Exploring the Realm of Chemical Biology: A Comprehensive Overview
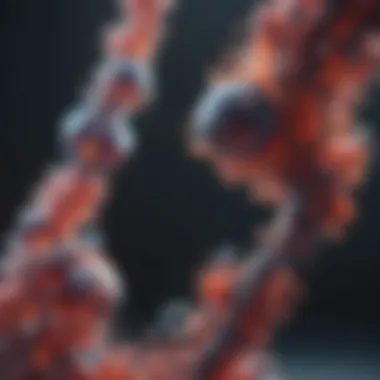
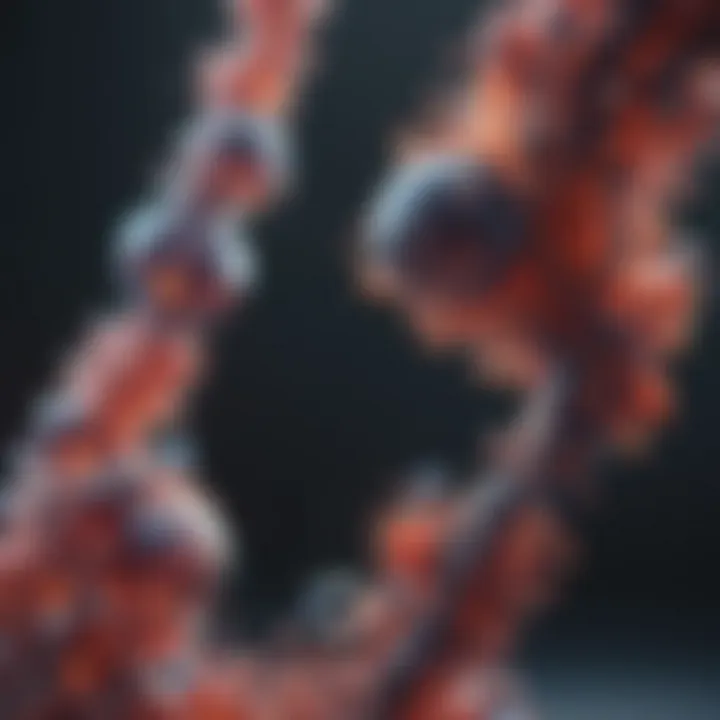
Intro
Chemical biology stands at the intersection of chemistry and biology, playing a critical role in our understanding of life at a molecular level. This discipline focuses on the development and application of chemical tools to study and manipulate biological systems. As we dive deeper into this subject, it becomes evident that chemical biology encompasses a wide array of techniques, ranging from small molecule synthesis to high-throughput screening of compounds. The impact of this field is vast, making it essential for research and development in drug discovery, genetic engineering, and various other applications.
Research Background
Overview of the scientific problem addressed
One of the primary challenges that chemical biology seeks to address is the complexity of biological systems. Living organisms operate through intricate networks of biochemical reactions and interactions. Understanding these systems is crucial for identifying how diseases develop and how they can be treated effectively. With the rise of personalized medicine, the need for tailored therapies that target specific biological pathways has never been more pressing. Chemical biology provides the tools necessary for this fine-tuned approach, enabling researchers to design compounds that can influence specific biological functions.
Historical context and previous studies
The roots of chemical biology can be traced back to the early days of modern chemistry and biology. Historical milestones include the discovery of penicillin, which exemplified how a chemical compound can profoundly alter biological pathways and treat infectious diseases. In the decades that followed, significant advancements in molecular biology, including the discovery of DNA's structure, have laid the groundwork for contemporary chemical biology. Pioneering studies by scientists such as Francis Crick and James Watson, and later by researchers like James D. Watson, helped to bridge the gap between these two disciplines, paving the way for innovative research.
As we examine the evolution of chemical biology, it becomes clear that the field has continuously adapted, integrating new technologies such as high-throughput sequencing and computational biology. This evolution has led to a better grasp of complex biological processes, enabling targeted therapeutic strategies.
In summary, the exploration of chemical biology is essential for advancing our understanding of life sciences. The interplay between chemistry and biology has opened new avenues for research and application, shaping the future of medicine, environmental science, and biotechnology.
