Exploring Hippocampal Neuron Culture Techniques
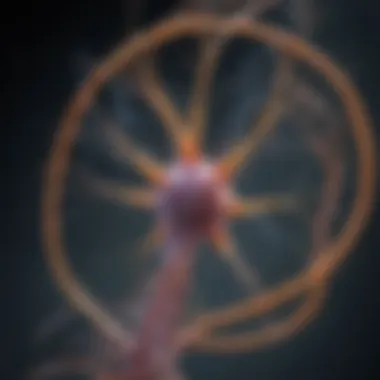
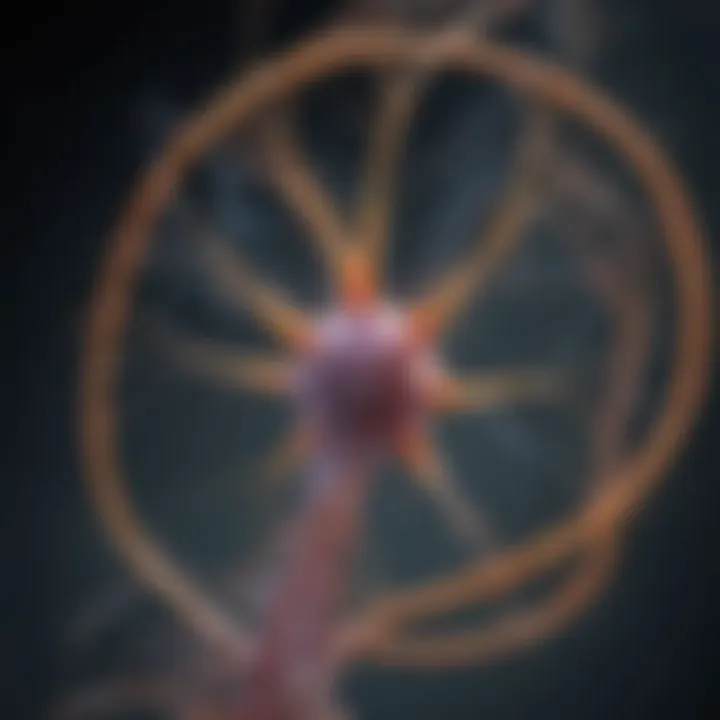
Intro
Hippocampal neuron culture has emerged as a critical aspect of neurobiology research, bringing a trove of insights into the complexities of neuronal behavior and development. With rapid advancements in methodologies, this area of study holds meaningful implications for understanding both the healthy and diseased brain. Here, we pave the way for navigating through the key aspects of isolating and nurturing neurons from the hippocampus, which is an area of the brain intimately involved in memory and spatial navigation.
Research Background
Overview of the Scientific Problem Addressed
The study of hippocampal neurons is pivotal for comprehending underlying mechanisms of neurodegenerative disorders, synaptic plasticity, and overall neural communication. Historically, the challenge lay in successfully culturing these delicate cells while maintaining their physiological properties. Issues such as neuronal death during culture and an inability to replicate in vivo conditions have impeded progress. Thus, addressing these hurdles remains a focal point in neurobiology.
Historical Context and Previous Studies
Looking back, pivotal studies catalyzed the advancement of hippocampal neuron culture techniques. Earlier work by scientist Santiago Ramón y Cajal laid the groundwork, presenting the concept of neurons as individual entities rather than simply a network. Then, in the late 20th century, researchers like Banker and Moses adapted methods for culturing neurons from dissociated brain tissue, honing in specifically on the hippocampus. This evolution has set the stage for current practices, where we can efficiently cultivate neurons while retaining their functionality.
Findings and Discussion
Key Results of the Research
Recent advancements spotlight the optimization techniques that have transformed hippocampal neuron culture. Significant results stem from the application of special media, specific coatings for culture dishes, and the importance of oxygen levels. The introduction of serum-free conditions has shown promise in increasing neuronal survival rates. Furthermore, chronic exposure to damaging substances like glutamate has enhanced the understanding of pathological mechanisms.
Interpretation of the Findings
The implications of these findings stretch beyond mere neuronal survival; they shed light on critical processes such as synaptic development and plasticity. Understanding how neurons react to their environment offers clues into therapeutic approaches for conditions like Alzheimer's disease. Moreover, this robust platform for studying neurons allows for the testing of pharmacological responses, giving researchers a controlled environment to explore neuronal resilience or vulnerability.
"Neuronal culture significantly contributes to our grasp of brain functioning, acting as a bridge between theoretical neuroscience and practical applications."
In light of these developments, it's clear that hippocampal neuron culture is not just a laboratory task; it is a window into the brain's vast operations. As we continue to refine these methodologies, the potential for groundbreaking discoveries in both basic and translational neuroscience remains boundless.
