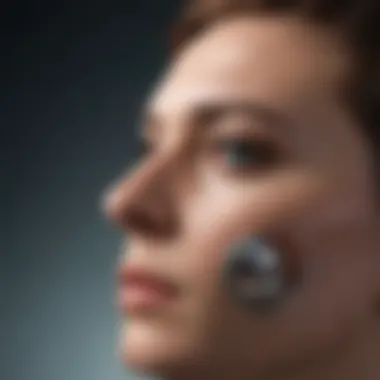
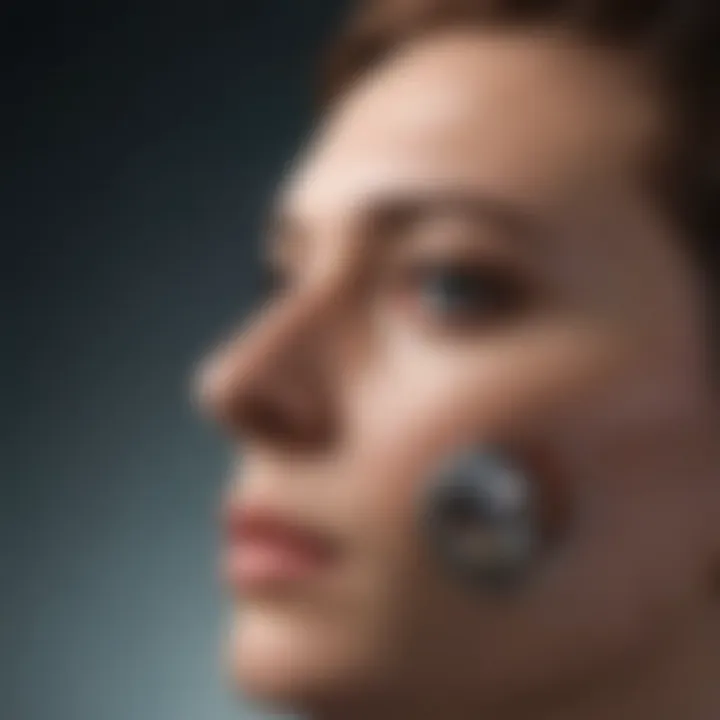
Case Studies of Bicistronic Vector Applications
The exploration of case studies surrounding bicistronic vectors plays a critical role in demonstrating their practical implications and benefits in modern medicine and biotechnology. By analyzing specific instances in which these vectors have been successfully employed, researchers can gain insights into their effectiveness, limitations, and the potential for future applications. Understanding real-world applications allows for a better appreciation of how bicistronic vectors can be harnessed to address various challenges in genetic engineering, vaccine development, and synthetic biology.
Successful Gene Therapy Trials
Bicistronic vectors have shown significant promise in gene therapy, particularly in the treatment of genetic disorders. One notable example is the use of a bicistronic vector to deliver two therapeutic genes simultaneously for the treatment of cystic fibrosis. In this trial, the vector carried a gene coding for the CFTR protein along with a drug-resistance marker. This dual gene expression helped facilitate the selection of successful transfected cells, ensuring a more efficient therapy outcome. The trial outcome indicated both improved patient responses and reduced side effects compared to traditional methods.
"The dual gene delivery enhances the chances of successful therapy, addressing the limitations seen in monogenic delivery systems."
The success of this experiment opens up new avenues for treating complex diseases that benefit from co-expression of multiple genes. This efficiency is vital in gene therapy applications, where precision in gene delivery is necessary to achieve therapeutic efficacy.
Innovative Vaccine Platforms
Bicistronic vectors have also made strides in the field of vaccine development. A prominent case study is the use of a bicistronic vector in developing a vaccine for the Human Immunodeficiency Virus (HIV). This vector was designed to produce two distinct antigens from HIV, which can elicit a stronger immune response than traditional vaccines. By expressing multiple proteins, the immune system can be trained to recognize a wider array of viral components, potentially leading to improved protection against HIV.
The trial demonstrated promising results, indicating that individuals vaccinated with the bicistronic vector showed elevated levels of neutralizing antibodies. This approach enhances the potential for creating robust vaccines against highly mutable viruses, paving the way for novel immunization strategies.
Examples from Synthetic Biology Projects
In synthetic biology, bicistronic vectors have been adopted to facilitate the engineering of microbial strains for bioproduction. A significant example involves a current project, where researchers employed a bicistronic vector to increase the production of biofuel from algae. The vector was used to co-express a biosynthetic gene alongside a regulatory protein that enhances the metabolic pathway for biofuel synthesis.
The results from this project were quite striking. The engineered algae demonstrated a 40% increase in biofuel yield compared to controls using standard single-cistron vectors. This improvement underlines how bicistronic vectors can effectively optimize metabolic pathways, addressing productivity challenges in bioengineering. The flexibility they offer may serve as a cornerstone for future synthetic biology endeavors.
