Exploring the Interlocking Technique: An In-Depth Analysis
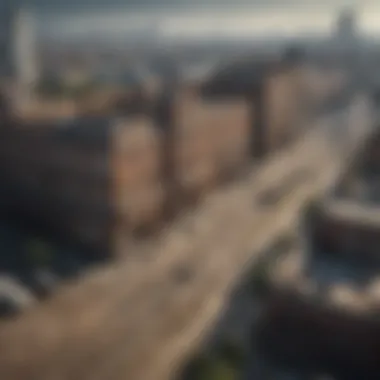
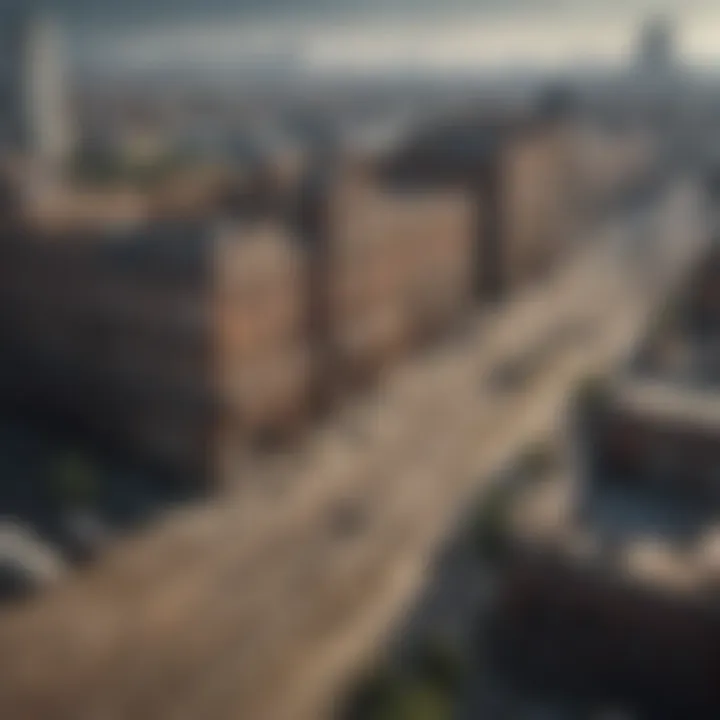
Research Background
The interlocking technique has emerged as a significant methodology across various disciplines. This technique provides a solution to complex structural, biological, and material challenges. Historically, the problem of integrating multiple elements into a cohesive unit has fascinated scientists and engineers alike. This integration not only enhances functionality but also improves the resilience of structures and systems against failure.
In structural engineering, the interlocking technique facilitates innovative designs that withstand both static and dynamic loads. For instance, ancient architectural marvels used principles akin to interlocking elements to enhance stability. The broader concept can be traced back to Italy's medieval architecture, where stones were cut to fit without mortar, creating strong and durable structures. Similarly, in biology, the interlocking of proteins and cellular structures has led to advancements in understanding disease mechanisms and developing synthetic biology. Previous studies highlight researchers' focus on how these interconnections can lead to breakthroughs in both fields.
Findings and Discussion
The results of recent studies on the interlocking technique reveal several key insights. Firstly, the technique enhances structural integrity across various applications. By understanding how components fit together, designers can optimize load distribution.
Additionally, in materials science, the interlocking design has developed new composite materials. Such materials exhibit superior mechanical properties compared to traditional structures. These insights suggest a substantial shift in how engineers and scientists think about design.
The interpretation of these findings indicates that the interlocking technique is not merely a design choice but a fundamental principle that can dictate future research directions. As researchers explore its application further, the potential benefits are wide-ranging.
"The interlocking technique, through its diverse applications, not only solves existing problems but also fosters innovation in uncharted realms of science."
Future prospects indicate a growing trend in employing the interlocking technique in advanced fields like nanotechnology and robotics. The evolution of this approach reflects a continual improvement in our ability to solve complex scientific challenges.