Foreword to Hippocampal Neuron Culture
The exploration of hippocampal neuron culture stands as a cornerstone in the field of neuroscience, particularly in understanding the complex architecture of the brain and its functional dynamics. This article aims to illuminate the methods and insights derived from culturing these neurons, which are integral to not just basic research but also translational studies that inform clinical practices.
Historical Background
Hippocampal neuron culture didn't spring into existence overnight. Its roots trace back to early neurobiology, with foundational work conducted in the mid-20th century. Researchers like Karl Deisseroth and others brought forth seminal findings, establishing baseline protocols for isolating and maintaining neuronal cultures. Over the decades, advancements in culture techniques and technology have profoundly influenced our capacity to study neurons in a controlled environment.
Following a path lined with trial and error, scientists have enhanced methodologies, leading to more effective neuronal cultures. Today, we have a rich tapestry of both historical knowledge and cutting-edge processes that are continuously evolving, shaping the landscape of neuroscience.
Importance in Neuroscience Research
The relevance of hippocampal neuron culture cannot be overstated. Firstly, it allows for the examination of neurons outside the intricate environment of the brain, making it possible to observe their behavior under various experimental conditions. This detachment highlights their physiological and pathological characteristics, offering a lens into phenomena like synaptic plasticity and neurodegeneration that are pivotal to understanding memory and learning processes.
Some key benefits include:
- Controlled environment: Offering a setting to manipulate various factors like nutrients and toxins, which can significantly affect neuron viability and function.
- Model systems: Acting as a robust model for diseases such as Alzheimer's, where neuronal death and dysfunction can be meticulously studied in vitro.
- Drug testing: Serving as a platform for pharmacological testing, helping to identify potential therapeutic agents faster than traditional models would allow.
Through these lenses, the significance of culturing hippocampal neurons becomes clearer. Not only is it foundational for academic inquiry but also a critical step in developing therapeutic interventions in neurobiology. Indeed, the work conducted in this domain not only advances scientific understanding but also possesses the potential to be transformative in clinical settings.
The Anatomy of the Hippocampus
Understanding the anatomy of the hippocampus is crucial for anyone delving into hippocampal neuron culture. The hippocampus is not just another structure in the brain; it's the centerpiece for various cognitive functions and neurological studies. Its anatomy influences how neurons develop, function, and respond to external stimuli. When we engage in neuron culture, being familiar with the intricate layout of the hippocampus helps in effectively selecting isolation and culture methods that best represent the in vivo conditions of these neurons. This relevance can’t be overstated, especially in the context of understanding diseases such as Alzheimer’s, where hippocampal function is significantly impaired.
Hippocampal Structure and Function
The hippocampus is divided into distinct regions: the dentate gyrus, CA1, CA2, and CA3. Each of these areas serves specific roles but is also integrated into a larger neural network. For instance, the dentate gyrus plays a pivotal role in generating new neurons, a process termed neurogenesis. The CA regions, particularly CA3, are integral for associating memories. This architecture is not merely decorative; it reflects the functional diversity essential for learning and memory processing.
Within the hippocampus, neurons are arranged in a manner that facilitates communications between different regions. For instance, the pyramidal neurons in the CA1 and CA3 layers are characterized by a unique morphology that enhances synaptic plasticity, a fundamental component in learning. Through long-term potentiation (LTP) and long-term depression (LTD), these neurons adapt to stimuli, a process reliant on their structural integrity.
One important consideration in neuron culture is the maintenance of the specific morphological features that are vital for synaptic interactions. It’s not enough to simply grow neurons; how they are constructed and how they function in harmony with neighboring cells sets the stage for effective research outcomes.
Role in Learning and Memory
The hippocampus is often dubbed the brain's "save button." It’s where short-term memories transition into long-term storage. This function underpins its critical role in learning processes—whether it's navigating a new environment or recalling a significant event in life.
