Exploring MEMS Accelerometer Sensors: Principles & Applications
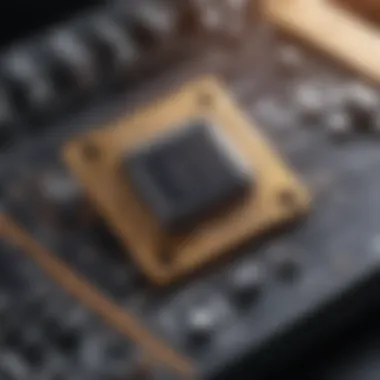
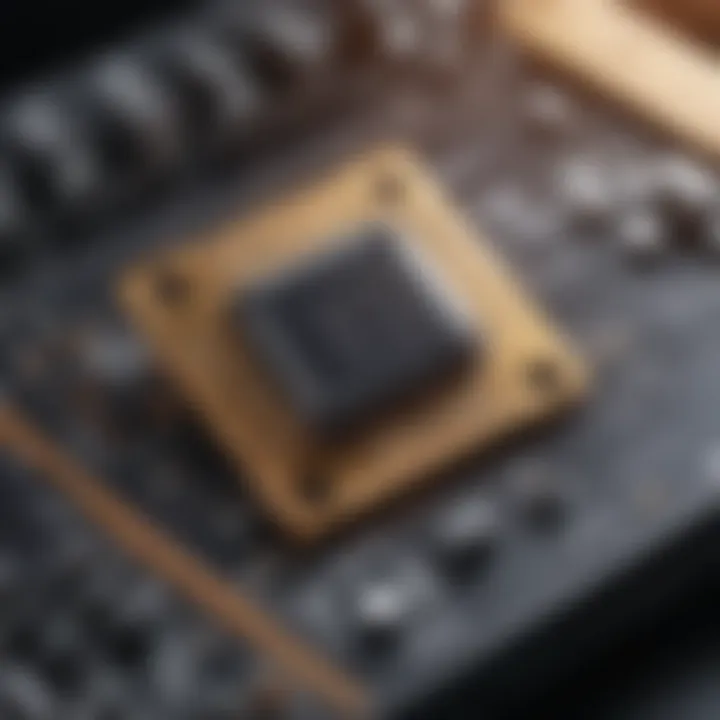
Intro
MEMS accelerometer sensors play a critical role in modern technology. They measure acceleration forces, which can be static, like gravity, or dynamic, caused by motion or vibration. This article delves into the principles that govern these sensors, their various applications across multiple fields, and the latest advancements in technology that continue to shape their functionality.
Research Background
Overview of the scientific problem addressed
The scientific challenge with accelerometer technology revolves around sensitivity, accuracy, and miniaturization. As devices become smaller and more complex, the need for compact sensors that provide precise measurements increases. MEMS, or microelectromechanical systems, present a solution that blends mechanical and electrical components at a microscopic scale. Understanding the mechanics behind MEMS accelerometers is essential for developing more advanced sensors that can enhance the functionality of diverse applications, from consumer electronics to aerospace.
Historical context and previous studies
The journey of accelerometer technology dates back several decades. Early models were based on bulk technology, which resulted in larger, less efficient devices. The transition to MEMS began in the late 20th century, driven by advancements in microfabrication techniques. Notable studies have focused on optimizing the design and performance of MEMS accelerometers, emphasizing the importance of material properties, fabrication processes, and environmental factors. Research has shown that these innovations lead to enhanced stability and functionality in various applications.
As a result, the MEMS accelerometer market has expanded rapidly, attracting significant interest from researchers and industry alike. Recent years have seen explosive growth in areas such as wearable technology, automotive systems, and health monitoring, underscoring the sensor's wide reach and relevance.
Findings and Discussion
Key results of the research
The findings in this field indicate that modern MEMS accelerometers have improved in terms of performance metrics like sensitivity and range. Current designs utilize advanced materials and innovative engineering solutions. For instance, the incorporation of silicon as a primary material allows for higher accuracy and lower power consumption. These aspects are critically examined in recent research articles, highlighting advancements that enable MEMS accelerometers to operate effectively even in challenging environments.
Interpretation of the findings
The implications of these findings are significant. As MEMS accelerometers become more sophisticated, they can be deployed in increasingly challenging applications. Areas such as autonomous vehicles benefit from the precise motion detection capabilities of these sensors. Furthermore, ongoing research aims to integrate machine learning algorithms with sensor data to enhance decision-making processes.