Defining Chemical Biology
Chemical biology stands at the intersection of chemistry and biology, facilitating a deeper understanding of living systems through a chemical lens. It is essential to recognize how this field bridges the gap between two traditionally separate disciplines. The integration of chemical principles with biological inquiry allows scientists to explore the molecular basis of life. The implications of this synergy are vast, ranging from drug design to the development of new materials. In this section, we will delve into the historical background of chemical biology, as well as its key concepts that form its backbone.
Historical Perspective
The inception of chemical biology can be traced back to the early 20th century when scientists began to systematically study the chemical foundations of biological processes. Early pioneers, such as Hermann Emil Fischer, made significant contributions by elucidating the structure of biomolecules. The introduction of biochemistry as a distinct field laid the groundwork for what we now recognize as chemical biology.
In the latter half of the century, advances in technology propelled this discipline forward. Techniques such as chromatography and mass spectrometry allowed for better analysis of complex biological molecules. The synthesis of DNA in the 1980s was a watershed moment that demonstrated the power of chemical approaches to manipulate biological systems. Today, chemical biology encompasses a broad range of techniques, enabling researchers to tackle complex biological problems.
Key Concepts
At its core, chemical biology revolves around several key concepts that are crucial for understanding its scope and applications:
- Molecular Recognition: This concept focuses on how molecules interact with each other in biological systems. Understanding these interactions is fundamental for drug development and targeting specific proteins.
- Chemical Probes: These are small molecules used to investigate biological pathways. They help in identifying the function of biologically active compounds, contributing to research in various fields.
- Engineering Biological Systems: Chemical biology often employs synthetic biology techniques to create or modify biological systems. This improves our ability to develop new therapeutic strategies and biotechnologies.
- High-Throughput Techniques: These methodologies enable the analysis of thousands of samples simultaneously. High-throughput screening, for example, accelerates the identification of potential drug candidates and biomolecular interactions.
By embracing these foundational concepts, researchers can harness the principles of chemistry to further biological understanding. Chemical biology not only illuminates the inner workings of organisms but also paves the way for innovative solutions to pressing scientific challenges.
Importance in Modern Science
Chemical biology plays a pivotal role in contemporary scientific inquiry. This field combines diverse principles from chemistry and biology, resulting in an interdisciplinary approach that tackles complex biological questions. Such integration fosters groundbreaking discoveries, revealing interactions within cells at a molecular level. This understanding significantly contributes to various fields including pharmacology and genetics, allowing for more targeted and effective treatments.
Interdisciplinary Nature
The interdisciplinary nature of chemical biology is what makes it immensely valuable. It bridges gaps between biology, chemistry, and other relevant disciplines such as physics and medicine. This collaboration leads to the creation and application of innovative tools and methodologies. For example, chemical biologists employ biophysical methods to examine protein structures, providing insights that guide drug design. As techniques evolve, they encourage the inclusion of computational biology, further enhancing the predictive capabilities regarding biological systems.
Influence on Research Fields
The impact of chemical biology on research fields is profound. It influences drug discovery, as scientists utilize chemical compounds to probe biological pathways. Understanding these pathways aids in the discovery of new therapeutic targets. Additionally, the applications extend to synthetic biology, where researchers manipulate genetic elements using chemical techniques. This has significant implications for creating tailored medical solutions and addressing global challenges like antibiotic resistance.
"Chemical biology is no longer just chemistry applied to biology; it is an essential component of research that shapes the future of medicine and biotechnology."
Overall, the importance of chemical biology in modern science cannot be overstated. It not only supports existing disciplines but also leads to unprecedented advancements. By fostering cross-disciplinary interactions, chemical biology is indeed at the forefront of addressing some of the most pressing scientific challenges of our time.
Core Techniques in Chemical Biology
The field of chemical biology relies heavily on established core techniques to drive research and advance understanding of biological systems. These techniques enable scientists to delve into complex interactions at a molecular level. Their relevance cannot be overstated as they bridge the gap between chemistry and biological science. Key methodologies include small molecule probes, bioconjugation strategies, and high-throughput screening. Each plays a role in discovering new therapeutic targets and developing innovative treatments.