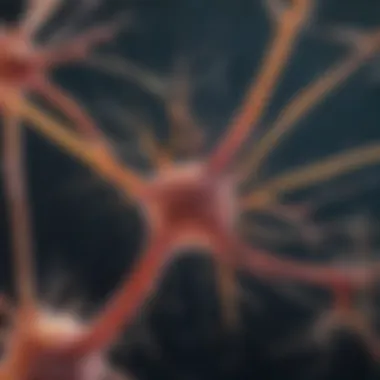
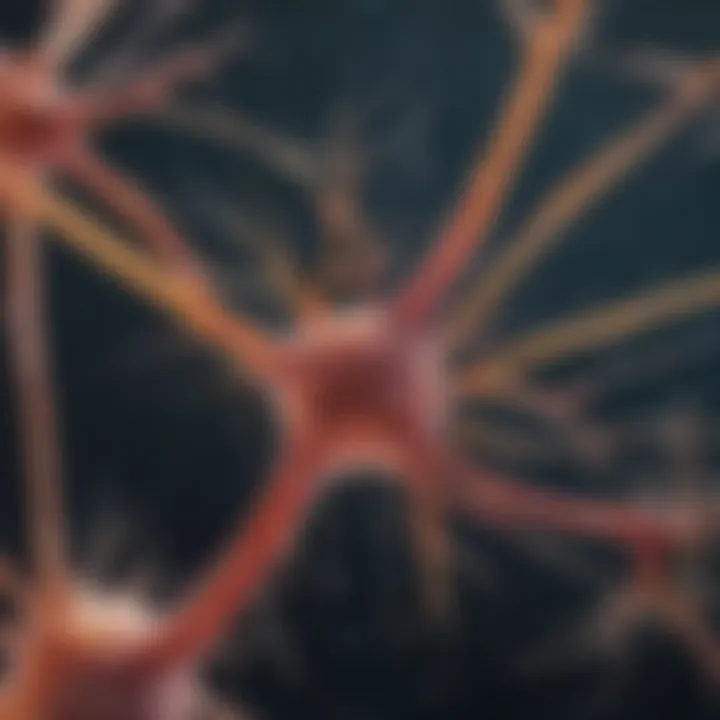
Research indicates that damage to the hippocampus can lead to profound memory deficits. This underscores the necessity of investigating hippocampal neurons not merely as isolated entities but as participants in a broader cognitive landscape. The findings in hippocampal neuron cultures can shine light on memory formation and retrieval. Hence, understanding the context and the role these neurons play in learning emphasizes the relevance of maintaining their biological integrity during culturing.
Moreover, interdisciplinary approaches utilizing advancements in genetic tools can fine-tune our understanding of the hippocampus—allowing researchers to dissect the cellular mechanisms that underlie memory and learning more precisely. This integration of genetic insights with foundational anatomical knowledge paves the way for innovative methodologies in hippocampal neuron research.
In summary, the anatomy of the hippocampus is not just a backdrop; it profoundly influences how memory and learning can be modeled and studied through cultured neurons. The structure provides not only a canvas but also a critical lens through which one can view the dynamic interplay of neuronal interactions—essential for both basic neuroscience research and potential translational applications.
Isolation Techniques for Hippocampal Neurons
The process of isolating hippocampal neurons is foundational for research that aims to understand the complexities of the brain. This section emphasizes the significance of these techniques in ensuring high-quality cultures that reflect the in vivo environment of the neurons. Proper isolation is essential to eliminate non-neuronal cells and contaminants, which can skew experimental results. By employing correct isolation methods, researchers can derive insights that better predict neuronal behavior, paving the way for advancements in neurobiology.
Dissection Methods
Dissection methods for isolating hippocampal neurons can vary somewhat depending on the goals of the study. Traditional techniques involve the manual dissection of brain tissue from embryos or neonates. Utilizing a precise toolset like tweezers and micro-scissors allows researchers to carefully extract the hippocampus from surrounding structures. This hands-on approach provides considerable control over tissue harvesting, but it requires a steady hand and good practice to avoid introducing damage that could compromise the health of the neurons.
- Considerations:
- Cleanliness is paramount; any contaminants introduced during dissection can lead to culture failure.
- Keeping the dissected tissue on ice or in cold solutions helps preserve viability.
- Familiarity with anatomical landmarks assists in accurately locating the hippocampus, making the dissection more straightforward and effective.
Enzymatic Dissociation
Enzymatic dissociation is another highly regarded technique for isolating neurons, especially when dealing with older animals or larger specimens. This method typically involves using enzymes such as papain or trypsin, which facilitate the breakdown of proteins holding cells together. The key advantage here lies in the gentleness; whereas mechanical dissociation can damage delicate cellular structures, enzymatic methods generally preserve cellular integrity.
- Benefits of Enzymatic Dissociation:
- Higher yield of viable neurons is often achieved compared to physical methods.
- Flexibility in timing allows researchers to digest tissues at their own pace, adapting to experimental needs.
- It enables isolation from more varied tissue types, expanding the possible scope for research.
It’s critical to adhere to optimized enzyme concentrations and incubation times, as over-digestion can lead to cell death. Most protocols recommend a careful balancing act of these factors to achieve the desired results without jeopardizing neuron survival.
"The right isolation method can mean the difference between a successful experiment and one that produces questionable data."
