Exploring Dry Biomass: Characteristics and Applications
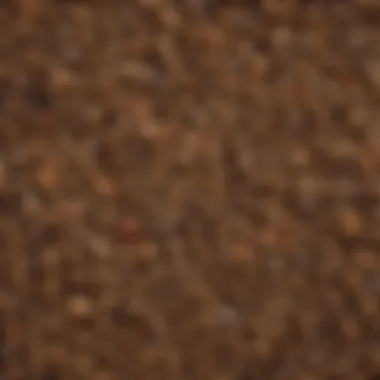
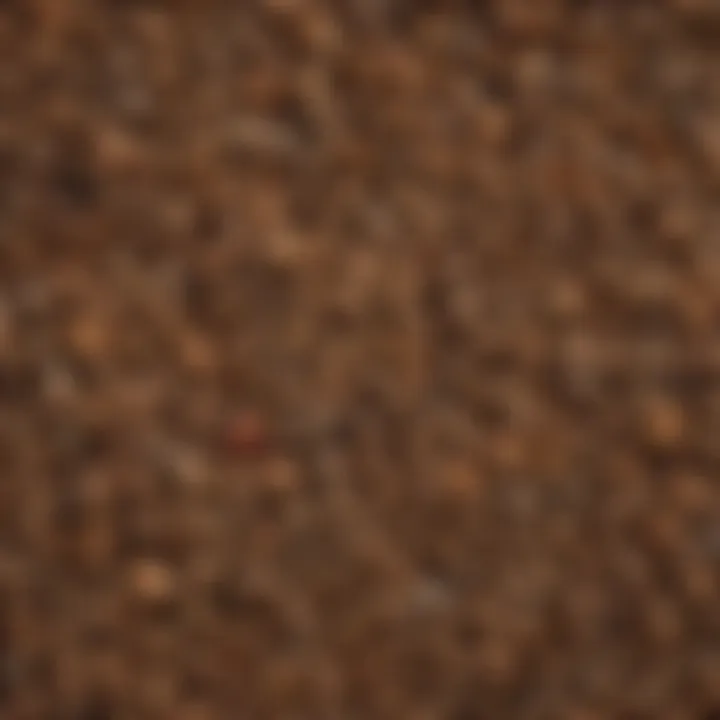
Intro
Biomass has emerged as a critical resource in today's quest for sustainable energy solutions. Among its various forms, dry biomass stands out for its unique characteristics and applications. Understanding dry biomass is crucial for both researchers and practitioners. This resource offers immense potential for energy production, agriculture, and waste management. Fueling discussions about sustainable practices, the exploration of dry biomass necessitates an examination of its favorable traits and inherent challenges.
Research Background
Overview of the Scientific Problem Addressed
The increasing demand for renewable energy sources has prompted extensive research into biomass. Dry biomass, characterized by a low moisture content, is particularly noteworthy. It provides an efficient energy source compared to wet biomass. Its role in reducing waste and enhancing sustainability needs scholarly attention. The core scientific problem focuses on optimizing the utilization of dry biomass within energy systems and agricultural frameworks.
Historical Context and Previous Studies
Historically, biomass has been used as a fuel for many centuries. However, a notable shift occurred in the late 20th century. Increased awareness of climate change led to a rise in research concerning bioenergy. The significant studies have predominantly focused on the energy release from different biomass types. Literature indicates that dry biomass has a higher calorific value compared to its wet counterparts. Researchers have also analyzed collection and storage methods. Despite these contributions, a thorough examination of dry biomass's specific applications remains underexplored.
Findings and Discussion
Key Results of the Research
Recent studies highlight several characteristics that make dry biomass advantageous. Firstly, its energy density is higher, leading to more efficient combustion processes. This efficiency translates into lower transportation costs and reduced emissions during burning. Secondly, the versatility of dry biomass enables its use across various sectors, from cooking fuel to electricity generation.
Interpretation of the Findings
The findings suggest that the integration of dry biomass within energy systems can contribute to reducing dependence on fossil fuels. Moreover, it supports sustainability goals by utilizing organic wastes, such as agricultural residues. As attention on climate change increases, the viability of dry biomass as a renewable resource places it at the forefront of sustainable energy solutions.
"The potential of dry biomass as a resource goes beyond energy production; it embodies a dual role in waste management and sustainability efforts."