Small Molecule Probes
Small molecule probes serve as a fundamental tool in chemical biology. They are typically low molecular weight compounds that can interact specifically with biological macromolecules, like proteins or nucleic acids. These probes allow researchers to investigate biological pathways and mechanisms in a controlled manner.
One prominent advantage of using small molecule probes is their ability to selectively modulate target proteins. This modulation can provide insights into protein function and reveal how alterations impact cellular processes. Additionally, small molecule probes can be used in live-cell imaging, showcasing their versatility in both in vitro and in vivo studies.
Small molecule probes are critical for uncovering the roles of biomolecules in disease states and elucidating therapeutic pathways.
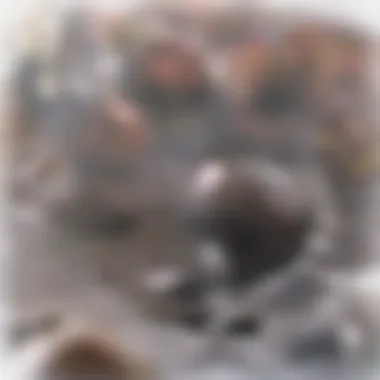
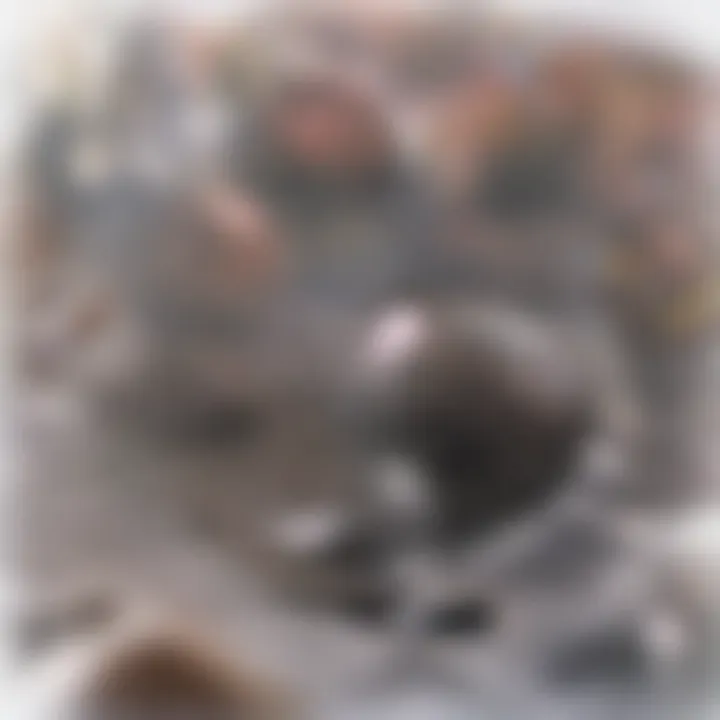
The synthesis of these probes often involves various chemical processes. Techniques such as combinatorial chemistry help produce diverse libraries of compounds, enhancing the likelihood of identifying effective probes.
Bioconjugation Strategies
Bioconjugation refers to the process of covalently linking biological molecules to synthetic compounds. This strategy is pivotal for advancing chemical biology applications. By linking small molecules to proteins or other biomolecules, researchers can facilitate targeted delivery of drugs or imaging agents. This method significantly impacts how therapies are developed.
There are several bioconjugation techniques available, including the click chemistry approach and amine-reactive crosslinking reagents. These methods often prioritize specificity and yield, ensuring that the label or therapeutic agent retains its biological functionality after conjugation.
Furthermore, bioconjugation improves the pharmacokinetic properties of drugs. By modifying the molecular structure, scientists can enhance solubility, stability, and bioavailability of therapeutics, leading to improved treatment efficacy.
High-Throughput Screening
High-throughput screening is a process that allows researchers to quickly conduct millions of chemical, genetic, or pharmacological tests. Each test aims to identify active compounds, antibodies, or genes that modulate a particular biomolecular pathway.
This method saves both time and resources compared to traditional techniques. Automated systems in high-throughput screening can handle large libraries of compounds, allowing for rapid identification of potential candidates for drug development or other biological studies.