Culture Methods for Hippocampal Neurons
The section about culture methods for hippocampal neurons focuses on the core techniques that ensure successful growth and maintenance of neurons in vitro. This is not just an academic exercise; the ability to cultivate these neurons under controlled conditions allows researchers to explore critical questions regarding neuronal behavior, synaptic formation, and responses to various treatments. Working with cultured hippocampal neurons provides insights into the underlying biochemistry and physiology essential for understanding broader neurological functions and pathologies. The delicacy of this process cannot be overstated; choosing the right methods can be make or break for any experimental setup.
Adhesion and Substrate Coating
When culturing neurons, ensuring proper adhesion to the substrate is crucial. Neurons need to attach firmly to the surfaces they grow on to thrive and develop their intricate networks. Using substrate coatings such as poly-D-lysine or laminin is common practice. These coatings promote neural adhesion and signal transduction, crucial for their growth and functioning.
- Poly-D-lysine: This synthetic polyamine positively charges the substrate, encouraging the negatively charged cell membranes of the neurons to attach. It’s essential for preventing neuron apoptosis during the initial seeding phase.
- Laminin: A glycoprotein from the extracellular matrix, laminin encourages neuronal differentiation and survival rates. Its structural significance helps in the formation of synapses, vital for studies in neural transmission.
"Choosing the right substrate significantly affects the morphology and function of cultured neurons."
When applying these substrates, researchers need to consider factors such as concentration and incubation time to ensure optimal efficacy. Too little might not promote sufficient adhesion while too much can induce toxicity. The balance is delicate and often requires optimization based on specific experimental conditions.
Nutritional Requirements
After establishing a stable adherence culture, the next step is to ensure that the nutritional needs of the cultured neurons are met. Neurons are demanding in terms of their metabolic requirements.
- Basal Medium: The choice of basal medium, such as Neurobasal or DMEM, is vital. These media provide the essential nutrients, vitamins, and salts required for neuronal cells to grow and function.
- Serum-Free Supplements: It’s also common to use supplements like B-27 or N-2, which enhance the viability of neurons. These formulations include essential growth factors and hormones that support neuronal health and growth.
In practice, maintaining the balance of glucose, amino acids, and growth factors is critical. Too little glucose can shut down neuronal metabolism, while too much may lead to harmful byproducts that adversely affect cell health. Keeping an eye on pH levels and osmolality is also important, as neurons are sensitive to changes in their environment.
Maintenance of Cell Viability
Long-term maintenance of neuron cultures presents its own set of challenges. Neurons in culture can quickly lose viability if not properly monitored and maintained. Cell viability is influenced by multiple factors, and taking proactive steps to ensure their health over time is imperative.
- Regular Feeding: Changing the culture medium regularly helps to remove waste products and provide fresh nutrients. Depending on the density of neurons and their consumption rate, medium changes might be required every 2-3 days.
- Temperature and CO2 Levels: Neurons thrive in a specific temperature range (typically around 37°C) and need a controlled atmosphere with about 5% CO2. These conditions resemble the in vivo environment and foster optimal growth.
- Minimizing Mechanical Stress: When handling neuron cultures, it’s important to be gentle. Mechanical stress can lead to cell death or damage. Pipetting should be done slowly, and tools should be used with care to avoid disturbing the cultures unduly.
Maintaining viability isn't just about surviving; it’s about promoting growth and functionality. Careful monitoring and adjustments can help preemptively address issues of cell degeneration and maintain rich, vibrant cultures for research purposes.