Foreword to the Interlocking Technique
The interlocking technique represents a significant advancement in various scientific disciplines. It has gained attention due to its effectiveness in enhancing structural integrity, efficiency in load distribution, and potential for innovative designs. Understanding this technique provides key insights into its applications not just in engineering, but also in biological systems and materials science.
The notion of interlocking involves the integration of components in a way that they mutually support and stabilize each other. This differs from traditional bonding methods, emphasizing mechanical interdependence rather than purely adhesive properties. This approach ensures resilience under stress, minimizing potential failure points. Thus, it offers a range of benefits that make it appealing for diverse applications.
As we delve deeper into the historical context and fundamental principles, we will see how this technique has evolved and what key elements define its success. The interlocking technique also presents various considerations, such as material choice, load requirements, and environmental factors. Exploring these aspects illuminates the technique's relevance in current and future research and application.
Definition and Overview
The interlocking technique can be defined as a method of joining materials or structural elements to create a cohesive assembly that relies on interdependence for stability. This technique utilizes geometrical designs that allow pieces to fit together without the need for additional fasteners or adhesives. The result is a system where parts are engaged, enhancing both strength and flexibility.
Practically, this method can be seen in various domains, from architectural designs using interlocking bricks to biological systems where cells interact in complex, interlinked networks. The versatility of this technique makes it a vital concept in many fields, highlighting the necessity of understanding its mechanisms and potential applications.
Historical Context
The origins of the interlocking technique can be traced back to ancient architecture and construction. Historical structures often utilized interlocking stone or brick arrangements for durability and stability. For instance, the remarkable construction of the Inca city of Machu Picchu demonstrates the use of interlocking stones that fit together perfectly without mortar. This is not just a testament to creative design but also to the practical benefits of enhanced structural integrity.
In more modern contexts, the evolution of engineered materials in the 20th century incorporated interlocking techniques into various structural applications. Innovations in design allowed for sophisticated interdependencies that improved load distribution, contributing to safer and more resilient constructions.
In science, the technique is relevant in the study of biological systems. For example, various cellular processes exhibit interlocking mechanisms, where cell signaling pathways depend on well-defined interactions. This historical perspective provides a backdrop against which contemporary innovations utilizing the interlocking technique can be examined.
Fundamental Principles of Interlocking
The fundamental principles of interlocking form the backbone of this technique's application in various disciplines. Understanding these principles is crucial for anyone looking to harness the potential of interlocking structures or systems. This section covers key elements, benefits, and considerations of these foundational principles.
Basic Mechanisms
Interlocking relies on specific mechanical aspects. The basic mechanism involves two or more components that fit together without the need for adhesive substances. This fitting can take many forms, including geometric shapes or profiles that allow for a snug connection.
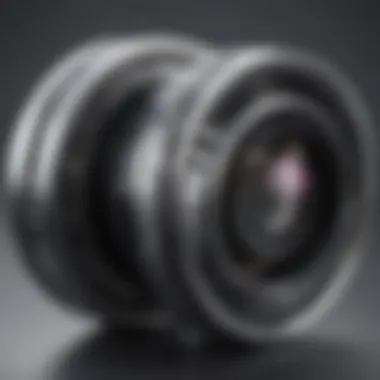
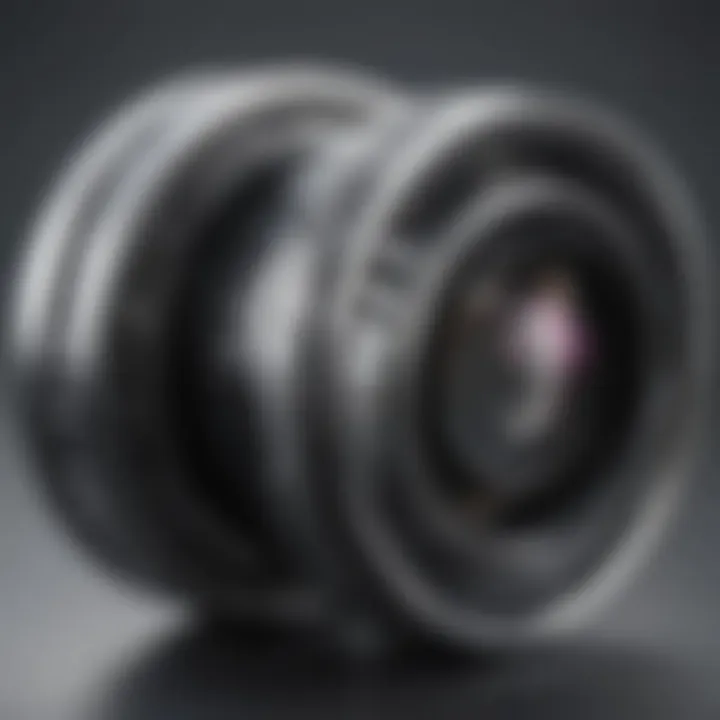