By understanding the role dry biomass plays in energy and sustainability, future research efforts can be directed to maximize its utility. This exploration serves to inform practitioners, researchers, and policymakers alike.
Prolusion to Dry Biomass
The study of dry biomass is essential in understanding its pivotal role in various ecological and industrial frameworks. As a renewable resource, it promises substantial benefits in sustainability, offering alternative pathways to traditional fossil fuels. The focus on dry biomass not only highlights its potential for energy production but also underscores its value in waste management and agricultural practices. An informed discourse about dry biomass can lead to innovative solutions addressing pressing environmental issues such as climate change and resource depletion.
Definition of Dry Biomass
Dry biomass generally refers to the organic material derived from plants and animals, which holds a low moisture content. It encompasses various forms, including agricultural residues, forestry byproducts, and certain types of waste. The moisture content of dry biomass is usually below 20%, making it suitable for combustion, gasification, or conversion to biofuels. Understanding the precise definition is critical as it influences the methods employed for its collection and processing.
Importance of Biomass in the Ecological Context
Biomass plays a vital role in ecological balance. It acts as a carbon sink, helping to sequester atmospheric carbon dioxide. Moreover, it fosters soil health and promotes biodiversity. The utilization of dry biomass in energy generation can significantly diminish greenhouse gas emissions if managed properly. As a result, it is crucial to analyze how integrating dry biomass into energy systems contributes to sustainable practices while minimizing the ecological footprint.
"The utilization of dry biomass as a renewable energy source has profound implications for both environmental sustainability and economic viability."
Researchers and practitioners must also consider the implications of using dry biomass on ecosystems. The methods of sourcing biomass can affect local biodiversity and soil structure. Thus, a thorough exploration of its ecological ramifications is essential to formulate effective policies that promote sustainable practices in biomass consumption.
Characteristics of Dry Biomass
Understanding the characteristics of dry biomass is essential for researchers and practitioners in the field. The physical and chemical attributes of dry biomass influence its usability across various applications. This section examines crucial elements such as moisture content, chemical composition, and physical properties, which determine how dry biomass can be processed and employed effectively.
Moisture Content Analysis
Moisture content is a primary characteristic of dry biomass that significantly impacts its energy efficiency and handling. Generally, ideal dry biomass should possess a low moisture level, typically below 15%. Excess moisture can hinder combustion efficiency and reduce the overall energy output when used for energy production.
In addition, the moisture content affects the transportation and storage of biomass. Heavier loads due to water can increase transportation costs. Therefore, it is important to analyze the moisture regularly. Techniques like oven drying and infrared drying are commonly used to evaluate moisture levels accurately.
Chemical Composition and Energy Content
The chemical composition of dry biomass defines its energy content and determines the types of chemicals and materials released during combustion or conversion processes. Common components include cellulose, hemicellulose, lignin, and various extractives. These constituents are responsible for the biomass's thermochemical behavior and energy density.
A higher lignin content is often associated with improved structural integrity but may also lead to a lower energy yield. The energy content can be assessed through standardized tests, such as calorimetry, which denotes the heat produced during combustion. Understanding the chemical makeup aids in predicting how the biomass will perform in different applications, especially for energy production and industrial uses.