Optimization of Neuron Culture Conditions
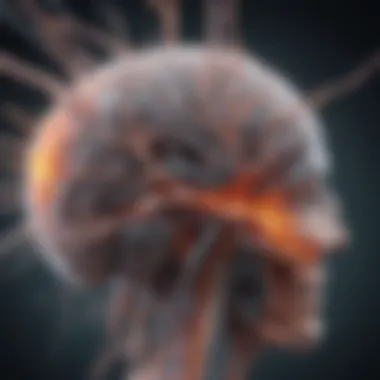
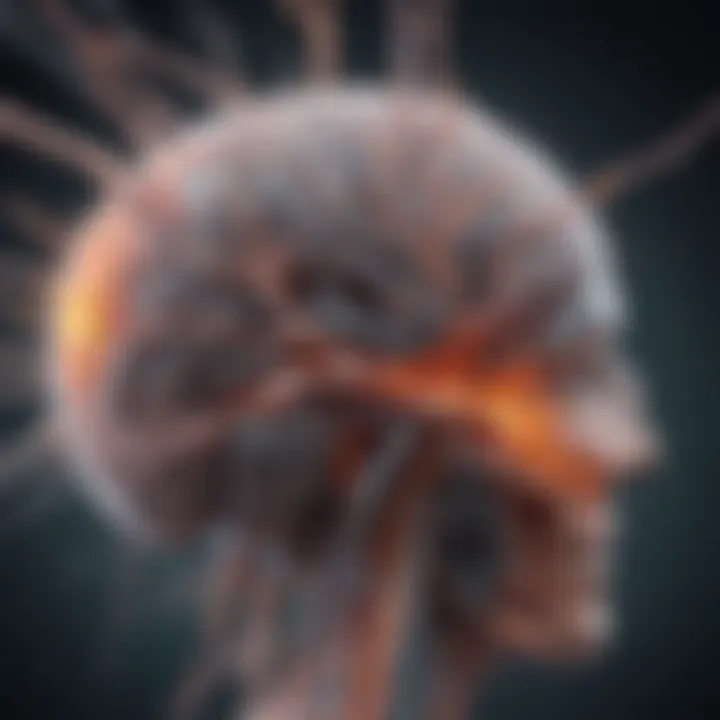
Optimizing neuron culture conditions is fundamental in ensuring that hippocampal neurons grow and function correctly. Without the right environment, even the most carefully isolated neurons can suffer from poor viability, limited growth, or altered functionalities. By fine-tuning various parameters like temperature, pH, and supplementation, researchers can create an optimal setting that mirrors the physiological conditions of the brain. This section delves into specific strategies involving adjustments in temperature and pH levels, as well as supplementation tactics, each of which plays a pivotal role in maximizing neuron health and performance.
Adjusting Temperature and pH
Temperature and pH are two critical factors influencing neuron cultures. Neurons thrive in a narrow temperature range, typically around 37°C, consistent with the normal body temperature of mammals. Deviations can lead to detrimental effects. For instance, temperatures exceeding 37°C may accelerate cell metabolism but lead to significant cell death if sustained. Conversely, temperatures that dip too low can slow metabolic processes, impairing growth and instigating stress responses.
Maintaining the correct pH is equally vital, with most hippocampal neurons requiring a pH level of around 7.2 to 7.4 for optimal performance. An overly acidic or basic environment can disrupt cellular respiration and impede neurochemical processes. Regular checks using calibrated pH meters are essential. Some labs use buffers in their culture media to stabilize pH levels, ensuring a steady environment throughout the culture period.
Supplementation Strategies
Supplementation strategies are necessary to provide essential nutrients and growth factors that hippocampal neurons need for healthy growth and functionality. Here are some key points regarding supplementation that any researcher should consider:
- Neurotrophic Factors: Adding substances like Brain-Derived Neurotrophic Factor (BDNF) or Nerve Growth Factor (NGF) can significantly enhance neurite outgrowth and synaptic development. These growth factors can help neurons communicate better and are instrumental in establishing a network in culture.
- Essential Nutrients: Neurons require a variety of nutrients, including amino acids, vitamins, and minerals. Commonly used media like Neurobasal medium often have added supplements such as B-27 or N-2, designed specifically for neuronal cells. These media compositions help mimic the in vivo environment and support neuronal health.
- Organic Compounds: Some studies suggest that adding compounds like glutamate or glucose can further optimize neuron performance by providing the necessary fuel for cellular processes.
- Regular Media Changes: Frequent media changes, perhaps weekly, help to remove waste products and replenish nutrients in the culture environment, reinforcing neuron viability and activity.
"Properly optimizing culture conditions is not merely a technical task; it is an art that requires attention to detail and an understanding of the peculiar needs of neuron cultures."
By judiciously adjusting temperature, pH, and supplementation, researchers can create an inviting milieu for hippocampal neurons, facilitating groundbreaking studies in cellular neurology and pathology. As the field of neuroscience continues evolving, these foundational techniques remain essential for unlocking the mysteries of neural functioning and therapies.
Characterization of Cultured Neurons
Characterizing cultured neurons is a cornerstone of neuroscience research, playing a pivotal role in not just understanding basic neuronal properties but also in exploring their implications in various neurological disorders. Characterization allows researchers to assess both the quality of neuron cultures and their utility in experimental applications. Accurate characterization informs scientists about the physiological state of the neurons, whether they are maintaining typical synaptic properties or exhibiting signs of stress. This assessment is critical for ensuring that the cultures are representative models of in vivo conditions, which is essential for translating findings into clinical settings.
Morphological Assessment
Morphological assessment involves the examination of the physical characteristics of neurons, which can provide insights into their health and functionality. Using staining techniques such as immunofluorescence or phase contrast microscopy, researchers can analyze several features:
- Cell body size: An increase or decrease in the size can indicate cellular distress or viability.