The strength of interlocking arises primarily from friction and contact forces between the interconnected parts. When two parts are interlocked, they distribute loads and stresses more efficiently compared to traditional fastening methods. This makes interlocking systems desirable for applications requiring high integrity and stability.
Key mechanisms include:
- Tension and Compression: Interlocking systems often operate within these two fundamental forces. They can hold substantial loads while distributing stress evenly throughout the structure.
- Friction Resistance: The interlocking points create areas of high surface contact, which significantly resist slippage.
- Geometric Design: The shapes utilized in interlocking systems are often engineered to enhance stability, reducing the likelihood of failure.
Material Properties Involved
The success of interlocking is significantly influenced by the materials used in its composition. Each material presents unique properties that can either enhance or limit the effectiveness of the interlocking technique.
Important material properties to consider include:
- Strength: High tensile and compressive strength materials are preferred as they can endure significant loading without deformation.
- Ductility: Materials with good ductility can absorb more energy and thus may sustain interlocking structures under dynamic loads.
- Durability: Resistance to environmental factors such as moisture and temperature changes is essential in maintaining the integrity of interlocking connections over time.
- Weight: Lightweight materials can enhance ease of handling and installation while still providing the necessary strength and stability.
In summary, understanding the fundamental principles of interlocking reveals its efficacy and broad applicability. By taking into account the basic mechanisms and the material properties involved, practitioners can achieve impressive levels of structural performance.
Applications Across Disciplines
Understanding the applications of the interlocking technique across various disciplines is essential for appreciating its multifaceted impact. This technique is increasingly integral in fields like engineering, biology, and materials science. By examining its diverse usages, we can highlight the advantages it brings to problem-solving and innovative design.
Usage in Engineering
Structural Integration
Structural integration refers to the method by which interlocking elements come together to provide enhanced stability and strength in engineering designs. This type of integration is fundamental as it allows for the construction of frameworks that can withstand various forces. A key characteristic of structural integration is its ability to distribute stress evenly, reducing the risk of failure.
The interlocking technique is a beneficial choice in structural integration because it maximizes load-bearing capacities without the excessive use of materials. This results in more efficient use of resources and minimizes waste. The unique feature of structural integration is its potential for modular construction. Using prefabricated interlocking components can speed up projects while ensuring high-quality construction. However, one must consider the precision required in manufacturing these components, as any misalignment can compromise structural integrity.
Load Distribution Techniques
Load distribution techniques are crucial for managing how forces act on structural components. Interlocking can effectively distribute loads in a way that prevents localized stress concentrations. One significant characteristic of these techniques is their adaptability to various structures, allowing for customized design solutions.
Load distribution techniques using interlocking components are beneficial because they enhance the resilience of structures under dynamic loads, such as earthquakes or winds. A unique feature is how these techniques can integrate with traditional building materials, offering a hybrid approach that enhances overall performance. Nevertheless, understanding how to implement these techniques can be complex and requires specialized knowledge to ensure optimal results.