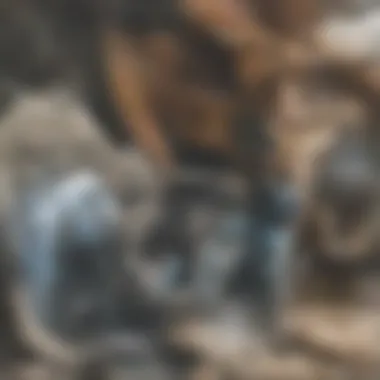
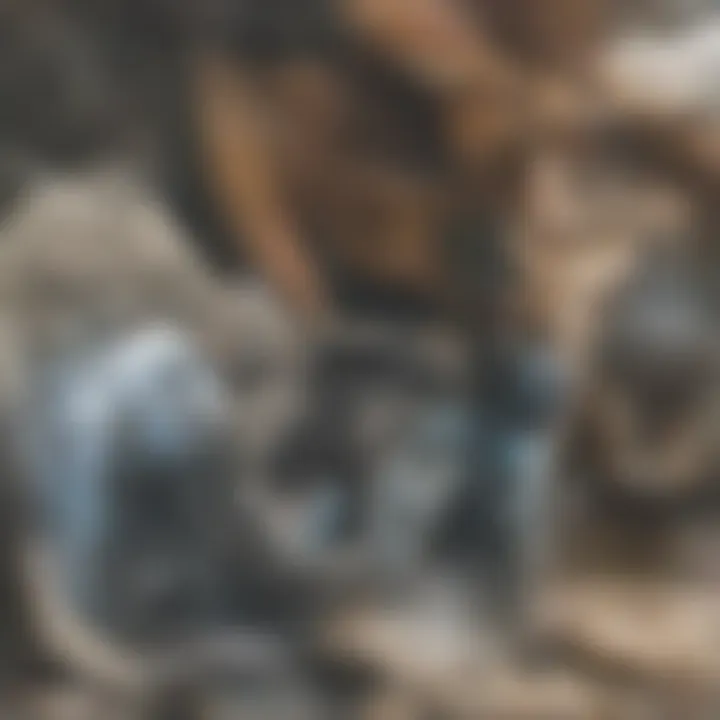
Physical Properties and Processing Challenges
The physical properties of dry biomass, like particle size, density, and structure, play a crucial role in determining processing strategies. These characteristics influence how dry biomass can be milled, pelletized, or otherwise prepared for specific applications. For instance, uniform particle size is desirable for consistent combustion and efficient storage.
However, the variation in physical properties can pose challenges. For example, when biomass is collected from different sources, it may exhibit inconsistencies in size and moisture content, complicating processing efforts. These challenges necessitate innovative pre-processing techniques such as size reduction and specific drying methods to enhance uniformity and optimize the characteristics of biomass for intended applications.
"The effectiveness of dry biomass utilization hinges critically on its intrinsic characteristics, which must be analyzed and managed judiciously."
Collection and Processing of Dry Biomass
The collection and processing of dry biomass are critical aspects of utilizing this resource effectively. These processes not only facilitate efficient use of biomass in various applications but also influence its sustainability and overall environmental impact. Efficient collection methods ensure the availability of dry biomass while proper processing techniques enhance its qualities for different uses, thus maximizing its benefits.
Sources of Dry Biomass
The sources of dry biomass are diverse, each contributing uniquely to its overall value. Understanding these sources is vital for effective collection and processing.
Crop Residues
Crop residues, such as straw, corn stover, and wheat chaff, represent a significant source of dry biomass. They are produced during the harvesting of crops and often left in fields, leading to underutilization. These residues are advantageous as they are abundant and inexpensive. The high carbon content of crop residues makes them suitable for applications in energy production and soil enhancement. However, their composition can vary widely depending on the type of crop and environmental conditions, which may pose challenges during processing.
Forestry Residues
Forestry residues include wood chips, sawdust, and bark, generated from timber harvesting and processing activities. This source is particularly rich in cellulose and lignin, making it valuable for energy generation and bio-material production. The key characteristic of forestry residues is their high energy density, which enhances their efficiency as a biomass source. However, the collection of these residues can be logistically complex due to their location in forested areas. Furthermore, care must be taken to prevent overharvesting, which can lead to significant ecological impacts, such as habitat destruction.
Animal Manure
Animal manure is another significant source of dry biomass, especially in agricultural settings. Beyond its potential as a fertilizer, manure can be processed into biogas through anaerobic digestion. This process not only recycles nutrients but also produces renewable energy. The key characteristic of animal manure is its high nutrient content, which can greatly improve soil health when used appropriately. Nonetheless, there are challenges, such as the presence of pathogens and the need for proper management to avoid environmental pollution.
Methods of Collection
Methods for collecting dry biomass must be thoughtfully designed to optimize yield while minimizing environmental disturbances. Various techniques can include mechanical harvesting, manual collection, and residue management practices. Each method carries its unique advantages and limitations, often influenced by the source of biomass and local conditions. Efficient logistics in collection could greatly enhance the feasibility of dry biomass utilization in energy production and agriculture.
Pre-processing Techniques
Pre-processing techniques are essential to prepare dry biomass for further application. These processes aid in enhancing biomass quality, increasing processing efficiency, and improving usability in various applications.
Size Reduction
Size reduction is a pivotal pre-processing technique that involves cutting, grinding, or shredding biomass into smaller pieces. This step is crucial because smaller particles improve the efficiency of subsequent processes, including combustion and gasification. The key characteristic of size reduction is its ability to facilitate more uniform particle size, enhancing energy yield and efficiency. However, this technique requires energy input and can introduce additional costs.