Another notable benefit is the ability to perform comprehensive assays under diverse conditions. This flexibility enables identification of target-specific interactions that may otherwise go unnoticed.
In summary, the integration of core techniques such as small molecule probes, bioconjugation strategies, and high-throughput screening is vital. They not only drive advancements in chemical biology but also enhance the overall understanding of complex biological systems. As the field progresses, these methods will continue to evolve, further unlocking uncertainties in biology and paving the way for new therapeutic strategies.
Applications of Chemical Biology
Chemical biology stands as a pillar in contemporary biomedical research, primarily because it interlaces the realms of chemistry and biology. This symbiosis yields a multitude of applications with profound implications for healthcare, environmental science, and beyond. Understanding these applications is vital for students, researchers, educators, and professionals who aspire to delve into this exciting field. The benefits of chemical biology applications are manifold, enabling innovation in drug discovery, pushing boundaries in genetic engineering, and laying the groundwork for synthetic biology.
Drug Discovery
The drug discovery process is one of the most significant applications of chemical biology. This involves the identification and development of new therapeutic agents that can effectively combat diseases. Traditionally, drug discovery was a lengthy and expensive venture, hindered by inefficiencies in identifying promising candidates. However, chemical biology accelerates this process through various innovative techniques, such as target identification and validation, high-throughput screening, and structure-based drug design.
Key Benefits:
- Enhanced precision in identifying biological targets for drug action.
- Streamlined screening methods that reduce time and costs.
- Targeted design of molecules with optimized properties.
Historically, the use of small molecule probes has revolutionized this area by allowing researchers to investigate biological processes in real-time. The manipulation of biomolecules becomes possible, leading to better understanding of diseases and their mechanisms. The integration of these techniques results not only in new drugs but also in personalized medicine approaches that cater to individual patient needs.
Genetic Engineering
Genetic engineering exemplifies another critical application of chemical biology. This practice involves the direct manipulation of an organism’s genes using biotechnology. By employing techniques such as CRISPR/Cas9 and gene editing, scientists are able to modify genetic sequences with high specificity and efficiency.
Considerations in Genetic Engineering:
- Ethical implications surrounding gene manipulation.
- Regulatory frameworks that govern genetic modifications.
- Potential impacts on biodiversity and ecosystems.
With these advances, the potential to combat genetic disorders increases significantly. The capability to address hereditary diseases at their root level presents a transformative opportunity in healthcare. Moreover, genetic engineering is pivotal in agriculture, leading to the creation of genetically modified organisms (GMOs) that can withstand diseases and pests while enhancing crop yields.
Synthetic Biology
Synthetic biology represents the future of biological engineering, where scientists synthesize complex biological systems from simpler biological parts. This application embraces an interdisciplinary approach, combining principles from biology, engineering, and computer science.
Core Aspects of Synthetic Biology:
- Designing and building new biological parts and systems.
- Reprogramming living organisms for enhanced functionality.
- Developing new organisms that can produce materials or biofuels.
The implications of synthetic biology are vast. It has the potential to create sustainable solutions to pressing global challenges, such as energy and food production. Furthermore, synthetic biology can lead to innovations in materials science through the production of bioplastics or biofuels, thus addressing environmental concerns. As research progresses, the ethical and societal implications will need thorough evaluation to guide responsible development in this robust field.
"The applications of chemical biology are not merely academic pursuits; they embody the potential to shape our future, from healthcare to environmental sustainability."
In summary, applications of chemical biology span a diverse array of fields and present substantial benefits and challenges. From drug discovery to introducing groundbreaking techniques in genetic engineering and synthetic biology, the fusion of these disciplines drives innovation and experimentation. As the world grapples with complex biological challenges, the role of chemical biology becomes increasingly critical.
Notable Case Studies
In the field of chemical biology, case studies play a crucial role in illustrating the practical applications of research. These case studies not only highlight specific advancements but also provide insights into how complex biological problems can be addressed through chemical approaches. Understanding these case studies can enhance the interest of students, researchers, and professionals. They reveal the interdisciplinary nature of chemical biology, showcasing its influence on various sectors of health, industry, and environmental science.