Prelims to MEMS Accelerometers
MEMS accelerometers play a vital role in a variety of modern applications. Their sensors are key in measuring acceleration forces, which can be static or dynamic. Understanding these sensors is increasingly important in fields like consumer electronics, automotive systems, and industrial monitoring. As devices become smarter and more integrated into our lives, the demand for precise motion capture grows. This article will explore various aspects of MEMS accelerometers, providing insights into their working principles, applications, and advancements.
Definition and Overview
A MEMS accelerometer is a miniaturized device that detects changes in motion and orientation. The acronym MEMS stands for Microelectromechanical Systems, which indicates its small size and mechanical functionality. Unlike traditional accelerometers, MEMS devices utilize microfabrication techniques to produce sensors that are significantly smaller and more efficient. These sensors generally consist of a proof mass that moves in response to acceleration, which creates changes in capacitance or voltage, leading to measurable electrical signals.
Historical Development
The evolution of MEMS technology can be traced back to the late 20th century when advancements in semiconductor technology laid the groundwork for miniaturization. Initially, the focus was on developing sensors for the aerospace and automotive industries. In the 1990s, MEMS accelerometers began commercializing, making their way into consumer electronics like smartphones and gaming devices. Today, these sensors are ubiquitous in applications beyond their initial use, including health and fitness trackers as well as industrial automation systems. Understanding this historical context enables us to appreciate current innovations and the future trajectory of MEMS accelerometers.
Fundamental Principles
Understanding the fundamental principles of MEMS accelerometers is essential for grasping their functionality and impact across various industries. These principles form the backbone of how these sensors detect motion and acceleration, enabling their widespread application in diverse fields such as consumer electronics, automotive systems, and health monitoring. The effectiveness of MEMS accelerometers in measuring inertial motion stems from the interplay between mechanical structure and electrical inputs, which results in accurate data collection under varying conditions.
Mechanical Operation
MEMS accelerometers operate based on a mechanical architecture that typically includes a seismic mass suspended by springs. When the device experiences acceleration, this mass moves relative to its housing. This movement can be captured through a variety of methods, including capacitive, piezoelectric, or resistive sensing. The choice of mechanism impacts the device's performance characteristics.
Key aspects of mechanical operation include:
- Inertia: The seismic mass's resistance to changes in motion allows the sensor to detect acceleration accurately.
- Damping: This is used to minimize oscillations and stabilize sensor readings.
By balancing these elements, manufacturers can fine-tune the sensor's responsiveness and accuracy, tailoring it for specific applications. The development of advanced fabrication techniques has led to enhancements in mechanical design, making these sensors not only more compact but also more reliable.
Electrical Signals
The output of a MEMS accelerometer is often in the form of electrical signals that represent the measured acceleration. These signals can be analog or digital, depending on the sensor design.
Crucial points regarding electrical signals include:
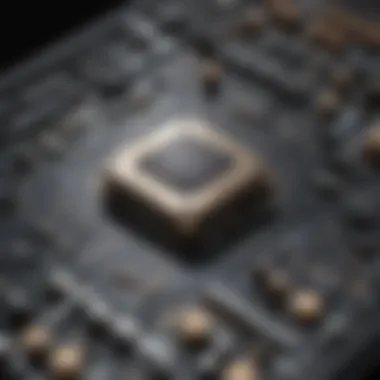
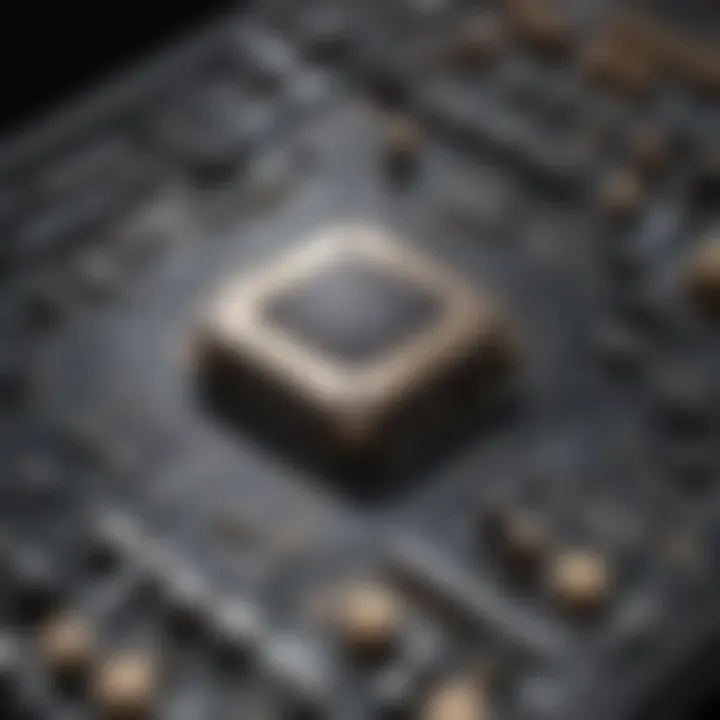
- Conversion: The mechanical movement of the seismic mass induces a change in capacitance or resistance, depending on the sensing method.