Drying Methods
Drying methods serve to reduce moisture content in biomass, which is vital for storage and combustion efficiency. There are several drying methods available, including solar drying, rotary drying, and flash drying. Each method has its specific advantages and drawbacks. For instance, solar drying is cost-effective and environmentally friendly but highly dependent on weather conditions. On the other hand, rotary drying is efficient but can be energy-intensive.
Applications of Dry Biomass
The applications of dry biomass are significant in various sectors including energy, agriculture, and industry. Understanding these applications is crucial for utilizing the full potential of dry biomass resources. Through proper implementation, dry biomass can enhance sustainability efforts, improve waste management, and contribute to energy needs. Each application type provides unique benefits and considerations that merit attention, especially in the context of advancing ecological practices.
Energy Production from Dry Biomass
Direct Combustion
Direct combustion is one of the most straightforward methods for employing dry biomass to generate energy. In this process, biomass is burned to produce heat, which can then generate steam for electricity production or heat for residential and industrial use. The key characteristic of direct combustion is its simplicity; it requires minimal processing compared to other methods.
The advantage of direct combustion lies in its accessibility. Many types of dry biomass, such as wood waste and agricultural byproducts, can be used directly with little alteration. However, this method can generate particulate matter which raises concerns about air quality.
Gasification
Gasification transforms dry biomass into a synthetic gas called syngas. This process involves heating biomass in a low-oxygen environment. The syngas can then be converted into electricity or used as a basic chemical feedstock. The significant aspect of gasification is its higher efficiency compared to direct combustion. It allows for the use of biomass to produce a cleaner form of energy.
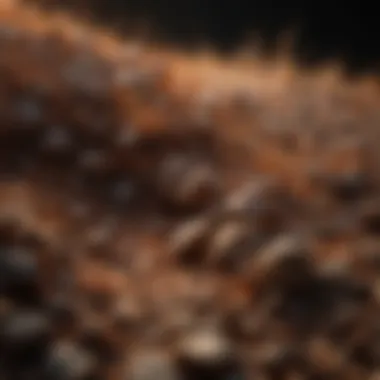
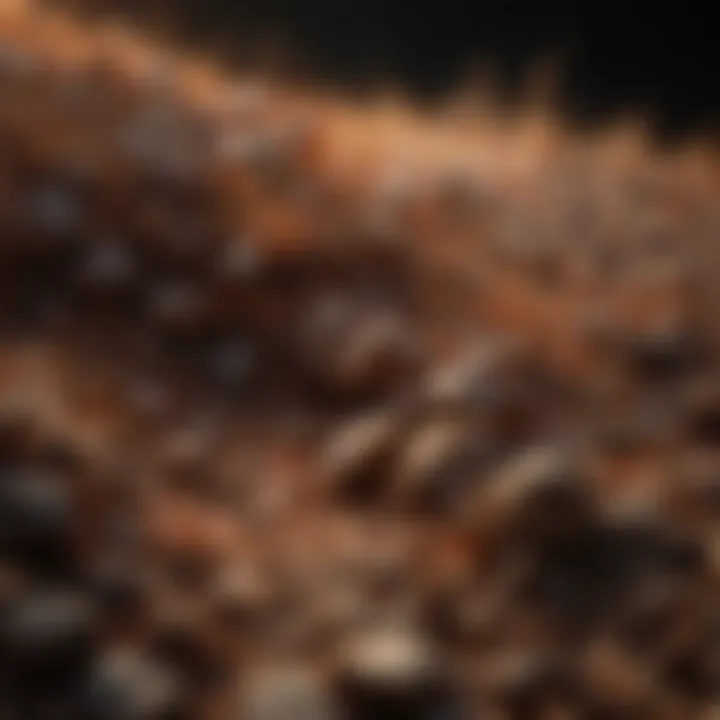
Gasification provides flexibility. Different types of biomass can be fed into the system, giving it a unique characteristic. Despite this, gasification requires more sophisticated technology and investment, which can be a barrier in some cases.
Biomass Pellets
Biomass pellets are a refined form of dry biomass, made by compressing organic materials like wood, agricultural residues, or other biomass. The key characteristic of biomass pellets is their density, which makes them easier to store and transport compared to loose biomass materials.