Biological Applications
Biological applications of interlocking techniques reveal their versatility beyond engineering. This area examines how interlocking principles can influence cellular dynamics and tissue functionality.
Cellular Mechanisms
Cellular mechanisms involve the interactions between cells that affect growth, repair, and functionality. Understanding how cells interlock at the molecular level can provide insight into how tissues maintain integrity. A critical feature of cellular mechanisms is their role in cellular communication and cohesion. This interconnectedness is essential for forming healthy tissues and preventing degenerative conditions.
This exploration is essential because it can lead to advancements in medical treatments and therapies. The unique aspect of cellular mechanisms is their ability to adapt to environmental changes, an advantage in understanding disease progressions. Challenges remain in manipulating these mechanisms for therapeutic purposes, as the complexity of cellular interactions poses difficulties.
Tissue Engineering
In tissue engineering, the interlocking technique is utilized to create scaffolds that promote cell growth and tissue development. This area contributes to the overall goal of repairing or replacing damaged tissues. A key characteristic of tissue engineering frameworks is their bio-compatibility, allowing for safe interactions with living cells.
Tissue engineering is a powerful choice for regenerative medicine, as it provides the potential for developing organs and tissues. The unique feature of these scaffolds is their capacity for mimicking natural tissue environments. However, producing these constructs at scale can be challenging, and ensuring their long-term functionality in the body is still an area of active research.
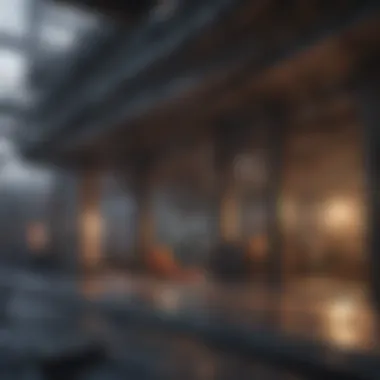
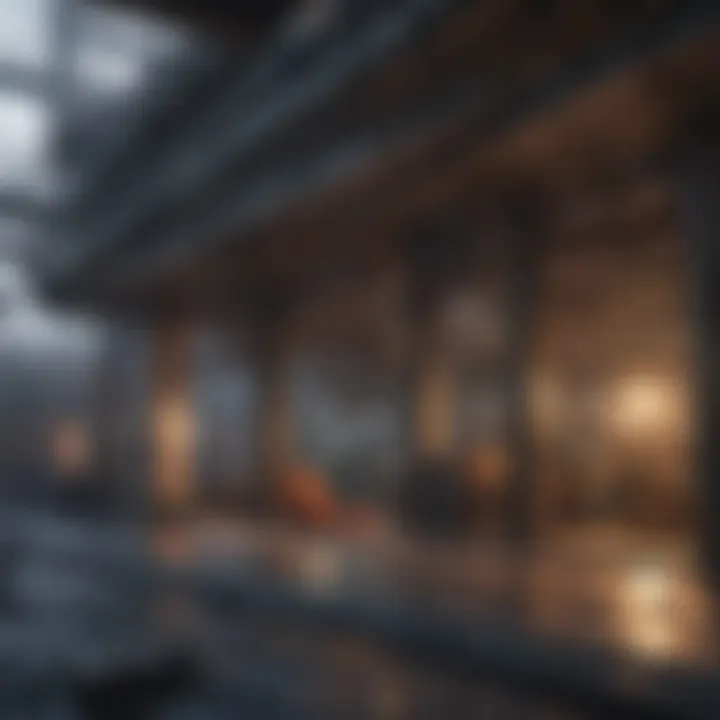
Materials Science Innovations
Materials science is another field significantly influenced by the interlocking technique. This area focuses on how material properties can be enhanced through interlocking structures.
Composite Materials
Composite materials are engineered to optimize specific properties, combining different substances to achieve superior performance. The interlocking technique can enhance the integrity and functionality of these materials. One of the key benefits of composite materials is their ability to resist damage under various stressors, making them suitable for high-performance applications.