- Signal Conditioning: Post-processing of raw signals enhances accuracy, making them suitable for interpretation. This can involve filtering noise and amplifying the signal for better clarity.
Effective signal processing ensures that the data collected by MEMS accelerometers is reliable. This reliability becomes critical in applications like automotive safety systems, where quick and precise data are necessary for responsive measures.
Technical Specifications
The technical specifications of MEMS accelerometers are crucial for understanding their performance and applicability in different use cases. These specifications encompass a range of characteristics that define how the sensors operate and what advantages they offer in various environments. Key elements include sensitivity, range, resolution, and noise performance. These aspects are integral not only to the selection of a suitable sensor for a specific application but also in determining the sensor's compatibility with existing systems.
Sensitivity and Range
Sensitivity refers to the ability of a MEMS accelerometer to detect changes in acceleration. It is typically measured in milli-g per volt (mV/g) or similar units. Higher sensitivity allows for finer detail in the measurements, which is essential in applications such as gaming or gesture recognition. The range, on the other hand, defines the maximum acceleration the sensor can measure without saturating its output. MEMS accelerometers often come in various ranges, typically from ±2g to ±16g, which provides flexibility based on the application requirements.
For instance, in a smartphone, a lower range may suffice, but in automotive applications, higher ranges are needed to accommodate rapid changes in movement. When selecting a MEMS accelerometer, it is vital to consider both sensitivity and range to ensure the sensor can capture the intended measurements accurately and reliably.
Resolution and Noise Performance
Resolution pertains to the smallest change in acceleration that the accelerometer can detect, often measured as the least significant bit (LSB). A higher resolution indicates that the sensor can provide more detailed data, which is particularly significant in research settings or precise industrial applications. Furthermore, noise performance involves the ability of the sensor to minimize any unwanted signals that could distort the measurement. Noise can arise from various environmental factors and can lead to erroneous readings.
A good MEMS accelerometer will have a low noise density, meaning it can maintain accuracy over prolonged use. In applications like drone navigation, where precision is paramount, both resolution and noise performance play critical roles. Poor resolution or high noise levels can lead to substantial errors, impacting functionality and safety. Thus, evaluating these specifications is fundamental when deciding on the right MEMS accelerometer for specific needs.
"Sensitivity and range are essential parameters that determine the effectiveness of MEMS accelerometers in real-world applications."
The appropriate selection of these specifications ensures the successful integration of MEMS accelerometers into various technologies, enhancing their performance in diverse fields such as consumer electronics, automotive safety, and beyond. Understanding these technical specifications not only aids in developing better products but also opens avenues for innovations in sensor technology.
Fabrication Techniques
The fabrication of MEMS accelerometers is a critical component that significantly influences their performance and application suitability. Understanding the methods used in manufacturing these sensors provides insights into their design complexities and operational efficiencies. Advances in fabrication techniques not only improve the functionality of MEMS accelerometers but also reduce costs and enhance adaptability across various industries. The following sections detail key elements of silicon microfabrication and the importance of packaging and integration in the development of MEMS accelerometers.
Silicon Microfabrication
Silicon microfabrication is the foundational technique in the creation of MEMS accelerometers. This method involves an array of processes such as photolithography, etching, and deposition. Each step is designed to create intricate geometries at a micro and nano scale, which is vital for the sensor’s sensitivity and accuracy.
- Photolithography: This process transfers a pattern onto a silicon wafer to define the regions for etching. The precision of this initial step dictates the overall resolution and scale of the accelerometer's components.