Antibiotic Development
Antibiotic development has been one of the most significant achievements in the realm of chemical biology. With the rise of antibiotic-resistant bacteria, innovative approaches are critical for the creation of new antimicrobial agents. For example, the discovery of penicillin by Alexander Fleming in 1928 marks a pivotal moment in medical history. Recent studies have focused on utilizing natural sources, such as soil bacteria and fungi, to isolate novel compounds with antibacterial properties.
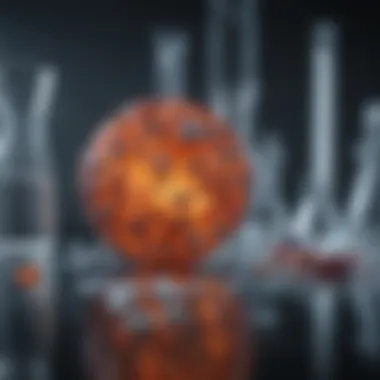
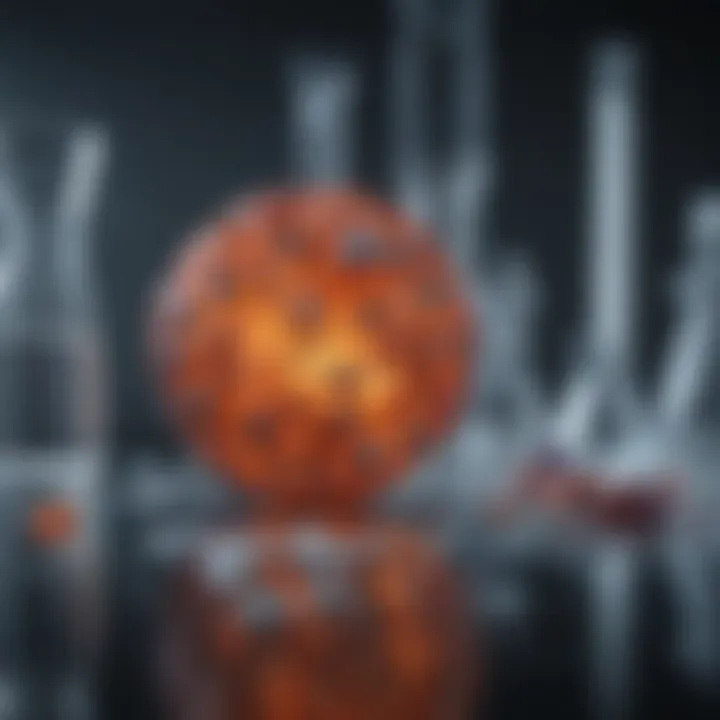
Through random screening of microbial extracts, researchers are identifying molecules that can potentially become future antibiotics. Techniques such as high-throughput screening allow for the rapid evaluation of thousands of compounds. This approach is complemented by computational methods that predict the activity of these compounds against resistant strains.
Cancer Therapeutics
Cancer remains one of the most complex diseases to tackle, and chemical biology has made substantial contributions in this area. Targeted therapies, like imatinib for chronic myeloid leukemia, have transformed treatment protocols. These drugs work by specifically targeting the biochemical pathways that cancer cells exploit for growth and survival.
In addition, chemical biologists are developing small molecules that can inhibit specific proteins involved in tumorigenesis. The use of monoclonal antibodies has also shown promise in modulating immune responses against tumors. Ongoing research aims to refine these therapies to enhance efficacy and reduce side effects, which are key considerations in patient management.
Fluorescent Imaging Agents
Fluorescent imaging agents represent a vital tool in chemical biology for visualizing biological processes in real time. These agents, often equipped with specific chemical modifications, can bind to target molecules or structures in living cells. The distinct advantage of using fluorescent probes is their ability to provide vivid and precise images of cellular activities, allowing researchers to monitor processes such as protein interactions and intracellular trafficking.
For example, molecular probes based on the fluorescence resonance energy transfer (FRET) technique enable detailed studies of molecular dynamics. These imaging agents contribute significantly to our understanding of disease mechanisms, helping to elucidate the biological pathways involved in conditions such as cancer and neurodegenerative disorders.