By utilizing interlocking design principles, it is possible to improve the durability and lifecycle of composites. A unique feature of these materials is their tailorability, allowing them to meet the precise needs of various applications. Nonetheless, the manufacturing processes for creating effective composites can be costly and time-consuming.
Nanostructures
Nanostructures represent a cutting-edge area where the interlocking technique shows considerable promise. At the nanoscale, materials exhibit unique properties that can be utilized in various applications, from electronics to medicine. One key characteristic of nanostructures is their surface area-to-volume ratio, which greatly affects their chemical and physical properties.
Incorporating interlocking principles into nanostructure design can enhance stability and functionality. The unique attributes of these materials allow for innovation in fields such as drug delivery and biosensing. However, the challenges in producing and manipulating nanostructures reliably require significant research and development efforts.
Advantages of Using the Interlocking Technique
The interlocking technique offers a range of advantages that significantly enhance its utility across various fields. Understanding these advantages is crucial for researchers and practitioners who seek to implement this technique in their work. The following sections explore three primary benefits: enhanced structural integrity, increased design flexibility, and cost-effectiveness in implementation.
Enhanced Structural Integrity
One of the most critical benefits of the interlocking technique is its ability to improve structural integrity. This aspect is essential, especially in disciplines such as civil engineering and architecture, where stability is paramount. The interlocking mechanism allows for effective load distribution, reducing the risk of failure or collapse. Structures designed with interlocking elements can better withstand forces such as wind, earthquakes, or heavy loads.
Moreover, interlocking systems often require fewer joints in construction. This reduction minimizes potential weak points and enhances the overall strength of the structure. Detailed studies have shown that buildings and bridges utilizing interlocking techniques exhibit increased resilience compared to traditional designs. Therefore, from the perspective of safety and reliability, the interlocking technique stands out.
Increased Design Flexibility
Another significant advantage of the interlocking technique is the flexibility it provides in design. Designers can create components that fit together in various configurations. Unlike conventional methods, which may limit creativity due to rigid geometries, interlocking systems allow for a broader exploration of shapes and forms. This flexibility is particularly important in architectural design and advanced manufacturing processes.
For example, in modular construction, interlocking components can be customized easily. This adaptability can lead to more innovative solutions, catering to specific aesthetic and functional requirements. Also, the ease of assembly and disassembly facilitates updates or repurposing of structures as needed. Consequently, this design freedom greatly benefits architects and engineers seeking to create unique and functional spaces.
Cost-Effectiveness in Implementation
Cost is always a consideration in any project. The interlocking technique can offer cost-effectiveness during implementation. By reducing the number of materials and construction processes required, this method can lower overall project expenses. For instance, the interlocking technique often uses materials more efficiently, minimizing waste.
Additionally, as interlocking components fit together seamlessly, the time needed for assembly can be significantly reduced. Construction projects that rely on traditional methods might involve extensive labor and longer timelines. With interlocking techniques, the speed of assembly not only minimizes labor costs but also allows for faster project completion, benefiting stakeholders.
"Cost-effectiveness is crucial in todayβs competitive market, making interlocking techniques appealing for large-scale projects."
Challenges and Limitations
In the domain of the interlocking technique, recognizing its challenges and limitations is fundamental. While this technique offers significant benefits across various fields, understanding the constraints it imposes is equally critical. These challenges can influence both practical applications and theoretical advancements. Addressing these limitations not only aids in refining current practices but also drives innovation, pushing researchers to seek solutions that enhance the robustness of the interlocking technique in real-world settings.
Future Directions in Interlocking Technique Research
The field of interlocking technique research is continuously evolving. As new challenges arise within various disciplines, innovative solutions must follow. This section will explore prospective advancements and interdisciplinary approaches that could reshape the way professionals implement the interlocking technique.