- Etching: Both wet and dry etching techniques are used. Wet etching relies on chemical solutions to remove material, while dry etching employs plasma to achieve more intricate and cleaner structures.
- Deposition: Materials such as polysilicon and silicon dioxide are deposited to form the required layers. This is crucial for creating the mechanical and electrical properties needed for sensor functionality.
The advancements in silicon microfabrication lead to enhanced performance metrics including reduced power consumption and increased sensitivity. As techniques evolve, the opportunity for miniaturizing components further promotes the proliferation of MEMS accelerometers in novel applications.
Packaging and Integration
Packaging and integration are pivotal in transforming silicon microfabricated components into functional MEMS accelerometers. The packaging must protect the delicate internal components while ensuring efficient signal transfer and thermal management.
Considerations in packaging include the use of protective materials, mechanical support structures, and integration of electrical interfaces.
- Protection: Sensors must be shielded from environmental influences such as moisture and dust which can degrade performance.
- Thermal Management: Effective packaging aids in dissipating heat, which ensures consistent operation under varying conditions.
- Electrical Interfaces: Seamless integration of electrical connections is necessary for accurate signal transmission to processing units.
The integration of MEMS accelerometers into broader systems also involves designing compatible interfaces for communication with other electronic devices. This is especially significant in fields like consumer electronics and automotive systems, where interoperability and reliable performance are paramount.
"Effective packaging and integration is as important as the sensor's design itself, since they ensure longevity and consistent functionality in real-world applications."
By focusing on advanced fabrication techniques, manufacturers can produce MEMS accelerometers that are not only cost-effective but also perform reliably across diverse applications, thus enabling ongoing innovations in technology.
Applications of MEMS Accelerometers
MEMS accelerometers serve a crucial role in various fields, impacting both consumer applications and industrial systems. Understanding their applications helps to appreciate the versatility and utility of these sensors. This section discusses the significance of MEMS accelerometers across different domains, emphasizing their benefits and the considerations that accompany their use.
Consumer Electronics
MEMS accelerometers are the backbone of many consumer electronics today. They enable devices to detect motion, rotation, and orientation. For example, smartphones employ these sensors for features like screen rotation and gaming applications. The integration of MEMS accelerometers enhances user experience by providing seamless interaction. Additionally, wearables, like smartwatches and fitness bands, utilize these sensors to track movement and activity levels, which fosters health monitoring.
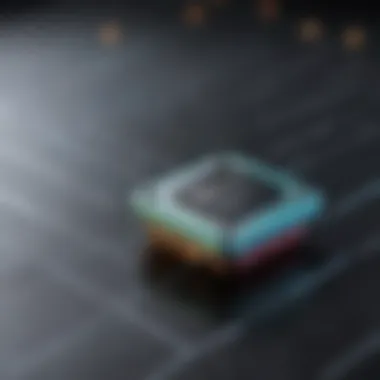
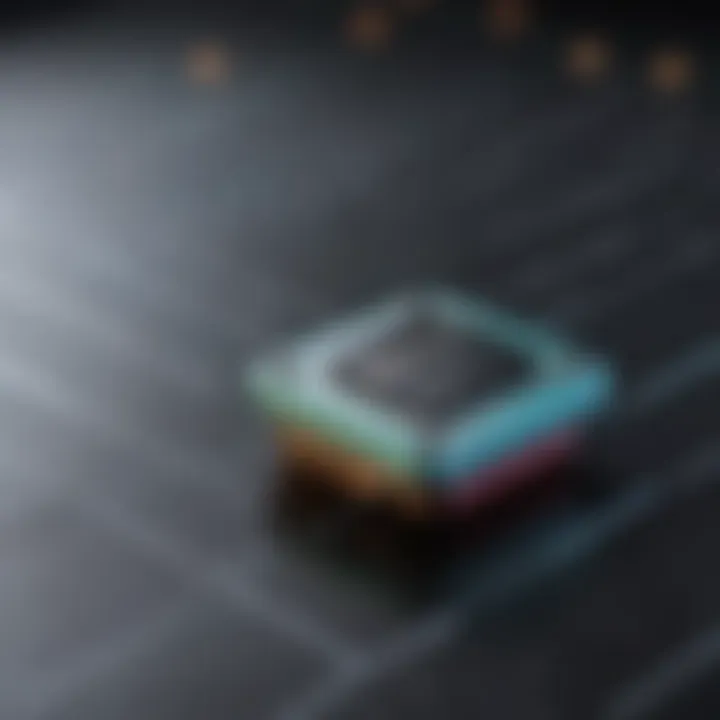
The small size and low power consumption make MEMS accelerometers ideal for compact devices. They provide precise measurements without adding significant weight or bulk. However, their performance can be influenced by environmental factors such as temperature and humidity.