In summary, case studies in antibiotic development, cancer therapeutics, and fluorescent imaging agents illustrate the multifaceted benefits of chemical biology. They not only reflect the evolving landscape of scientific research but also underline the importance of interdisciplinary efforts in confronting global challenges.
Challenges in Chemical Biology Research
The exploration of chemical biology is not without its challenges. Understanding and manipulating biological systems through chemistry involves navigating complex interactions and mechanisms. The research landscape is filled with hurdles that require astute problem-solving skills and innovative thinking from researchers. Addressing these challenges is vital for advancing the field and optimizing outcomes in various applications like drug development and genetic engineering.
Complexity of Biological Systems
Biological systems are inherently complex. They function through intricate networks of biochemical reactions, often involving multiple pathways and feedback loops. The behavior of these systems is often unpredictable, making it difficult to apply chemical principles effectively. One must consider numerous variables, such as protein folding, enzyme activity, and cellular environment, which can all impact results.
Researchers face the difficulty of reproducibility, where results from experiments may vary due to the inherent biological variability. This complexity necessitates sophisticated modeling techniques and thorough experimental designs to draw reliable conclusions about how specific chemical agents interact with biological systems.
- Interconnectedness: Biological systems do not operate in isolation. Changes to one component can affect many others.
- Variability: Genetic differences among organisms lead to variability, which must be accounted for in experimental setups.
"Understanding the complexity of biological systems requires a multidisciplinary approach, integrating insights from chemistry, biology, and even computational modeling."
Regulatory Hurdles
Research in chemical biology is often subject to strict regulatory requirements. These regulations are crucial for ensuring safety and efficacy, especially when it comes to drug development and therapeutic applications. However, they can also present significant challenges. The approval process can be lengthy, involving rigorous testing and validation phases that can delay potential breakthroughs.
Researchers must familiarize themselves with regulatory frameworks that vary by region. Navigating these requirements involves:
- Preclinical Testing: Extensive studies must be conducted before clinical trials can commence, often requiring significant time and resources.
- Documentation and Compliance: Researchers are tasked with maintaining comprehensive records of their methodologies, results, and any adverse effects, which can be labor-intensive.
The burden of regulatory compliance can limit the speed at which new discoveries transition from the lab to practical applications. Often, researchers must balance adherence to regulations with the need for creative approaches in their studies.
In summary, the challenges in chemical biology research highlight the need for ongoing innovation and collaboration. By addressing these complexities and regulatory demands, researchers can pave the way for significant advancements in the field, ultimately enabling more effective interventions in health and disease.
Future Directions
The realm of chemical biology is constantly evolving, driven by advancements in both genetic understanding and technological prowess. This section will explore future directions for research and application within chemical biology, focusing on key areas such as emerging technologies and personalized medicine. Understanding these elements is crucial for scientists and researchers aiming to harness chemical biology for innovative solutions in health and beyond.
Emerging Technologies
Emerging technologies are reshaping the tools and methodologies available in chemical biology. For example, CRISPR-Cas9 represents a significant breakthrough in gene editing, allowing precise modifications in DNA. This technique enhances our ability to study gene functions and develop tailored therapeutics.
Other technologies like single-cell sequencing and mass spectrometry have also made substantial contributions. These technologies enable detailed analysis of complex biological samples at unprecedented resolutions. They help researchers to uncover novel interactions within cellular environments and to explore cellular heterogeneity.
In addition, artificial intelligence is being increasingly applied to streamline drug discovery processes. AI algorithms can analyze vast datasets, predict molecular interactions, and optimize compounds swiftly. This not only accelerates the pace of discovery but also enhances the precision of developing new therapeutic agents.
"The integration of advanced technologies will likely yield innovative strategies that are vital for addressing complex biological challenges."
Personalized Medicine
Personalized medicine signifies a paradigm shift in therapeutic approaches, tailoring treatments to individual patients based on their genetic makeup and unique biological profiles. Chemical biology plays a pivotal role in this transition, as understanding molecular mechanisms allows clinicians to design more effective treatments.