- Dendritic arborization: An extensive dendritic network is crucial for synaptic connectivity. The complexity of dendritic branches correlates with the neuron's readiness to form synapses.
- Axonal growth: Evaluating the length and branching of axons helps determine if the neurons are successfully projecting their signals to other cells.
Furthermore, changes in morphology might signal neurodegeneration or the response to treatments. For example, long-term culture or exposure to toxic substances can lead to retraction of dendrites or degradation of axons, compromising the neurons’ functionality. Therefore, periodic morphological assessment becomes a routine part of maintaining neuron cultures, enabling researchers to catch issues before they affect experimental outcomes.
Functional Testing
Functional testing complements morphological assessment by evaluating the electrophysiological properties of the neurons. This aspect is key in discerning whether the neurons can effectively perform their functions, such as firing action potentials and transmitting signals. Here are some common functional tests used in research:
- Patch-clamp recording: This technique measures ionic currents through individual ion channels, allowing researchers to characterize specific properties such as excitability and synaptic transmission.
- Calcium imaging: By utilizing fluorescent indicators, scientists can monitor intracellular calcium levels, which are crucial for synaptic activity and various cellular functions.
- Electrophysiological assays: These tests can assess synaptic activity by recording responses to stimuli, determining both the postsynaptic and presynaptic interactions within neuronal networks.
Understanding the functional repertoire of cultured neurons is vital for elucidating the mechanisms behind neuroplasticity and assessing the impact of pharmacological agents. When neurons exhibit robust functional characteristics, they offer a reliable platform for examining the underlying neurobiological processes.
In wrapping up the section, it’s clear that both morphological and functional assessments are integral for validating the success of hippocampal neuron cultures. The interplay between what is observed morphologically and what can be measured functionally helps to create a well-rounded understanding of the cultured neurons, ultimately guiding future research directions.
Challenges in Hippocampal Neuron Culture
Culturing hippocampal neurons can be akin to walking a tightrope; the balance between optimal conditions and various potential pitfalls is fragile yet critical. This section delves into the notable challenges faced during the culturing process. Addressing these matters not only sharpens the understanding of neuron behavior but also enhances the reliability of experimental outcomes.
Contamination Issues
Contamination in neuron cultures is like an unwelcome guest that shows up uninvited, undermining the entire experiment. There are several types of contamination to be aware of:
- Microbial Contamination: Bacteria and fungi can quickly compromise cell cultures. They outcompete neurons for nutrients, leading to cell death. Maintaining sterile conditions is paramount.
- Cross-contamination: Mixing of cell lines can skew results and lead to ambiguous findings, making it crucial to keep cultures distinct and well-labeled.
- Chemical Contamination: Residual chemicals from previous experiments or improper cleaning of equipment can introduce toxins into culture media, negatively affecting cellular health.
Prevention strategies include rigorous disinfection practices, using antibiotics judiciously, and double-checking that all materials are sterilized. Even implementing a culture room set up solely for neuron work can pay dividends.
“In the realm of neuron culture, consistency is the golden rule. One slip can turn a breakthrough into a breakdown.”
Cell Death and Senescence
Cell death is a natural part of cellular life, but in cultured hippocampal neurons, it can present significant challenges. Neurons are particularly sensitive to their environment. There are a few main factors to consider:
- Nutrient Deprivation: When cells aren't getting the right supply of nutrients, it’s like depriving them of a good meal. Inadequate glucose, amino acids, or growth factors can trigger cell death.
- Oxygen levels: While neurons are oxygen-hungry cells, too much or too little can lead to hypoxia or oxidative stress. Both conditions can spiral cell health downward in no time.
- Senescence: Over time, cultured neurons may enter a state of senescence—a sort of biological aging—not necessarily leading to immediate cell death but significantly impairing functionality. This state can complicate experimental results and may necessitate regular passaging or careful monitoring.
To mitigate these risks, it is essential to maintain precise control over culture conditions, regularly replenish media, and keep a keen ear on any changes in neuron behavior; subtle shifts could signal bigger issues down the line.