Automotive Systems
In the automotive industry, MEMS accelerometers play a pivotal role in enhancing vehicle safety and performance. They are integral for various systems such as electronic stability control, airbag deployment, and anti-lock braking systems. By accurately sensing acceleration forces, these sensors can trigger automatic responses to maintain stability and prevent accidents.
Moreover, MEMS accelerometers assist in navigation and terrain sensing, providing valuable data for systems like GPS. This helps improve route accuracy and enhances driver awareness. While the benefits are substantial, it is essential to ensure proper calibration and reliability under different driving conditions.
Industrial Monitoring
MEMS accelerometers find applications in industrial monitoring as well. They facilitate the maintenance of equipment by detecting vibrations and anomalies, assisting in predictive maintenance. By continuously monitoring machinery, these sensors can signal when an operation is deviating from normal parameters.
This capability enables industries to minimize downtime and reduce repair costs. Additionally, MEMS accelerometers can be used in structural health monitoring, ensuring the safety of buildings and bridges by measuring vibrations and movements. However, their effectiveness can vary based on installation and environmental influences.
Health and Fitness Devices
In the realm of health and fitness, MEMS accelerometers are increasingly used in devices aimed at promoting wellness. They track physical activities, such as walking, running, and cycling. By analyzing the data collected by these sensors, users can gain insights into their daily habits, which can encourage active lifestyles.
These sensors contribute to advanced features in fitness devices, such as sleep tracking and workout analysis. The user-friendly data allows individuals to set and achieve fitness goals. Yet, it is necessary to consider the accuracy of data derived from these sensors, as various external factors can impact readings.
"The integration of MEMS accelerometers across different sectors highlights their adaptability and effectiveness in various applications."
In summary, MEMS accelerometers are a vital technology in consumer electronics, automotive systems, industrial monitoring, and health devices. Their compact size, low power usage, and versatile applications illustrate the importance of these sensors in modern technology.
Advantages of MEMS Technology
The advantages of MEMS technology play a crucial role in understanding the functionality and appeal of MEMS accelerometers. These benefits significantly enhance their usability in a wide range of applications. Just as MEMS devices have evolved, their advantages have continuously improved, positioning them as vital components in various industries.
Compact Size and Weight
One of the primary advantages of MEMS accelerometers is their compact design and light weight. These sensors can measure acceleration with remarkable precision while occupying minimal space. This feature benefits consumer electronics such as smartphones and tablets, where space constraints are of utmost importance.
The small form factor does not compromise their performance. Instead, it allows for easy integration into smaller devices, enabling innovations that were not feasible before. In automotive systems, for instance, the compact size allows for effective placement in various components without adding significant bulk or weight.
Additionally, a lighter sensor contributes to enhanced energy efficiency. For wearables like fitness trackers, lower weight means more comfort for users, increasing the likelihood of prolonged wear.
Cost-Effectiveness
The cost-effectiveness of MEMS accelerometers also stands out as a key advantage. As production methods mature and scale increases, the per-unit cost of MEMS devices has steadily declined. This has led to widespread adoption in applications that require multiple sensors, such as in smart home devices and industrial systems.
By reducing manufacturing costs, businesses can offer competitive pricing without sacrificing quality. This is particularly crucial in consumer electronics, where cost directly influences market share.
Moreover, MEMS technology has a relatively simple manufacturing process compared to traditional accelerometers. As a result, these devices can be produced more rapidly, which aids in meeting rising demand.
"The integration of MEMS accelerometers in everyday devices demonstrates the technology's efficiency and cost advantages, reshaping industries significantly."
Challenges in MEMS Accelerometer Development
MEMS accelerometers have revolutionized the way we measure acceleration and motion across various applications. However, their development is not without challenges. Understanding these hurdles is essential for improving the design and functionality of these sensors.
The first major challenge is environmental sensitivity. MEMS accelerometers are prone to changes in temperature, humidity, and other surrounding conditions. These variations can affect sensor performance and accuracy. For example, high temperatures might lead to thermal drift, which alters the output signal. In critical applications, such as safety systems in automobiles, it is vital to ensure that the accelerometer remains reliable regardless of environmental factors. Thus, manufacturers must focus on improving materials and designs that can tolerate a wider range of conditions without compromising accuracy.