The application of biomarker discovery is a crucial aspect of personalized medicine. Identifying specific biomarkers can guide treatment decisions, ensuring that therapies are more targeted. For instance, some cancer treatments are now prescribed based on genetic mutations found within tumors rather than broad categories of cancer types.
Additionally, pharmacogenomics, the study of how genes affect a person's response to drugs, is integral to developing personalized strategies. By analyzing variations in patients' genomes, researchers can predict which medications will be the most effective, minimizing adverse reactions.
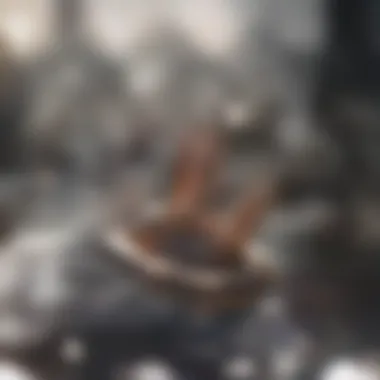
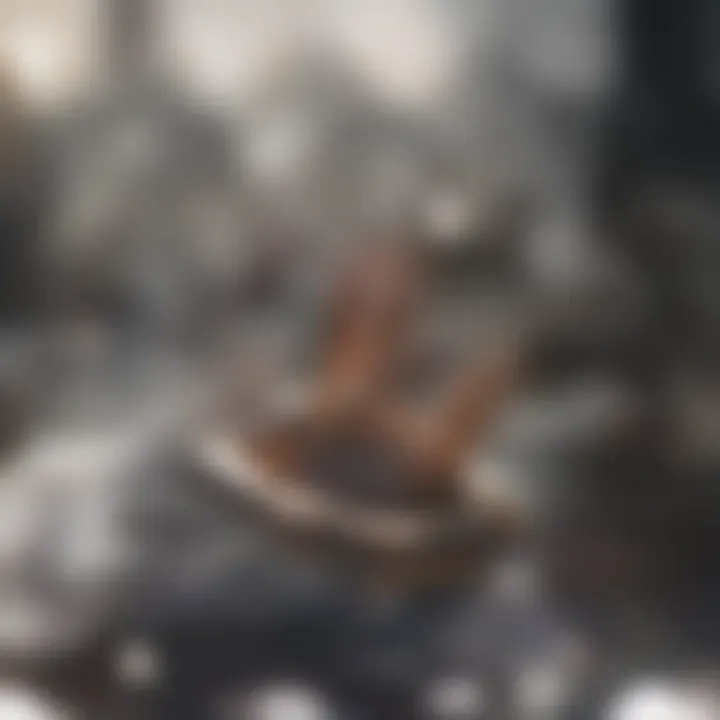
In summary, the future of chemical biology holds significant promise through emerging technologies and the advent of personalized medicine. These advancements are essential for fostering more effective therapeutic strategies, demonstrating the ongoing relevance and importance of this interdisciplinary field in addressing future challenges in health and disease.
Educational Pathways in Chemical Biology
The field of chemical biology is not only complex but also ever-evolving, necessitating a robust educational framework to equip future scientists. Educational pathways in chemical biology are essential in shaping the next generation of researchers and professionals. These pathways provide the foundational knowledge and practical skills required to understand and manipulate biological systems utilizing chemical principles. This section examines the various educational avenues available and their significance in fostering a deeper understanding of the discipline.
Undergraduate Programs
Undergraduate programs in chemical biology typically encompass a broad spectrum of courses that integrate chemistry and biology. Students may pursue degrees specifically in chemical biology or adjacent disciplines like biochemistry or molecular biology. These programs often emphasize core concepts such as organic and inorganic chemistry, cell biology, and biostatistics. Importantly, early exposure to lab-based work is crucial.
Key components of undergraduate programs include:
- Interdisciplinary curriculum that covers essential principles from both fields.
- Hands-on laboratory experiences that enhance the understanding of experimental techniques.
- Opportunities for internships or research projects that connect students with real-world applications.
Undergraduate studies serve as the bedrock for students, effectively preparing them for more advanced work in the field.