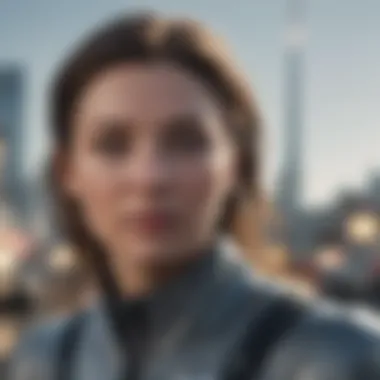
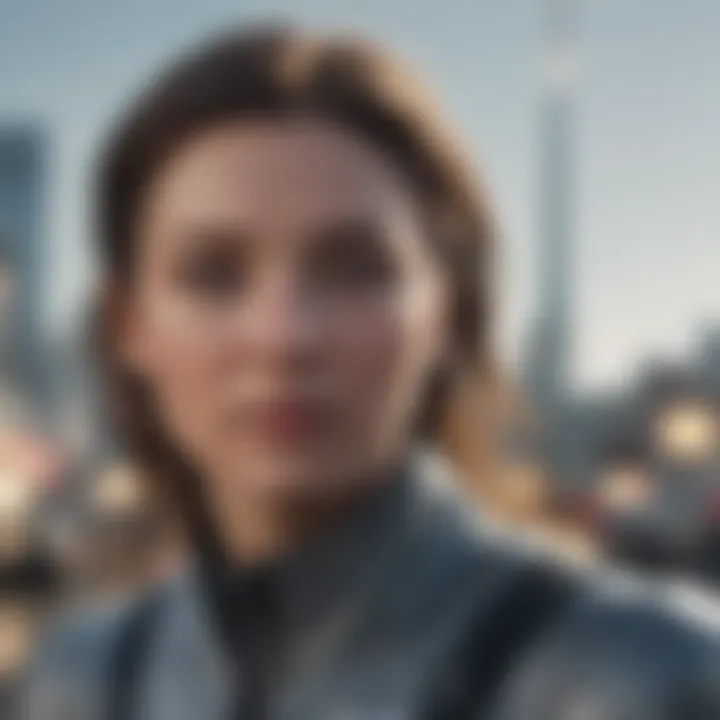
Innovations on the Horizon
Innovations in interlocking techniques focus on improving efficiency and easing execution in various applications. One significant area of development is the integration of smart materials. These materials can adapt their properties in response to environmental changes. For example, incorporating shape-memory alloys can enhance load-bearing structures by allowing them to change shape under stress, optimizing performance.
Furthermore, advancements in 3D printing technologies present opportunities for creating complex, interlocking designs that were not feasible before. This methodology could revolutionize production methods in engineering and biological applications, allowing for more precise customizations. Interested professionals might explore the potential of biomimetic designs, which take inspiration from nature to solve engineering problems. Such designs could lead to more efficient interlocking mechanisms that replicate the resilience seen in biological organisms.
Implementing innovative materials and technologies can streamline processes and enhance the interlocking technique's adaptability across sectors.
Interdisciplinary Approaches
Interdisciplinary collaboration is vital for advancing research in the interlocking technique. By merging expertise from diverse fields, new insights can emerge. For instance, collaborations between engineers and biologists can lead to unique solutions in tissue engineering. Combining knowledge from both disciplines enables the development of interlocking systems that support cell growth while ensuring structural stability.
Additionally, partnerships between artists and scientists could inspire novel designs by incorporating aesthetic considerations into functional interlocking techniques. This connection may not only enhance usability but also improve public engagement with scientific applications.
Incorporating psychological insights into engineering designs helps understand how end-users interact with interlocking systems. Understanding user behavior allows researchers to refine techniques for better usability and integration into existing frameworks.
Finally, fostering collaborations between industry and academic institutions can bridge the gap between theory and practice. Such partnerships often facilitate knowledge transfer, driving both innovation and practical applications in the interlocking technique.