Biomass pellets are popular as they can be used in various heating systems and power plants designed for pellet use. Their uniform size and moisture content provide a consistent burning experience, but producing pellets requires additional processing. This extra step may increase costs, impacting overall economic feasibility.
Agricultural Uses
Soil Amendment
Using dry biomass as a soil amendment enriches soil quality, promoting plant health. It typically involves integrating dry leaves, crop residues, or composted animal manures back into the soil. A major benefit of soil amendment is its ability to improve soil structure and nutrient retention.
This practice also contributes to sustainable agriculture by recycling nutrients that would otherwise be lost. The unique feature of soil amendment using dry biomass is its role in promoting microbial activity, which enhances soil fertility. However, there may be variations in composition that must be managed to avoid nutrient imbalances.
Mulching Material
Dry biomass can serve as an effective mulch material, covering soil surfaces to suppress weeds and retain moisture. Common materials include straw, wood chips, and grass clippings. The primary benefit of using dry biomass for mulching is its ability to control soil temperature and moisture levels, promoting healthier plant growth.
Another advantage is that it utilizes materials that may be considered waste. The unique feature of mulching material lies in its capability to break down and add organic matter to the soil over time. However, the decomposition process can take longer and may require careful management to avoid nutrient depletion.
Industrial Applications
The industrial sector utilizes dry biomass in multiple ways, primarily to reduce carbon footprints and capitalize on renewable resources. Biomass is increasingly seen as a substitute for fossil fuels in various processes. This can include its use in manufacturing, heating, and chemical production. The key aspect of industrial applications of dry biomass is diversification; multiple sectors can integrate biomass sustainably.
One notable characteristic is its potential to reduce operational costs over time when used as a substitute for traditional fuels. However, variability in supply and the need for specific processing can create complexities in the supply chain.
Overall, the various applications of dry biomass highlight its flexibility and potential in improving sustainability across sectors. By understanding these applications, stakeholders can harness dry biomass effectively.
Sustainability and Environmental Impact
Sustainability and environmental impact are crucial elements in the exploration of dry biomass. The utilization of dry biomass not only addresses energy needs but also aligns with ecological goals. The intricate relationship between biomass use and environmental health necessitates an understanding of various impacts and benefits.
The drive for sustainable energy solutions has pushed the focus onto renewable resources like dry biomass. Unlike fossil fuels, dry biomass can potentially reduce greenhouse gas emissions. As the world grapples with climate change, finding cleaner energy options becomes paramount. In this context, assessing the sustainability of dry biomass involves examining its life cycle from collection to application.
Life Cycle Assessment of Dry Biomass
Life cycle assessment (LCA) of dry biomass evaluates its environmental impacts over its entire life cycle. This involves stages such as raw material extraction, processing, usage, and finally, disposal or recycling. Each phase presents opportunities and challenges in terms of sustainability.
In the collection phase, the source of biomass plays a significant role. For instance, using agricultural residues from crops can minimize waste while providing a renewable energy source. The processing involves conversion techniques which also greatly affect energy efficiency and emissions.
The utilization of dry biomass for energy production, as opposed to burning fossil fuels, demonstrates its potential benefits. However, emissions released during combustion need assessment. The LCA methods provide quantitative data to determine both positive and negative impacts, ensuring the informed selection of biomass resources for sustainable practices.
Carbon Footprint Considerations
The carbon footprint of dry biomass depends on various factors including the type of biomass, collection methods, and conversion techniques used. A significant aspect of utilizing dry biomass is its potential for carbon neutrality. Theoretically, the carbon dioxide released during combustion can be offset by the carbon dioxide absorbed by the plants during their growth.
However, practical applications reveal complexities. For instance, if biomass is sourced from deforestation or poor management practices, the overall carbon emissions can increase. The transportation of biomass also contributes to its overall carbon footprint.
Here are some key factors to consider:
- Sourcing practices: Sustainable sourcing minimizes the carbon footprint.
- Transportation emissions: Local sourcing reduces emissions.
- Conversion efficiency: More efficient conversion leads to less waste and emissions.
Proper assessment and management of these elements are essential to ensure that the use of dry biomass genuinely contributes towards lowering overall emissions.
"Sustainability in biomass production is not just about renewable energy but ensuring a balance between energy production and environmental health."
Maintaining an accurate understanding of the carbon footprint is integral for advancing the use of dry biomass as a reliable and sustainable energy source.