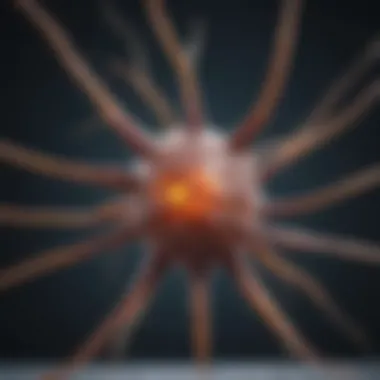
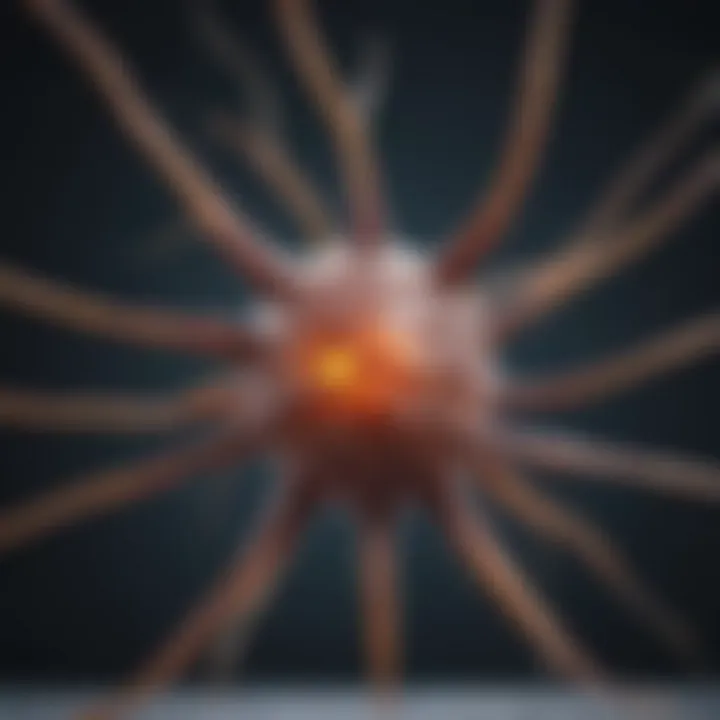
In sum, the intricacies of culturing hippocampal neurons extend beyond basic methodologies. Addressing contamination and cell viability challenges bolsters not just the integrity of scientific findings but also enriches our understanding of neuronal health and diseases.
Applications of Hippocampal Neuron Culture
The applications of hippocampal neuron culture serve as a crucial pillar in the landscape of neuroscience research. The ability to cultivate neurons from the hippocampus enables scientists and researchers to study various phenomena related to brain function, diseases, and disorders. These applications are pivotal in increasing our understanding of complex neurobiological processes, providing unique opportunities to explore how neurons behave under controlled conditions. This section covers two major areas: studying neurodegeneration and drug screening and toxicology.
Studying Neurodegeneration
Neurodegenerative diseases such as Alzheimer’s, Parkinson’s, and Huntington's are characterized by the progressive loss of neuronal function and structure. By using cultured hippocampal neurons, researchers can mimic aspects of these diseases in vitro. This approach permits a detailed examination of the mechanisms and changes that occur during degeneration.
- Pathological Insights: Cultured neurons allow for the observation of cellular responses to neurodegenerative markers. For instance, using amyloid-beta in the culture can help in studying Alzheimer’s pathology.
- Toxicity Testing: Various toxins can be introduced to the culture to evaluate their effects on neuronal health and function, aiding in the identification of potential therapeutic targets.
- Protein Aggregation: The study of protein misfolding and aggregation, which plays a significant role in neurodegenerative conditions, can be performed within these in vitro systems.
Experiments that leverage hippocampal neuron cultures can lead to breakthroughs in understanding how neurodegeneration unfolds and what interventions may halt or reverse these processes.
Drug Screening and Toxicology
Another prominent application of cultured hippocampal neurons is in drug screening and toxicology. The pharmaceutical industry benefits greatly from this approach as it provides a reliable platform for evaluating new compounds before clinical trials.
- Efficacy Assessment: Cultured neurons help in determining the effectiveness of potential drugs. By monitoring changes in synaptic activity or neuron morphology, researchers gain insights into how a drug alters neural function.
- Safety Evaluation: Toxicity studies are essential in the drug development process. Hippocampal neuron cultures serve to assess the potential harmful effects of a drug. For example, understanding how a drug triggers cell death or induces oxidative stress is critical.
- Personalized Medicine: With advancements in tissue engineering and stem cell technology, there is potential for developing patient-specific neuron cultures. This means that drug responses can be evaluated based on individual genetic backgrounds, leading to tailored therapeutic strategies.
"In essence, the applications of hippocampal neuron culture extend far beyond mere observations; they form the basis for novel therapies and critical insights into brain health and disease."
Cultured hippocampal neurons are invaluable for advancing our knowledge of neurobiology. As methodologies improve and new technologies emerge, the potential for groundbreaking discoveries related to neurons and their role in the nervous system will only grow.
Future Directions in Hippocampal Neuron Research
The landscape of hippocampal neuron research is rapidly evolving, and staying ahead of the curve has become crucial for advancing our understanding of this intricate aspect of neuroscience. Future directions in this field hold promise for both scientific discovery and translational applications. By focusing on innovative culture techniques and the integration of genetic tools, researchers are not just looking to enhance cell viability in vitro but also gain deeper insights into neuronal behavior and pathology.