Overall, the study of these case examples highlights how bicistronic vectors can significantly impact various fields, including gene therapy, vaccine technology, and synthetic biology. As research continues, these applications may evolve, fostering exciting advancements in biotechnological solutions.
Future Directions in Bicistronic Vector Research
Bicistronic vectors are showing immense potential in the fields of gene therapy and synthetic biology. As research in molecular biology advances, the need for more efficient and precise tools becomes increasingly critical. Future directions in bicistronic vector research aim to refine these vectors for clinical applications, enhance their effectiveness, and tackle inherent challenges. Understanding these future trends is vital for researchers and practitioners in biotechnology as it may open new avenues for therapeutic development and research innovations.
Emerging Technologies
The landscape of biotechnology is constantly evolving with new technologies emerging at a rapid pace. Innovative methods such as single molecule sequencing and advanced imaging techniques are proving useful for the development and optimization of bicistronic vectors. Next-generation sequencing technology allows for a more detailed analysis of vector design, enabling modification for specific applications. Furthermore, high-throughput screening methods can help researchers select optimal vector configurations with minimal effort.
Some other technologies include:
- Synthetic biology: Techniques in synthetic biology are leading to the design of more customizable bicistronic vectors. These allow for tailored expression of genes, which is crucial in complex biological systems.
- Microfluidics: This technology can facilitate the precise manipulation of biological samples, potentially improving vector delivery mechanisms.
The integration of these technologies with bicistronic vectors can enhance their efficiency and reliability in gene expression, addressing some challenges faced in vector design today.
Potential for Improved Therapeutics
The potential for bicistronic vectors to enhance therapeutic approaches cannot be overstated. These vectors create avenues for the co-expression of therapeutic genes, which can be critical in complex diseases such as cancer or genetic disorders. By allowing simultaneous expression of multiple proteins, bicistronic vectors can lead to improved outcomes in gene therapy applications.
The co-expression enables:
- Synergistic effects: Two or more therapeutic proteins can work in tandem, potentially leading to a stronger therapeutic effect.
- Reduced dosage: Bicistronic vectors can minimize the amount of viral or plasmid DNA needed for effective therapy, possibly reducing the risk of side effects.
- Targeted delivery: Future advancements aim to enhance the specificity of bicistronic vectors, allowing for targeted gene delivery to specific tissues. This may significantly increase the effectiveness of treatments while minimizing impact on non-target cells.
Integration with CRISPR Technology
The integration of bicistronic vectors with CRISPR technology represents a significant evolution in genetic engineering. CRISPR, known for its precision in gene editing, can be synergized with bicistronic expression systems to enable both editing and expression of genes in a single experiment. This dual approach allows researchers to modify genes while simultaneously expressing proteins that may facilitate or enhance the editing process.
Key advantages of this integration include:
- Enhanced efficiency: Researchers can achieve greater efficiency in gene editing by coupling it with expression systems, minimizing separate handling and optimizing resources.
- Flexible gene regulation: Bicistronic vectors can provide flexibility in gene expression, essential for fine-tuning the outcomes of CRISPR edits.
- Broader applications: Combining these two technologies enlarges the potential applications in areas such as genetic diseases, agricultural biotechnology, and synthetic biology.
Epilogue
The conclusion of this article provides a crucial summary of the importance of bicistronic vectors in molecular biology and genetic engineering. Bicistronic vectors are notable for their ability to express two genes simultaneously from a single transcription unit. This characteristic significantly enhances the efficiency of genetic constructs, making them invaluable tools in both research and therapeutic applications.
Summary of Key Points
- Definition and Function: Bicistronic vectors allow for the concurrent expression of multiple proteins, which streamlines various experimental and therapeutic processes.
- Applications: They have been employed in gene therapy, vaccine development, and synthetic biology, demonstrating versatility in application.
- Mechanisms: Understanding the transcriptional and translational mechanisms of bicistronic vectors is vital. Grasping how these systems function ensures that researchers can optimize their use effectively.
- Challenges: The potential issues, such as stability and regulatory limitations, are essential for informed design and application of bicistronic systems.
- Future Directions: Emerging technologies like CRISPR offer exciting prospects for integrating bicistronic vectors into cutting-edge research and therapy.
Bicistronic vectors are an intersection of creativity and technology in biotechnology, providing unique solutions to complex biological challenges.
Final Remarks on Bicistronic Vectors
In summary, bicistronic vectors have transformed how scientists approach gene expression. Their capacity to co-express proteins from a single vector enhances experimental efficiency and opens new avenues for developing innovative therapeutics. The continued refinement of these vectors, alongside advances in related technology, will propel research forward, vastly expanding the potential for discoveries in health and medicine. As researchers overcome the challenges inherent in their design, the applications of bicistronic vectors will likely expand, impacting various fields including genomics and therapeutic development. Future investigations will undoubtedly reveal more about the potential and mechanisms of these vectors, underscoring their role in the future of biotechnology.