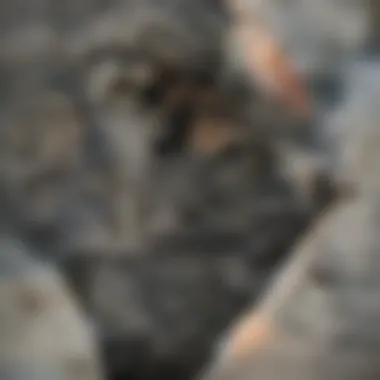
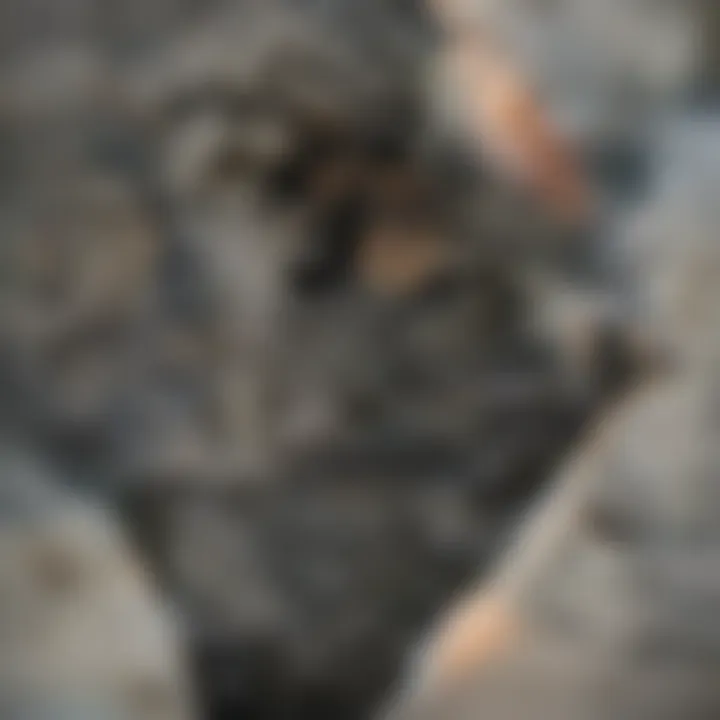
Advantages and Limitations of Dry Biomass
Dry biomass plays a significant role in various sectors, including energy, agriculture, and industry. Understanding the advantages and limitations of dry biomass is crucial for evaluating its potential and addressing issues arising from its use.
Benefits of Utilizing Dry Biomass
Sustainability Potential
Sustainability potential is a critical aspect of dry biomass. The utilization of dry biomass can lead to a reduction in fossil fuel consumption. As it is renewable and biodegradable, dry biomass contributes to a circular economy, minimizing dependence on non-renewable resources. This makes it a compelling choice for sustainable energy solutions.
One key characteristic of sustainability potential is its ability to be sourced from agricultural waste, forestry residue, and other organic materials. These sources not only provide energy but also help in waste management. The unique feature of utilizing waste reduces environmental hazards associated with landfill accumulation. Thus, it limits greenhouse gas emissions, making it a beneficial option for addressing climate change while ensuring energy security.
Waste Minimization
Waste minimization is another important benefit of dry biomass. By converting waste into energy or useful products, the overall volume of waste is reduced. This process also prevents waste from incineration or dumping in landfills, which have detrimental environmental impacts. The emphasis on waste minimization aligns with broader sustainability goals and environmental regulations.
A key characteristic of waste minimization is its dual role in energy recovery and environmental protection. This aspect positions dry biomass as a vital component in achieving zero waste initiatives. The unique feature of integrating waste into the energy supply chain presents advantages in economic savings and environmental gains, creating a win-win scenario.
Challenges in Dry Biomass Utilization
While there are clear benefits, dry biomass also faces challenges in its utilization.
Variability in Composition
Variability in composition is a significant challenge associated with dry biomass. Biomass materials can differ widely in their physical and chemical properties, which can influence their performance as a fuel source. This inconsistency makes it difficult to predict energy output and can complicate the design of efficient processing systems.
A notable characteristic of this variability is that it can impact combustion efficiency and emissions. The unique feature of differing compositions may lead to fluctuations in energy content and combustion behavior, which can result in operational issues for biomass energy facilities. As such, addressing variability is critical for optimizing biomass use in energy generation.