Innovative Culture Techniques
As the saying goes, "there’s always room for improvement," and this rings particularly true regarding neuron culture methodologies. New techniques, such as 3D neuronal cultures and organoids, are gaining traction. These methods allow for a more biologically relevant microenvironment, leading to more accurate modeling of neuronal behavior.
- 3D Cultures: Traditional 2D cultures can limit the growth and interactions of neurons. By cultivating neurons in a three-dimensional context, we can replicate more natural cellular environments.
- Microfluidic Devices: Utilizing microfluidics can compartmentalize different neuron types or introduce pharmacological agents in a controlled manner. This precision allows scientists to observe cellular interactions or drug responses in real time.
- Benefits: This fosters better synaptic connectivity and axonal growth, aligning more closely with in vivo conditions.
- Considerations: Researchers must pay attention to factors like nutrient diffusion and waste removal.
- Benefits: Leads to better reproducibility and a finer resolution of experimental outcomes.
- Considerations: Development and maintenance of these devices can be technically demanding.
Exploring these innovative techniques is vital for pushing the boundaries of current research and offers a window into more complex cellular interactions that traditional methods may overlook.
Integration with Genetic Tools
In the world of neuroscience research, integrating genetic tools into neuronal culture practices has opened up new avenues of exploration. Harnessing the power of CRISPR and other gene-editing technologies is key to manipulating neuronal populations with pinpoint accuracy. The implications are profound.
- Gene Editing: By employing CRISPR-Cas9, scientists can induce specific mutations or correct genetic aberrations. This allows for the direct observation of phenotypic changes in neurons and their associated functions.
- Transgenic Models: Creating transgenic mice or cultured neurons that express fluorescent markers can facilitate the visualization of developmental processes, synaptic dynamics, and even aid in the study of neurodegenerative diseases.
- Benefits: It provides clarity on the genetic basis of neurological diseases and conditions.
- Considerations: Ethical concerns and off-target effects need to be navigated carefully.
- Benefits: This approach allows for tracking neuronal activity over time.
- Considerations: The generation of such models can be resource-intensive.
Integrating these genetic tools not only enhances neuron culture but also fortifies our approach to understanding neurobiology. As we break through the barriers in technology and methodology, we'll likely uncover the molecular mechanisms underlying various neurological disorders.
"The future belongs to those who believe in the beauty of their dreams." - Eleanor Roosevelt
In summary, the future directions in hippocampal neuron research signal an era rich in potential. By harnessing innovative culture techniques and integrating genetic manipulation tools, the depth of our understanding will not only broaden but also refine our approach to tackling neurological diseases. With careful consideration and persistent curiosity, researchers can pave the way for significant advancements in neuroscience as a whole.
Finale
In the realm of neuroscience, the cultivation of hippocampal neurons stands as a pivotal undertaking. It opens doors to understanding both the fundamental workings of the brain and the potential pathways to address neurological disorders. Notably, this process not only enhances our comprehension of neural behavior but also serves as a critical model for drug development and neurodegenerative research. By meticulously isolating and cultivating these neurons, researchers embark on a voyage that provides insights into the complexities of synaptic plasticity and neural connectivity.
Summarizing the Importance of Hippocampal Neuron Culture
Hippocampal neuron culture harbors vast significance in neuroscience for various reasons:
- Understanding Mechanisms of Learning and Memory: The hippocampus plays a central role in memory formation. Exploring cultured neurons allows scientists to dissect molecular processes behind memory and learning.
- Testing Therapeutic Approaches: Cultured neurons serve as a platform for testing the efficacy of novel drugs, helping in the fight against conditions such as Alzheimer's and Parkinson's disease. The ability to observe cell responses in real time contributes immensely to translational research.
- Modeling Disease States: These cultures can simulate various pathological conditions, rendering them invaluable for studying neurodegenerative diseases, thus enabling researchers to formulate targeted interventions.
- Investigating Synaptic Plasticity: Insights garnered from these cultures contribute to our understanding of how synapses strengthen or weaken over time, which is fundamental to learning processes and behavioral adaptation.
"The journey through hippocampal neuron culture is akin to peeling an onion; each layer unveils deeper complexities and new understandings of neurological phenomena."
In summary, the meticulous work invested in hippocampal neuron culture significantly propels the field of neuroscience forward. It underscores a blend of artistry and science, revealing not just cellular characteristics but also the broader narrative of brain function and dysfunction. As the methodologies and technologies evolve, the insights gained hold promise for both academic exploration and clinical applications.