Graduate Studies
Graduate studies in chemical biology allow for specialization and in-depth exploration of specific areas of interest. Graduate programs often offer Master's and Ph.D. degrees, focusing on advanced topics, such as drug design, molecular signaling, or synthetic biology. These programs insist on a strong research component, as candidates must contribute original findings to the scientific community.
Benefits of pursuing graduate studies include:
- Development of specialized skills critical for advanced research and professional work.
- Emphasis on independent thinking, fostering innovation among scholars.
- Networking opportunities with other researchers and access to collaborative projects.
Research Institutions and Organizations
The field of chemical biology relies significantly on research institutions and organizations. These entities play a crucial role in advancing the science, fostering collaboration, and propelling innovative discoveries. By bringing together experts from diverse scientific backgrounds, these organizations help to bridge the gap between chemistry and biology, facilitating a multidisciplinary approach. The benefits they provide include access to resources, funding opportunities, and platforms for collaboration.
Research institutions often house cutting-edge laboratories equipped with advanced technology. This facilitates experimentation and allows researchers to design and execute complex studies that may not be possible in less-equipped settings. Additionally, many institutions maintain extensive libraries and databases that compile vast amounts of scientific data, enhancing research capabilities.
Considerations about research organizations include their distinct focus areas, which can vary widely. Some institutions prioritize applied research, while others may focus on basic science. This variability can impact the type of training and mentorship available to students and early-career scientists. Choosing the right institution or organization can profoundly influence one’s career trajectory in the field of chemical biology.
In summary, research institutions and organizations are integral to the progress of chemical biology. They not only provide the infrastructure necessary for research but also create environments where innovation can thrive.
Leading Research Centers
Leading research centers in chemical biology are often at the forefront of discovery and innovation. Institutions like The Scripps Research Institute and the Broad Institute of MIT and Harvard are notable examples. These centers not only contribute to significant research outputs but also offer robust training programs.
- The Scripps Research Institute: Known for its pioneering work in drug discovery and biochemical research.
- Broad Institute: Focuses on genomic medicine and has a significant role in the integration of chemical biology with genomic technologies.
These institutions attract top talents and facilitate collaborations across global networks, thus enhancing their research output and impact.
Collaborative Networks
Collaborative networks are essential in the realm of chemical biology. Such networks allow for the sharing of resources, data, and ideas across multiple institutions and organizations.
These networks often involve:
- Interdisciplinary collaboration: Professionals from various fields, including chemists, biologists, and bioinformaticians, work together to solve complex problems.
- Global partnerships: Working with institutions around the world can provide diverse perspectives and expertise, leading to innovative solutions.
Effective collaboration can lead to breakthroughs that might not be achievable by individual labs.
The establishment of collaborative networks has resulted in several high-impact projects, enhancing the overall capacity of the field to address challenging scientific questions. Through these partnerships, researchers can push the boundaries of chemical biology, making significant contributions to medicine and environmental science.
Culmination
In understanding the multifaceted domain of chemical biology, it becomes evident just how pivotal it is for the advancement of scientific inquiry. The cross-disciplinary approach inherent in this field encourages the application of chemistry in deciphering biological mechanisms. As presented throughout this article, the significance of chemical biology can be summarized through various essential elements and benefits.
Summing Up Contributions
The contributions of chemical biology are profound and varied. These include but are not limited to:
- Innovation in Drug Development: Chemical biology has propelled advancements in therapeutics, leading to the development of more effective drugs, particularly in treating diseases like cancer.
- Understanding Biological Processes: By using small molecule probes and other techniques, scientists better comprehend complex biological pathways and interactions.
- Facilitating Genetic Research: Through applications such as genetic engineering and synthetic biology, chemical biology contributes immensely to our understanding of genetics and its implications in health.
This article also offers insights into notable case studies, which reinforce the varied impact of chemical biology. From antibiotics to imaging agents, each case illustrates how critical this field is in bridging gaps between chemistry and biology.
Final Thoughts
As we look ahead, the future of chemical biology appears promising. Emerging technologies and the trend towards personalized medicine signal a shift in how we approach treatment and disease control. As such, investing time and resources into this field is not merely beneficial but necessary for continued progress.
The true power of chemical biology lies in its ability to merge the fundamental principles of chemistry with the dynamic complexities of life.