Logistical Constraints
Logistical constraints present practical challenges in the collection, transportation, and processing of dry biomass. The decentralized nature of biomass resources often leads to increased costs and extended supply chains. Issues such as seasonal availability and transportation logistics also add complexity to utilizing dry biomass effectively.
A key characteristic of logistical constraints is the need for efficient logistical planning and coordination. Without a well-structured approach, economic viability can be compromised. The unique feature of lengthy supply chains may lead to time delays and increased carbon footprints, counteracting some of the sustainability benefits that dry biomass offers. Therefore, tackling these logistical challenges remains essential for maximizing the potential of dry biomass.
Future Trends and Research Directions
Understanding the future trends and research directions related to dry biomass is essential for advancing this field. The shift towards renewable energy sources and sustainable practices positions dry biomass as a key player in energy production and environmental management. Innovative methods can enhance efficiency and create new applications. Therefore, continuous research into these areas is vital for maximizing the benefits that dry biomass can offer in the fight against climate change and resource depletion.
Innovative Processing Technologies
Innovative processing technologies play a significant role in enhancing the utilization of dry biomass. Current methods focus on improving the efficiency of energy extraction and minimizing waste. For instance, technologies such as advanced pyrolysis and enzymatic hydrolysis can increase the yield of biofuels from dry biomass. These methods allow for the conversion of biomass into a variety of energy products, thereby broadening the scope of its applications.
Research into chemical and biological pretreatments also shows promise. By modifying the structure of biomass, these processes can improve digestibility and energy output. For example, using ionic liquids can solubilize lignocellulosic materials more effectively. Such processing technologies not only augment energy production but also make the overall biomass conversion process more economically viable.
Policy and Regulatory Frameworks
The development of effective policy and regulatory frameworks is vital for the successful adoption of dry biomass technologies. Policymakers must recognize the environmental benefits of biomass and provide support for innovation. Incentives such as tax breaks or subsidies can encourage research and commercial investment in the biomass sector.
Additionally, clear regulations are necessary to guide the sourcing and utilization of dry biomass in a sustainable manner. This includes establishing standards for biomass quality and environmental impacts. Such frameworks not only facilitate market growth but also ensure that biomass is sourced ethically and responsibly.
Effective policies can create an environment where innovation thrives, ultimately leading to greater efficiency in biomass utilization.
By fostering collaboration between governments, academia, and industry participants, policy frameworks can stimulate research funding and knowledge sharing. This integrated approach is crucial for exploring new uses and technologies that can significantly enhance the role of dry biomass in our energy systems.
Culmination
The conclusion serves as a crucial summation in the exploration of dry biomass, encapsulating its multifaceted importance. Reflecting on the data and analyses presented throughout this article, we underscore how dry biomass stands at the intersection of environmental sustainability, energy efficiency, and agricultural productivity. By examining the unique characteristics, collection methods, and applications of dry biomass, it becomes clear that this resource offers substantial benefits in mitigating waste and sourcing renewable energy.
Recapitulation of Key Points
In summary, key points discussed include:
- Definition and Importance: Dry biomass is derived from various organic materials, contributing significantly to ecological balance.
- Characteristics: We explored the moisture content, chemical composition, and physical properties of dry biomass, revealing the challenges in processing.
- Collection and Processing: Effective methods of collection reflect the diverse sources ranging from crop residues, forestry waste, and manure.
- Applications: Dry biomass finds utilization in energy production, agriculture, and various industrial applications, showcasing its versatility.
- Sustainability: The life cycle assessments and carbon footprint considerations elevated the conversation about biomass towards a sustainable future.
- Advantages and Limitations: While dry biomass presents several benefits like sustainability potential, challenges related to its variability and logistics remain.
- Future Trends: Innovations in processing technologies and fostering regulatory frameworks will shape the future viability of dry biomass.
Final Thoughts on Dry Biomass
The discourse surrounding dry biomass ultimately emphasizes its role as a pivotal resource in the transition towards sustainable energy solutions. As societal demands for clean energy escalate, dry biomass should be regarded not merely as waste but as an asset with untapped potential. Future research must focus on overcoming existing challenges. This includes enhancing processing techniques and developing effective policies that support sustainable utilization. The intersection of agriculture, energy, and environmental preservation hinges on our ability to leverage dry biomass efficiently.