In summary, future directions in interlocking technique research will depend significantly on innovative materials, advanced technologies, and interdisciplinary collaboration. By embracing these elements, the potential for impactful and adaptable applications will expand significantly.
Case Studies Illustrating Success
Case studies play a significant role in demonstrating the practical applications and effectiveness of the interlocking technique across various fields. They translate theoretical concepts into real-world solutions, providing concrete evidence of the benefits and challenges associated with implementing this approach. By examining specific examples, we can gain insights into both the successes and limitations that practitioners face. These case studies not only illustrate how the interlocking technique enhances design and functionality but also help to identify best practices and potential areas for further research.
"Case studies serve as a bridge between theoretical knowledge and real-world application, showcasing the interlocking technique's adaptability and effectiveness."
Structural Engineering Examples
In structural engineering, the interlocking technique has been utilized effectively to create designs that optimize stability and load distribution. One notable example is the construction of the Guggenheim Museum in Bilbao, Spain. The museum's innovative design incorporates interlocking forms that not only provide aesthetic appeal but also ensure structural integrity. The technique allowed architects to distribute loads more evenly across the building, which is particularly important given the unique shape and materials used.
Another relevant case is the development of modular bridges in various urban settings. These bridges feature interlocking components that facilitate easier assembly and disassembly, providing flexibility in design and maintenance. This modular approach has shown to significantly reduce construction time and costs, thereby increasing the overall efficiency of infrastructure projects. The interlocking technique allows for improved resilience against natural forces, showcasing its potential in mitigating risks associated with structural failures.
Biological Research Outcomes
In biological research, the interlocking technique has shown promise in cellular engineering and tissue regeneration. A case study involving the interlocking of hydrogels demonstrated enhanced cell adhesion and growth. Researchers found that the configuration of the interlocking hydrogels created an environment conducive to cell proliferation, thus benefiting tissue engineering applications. This has implications for developing better scaffolding materials that can support tissue regeneration in medical implants.
Further exploration in this area has led to success in creating interlocking biomaterials that mimic natural structures. For example, interlocking patterns were utilized in designing scaffolds for bone tissue engineering. These scaffolds not only facilitate nutrient exchange but also provide the necessary support for cell attachment and growth, ultimately promoting bone regeneration.
These examples underscore the significance of the interlocking technique across different disciplines. By analyzing these case studies, researchers and practitioners can learn valuable lessons that inform their future projects, ensuring continuous innovation and improvement.
Culmination
The conclusion serves as a fundamental part of the article as it synthesizes the vast amount of information presented throughout the text. It highlights the key findings and insights gained from the exploration of the interlocking technique. A well-structured conclusion does more than just summarize; it offers a final perspective that can shape the reader's understanding and implications of the subject matter.
Summary of Findings
In our analysis, we discussed the historical context of the interlocking technique, shedding light on its evolution and significance across several disciplines. Key mechanisms such as properties of materials and structural integration were explored in depth. Furthermore, the advantages of using this technique, including enhanced structural integrity and design flexibility, were highlighted. The challenges faced, involving technical and environmental considerations, were also addressed, providing a balanced view of its practical applicability. Ultimately, the case studies showed real-world success, illustrating the practicality and efficiency of the interlocking method in various applications.
Implications for Future Research
Looking forward, research must continue to delve into the innovations surrounding the interlocking technique. There are opportunities for interdisciplinary approaches to enhance not only its applicability but also its efficiency in real-world situations. Further investigations should focus on refining materials and techniques that leverage the principles of interlocking. Additionally, examining environmental impacts and sustainability will be critical as the urgency for eco-friendly solutions grows. As such, future endeavors in this field could lead to significant advancements, pushing the boundaries of what is currently possible with the interlocking technique in engineering and biology.
"The future of the interlocking technique rests on our ability to adapt and innovate."
In summary, the conclusion reiterates the multifaceted nature of the interlocking technique and its relevance across various sectors. By thoughtfully considering the implications for future research, stakeholders can ensure continued advancement in this vital area.