Another significant issue is calibration problems. Precise calibration is crucial in making sure that the sensor's output matches the actual acceleration. However, MEMS sensors can shift over time due to mechanical stress or wear and tear. This change in performance may lead to inaccurate readings, which can be detrimental in applications such as navigation or structural health monitoring. Regular calibration tends to be time-consuming and costly, adding another layer of complexity to the development process. Researchers are exploring new solutions that can minimize the need for frequent recalibrations or develop self-calibrating systems.
"The accuracy and longevity of MEMS accelerometers hinge on overcoming environmental sensitivity and calibration challenges."
To summarize, the challenges faced in MEMS accelerometer development revolve around environmental sensitivity and calibration issues. Addressing these elements will be fundamental to the enhancement of sensor performance and reliability. Innovation in materials and design, alongside novel calibration techniques, could pave the way for better solutions in the upcoming years.
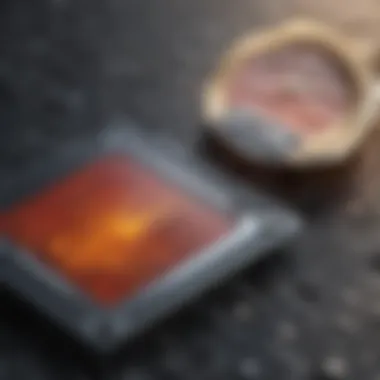
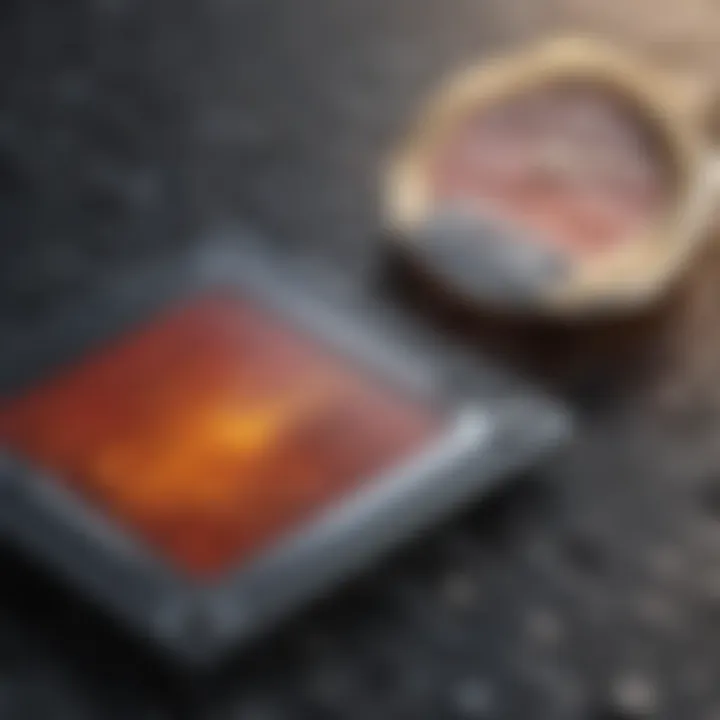
Recent Advancements
Recent advancements in MEMS accelerometer technology are pivotal in shaping the next generation of sensors. These innovations have enhanced the functionality and utility of MEMS accelerometers, making them vital components in various applications. Understanding these advancements is crucial for students, researchers, educators, and professionals working with these sensors.
Integration with IoT
The phenomenon of the Internet of Things (IoT) has brought a new perspective to the design and application of MEMS accelerometers. Integrating MEMS accelerometers with IoT provides several advantages.
- Real-time Monitoring: When connected to IoT systems, accelerometers can facilitate real-time data collection and analysis. This capability enhances proactive maintenance and operational efficiency in industries such as manufacturing and transportation.
- Data Accuracy: With the ability to transmit data wirelessly, MEMS accelerometers can ensure more accurate readings in various environments. Removing the need for traditional wiring decreases potential interference and ensures cleaner data streams.
However, integrating these sensors within IoT systems comes with unique challenges. Data validation and security become prominent topics. Ensuring that the data coming from accelerometers is reliable and secure is critical, especially in applications concerning safety and high-stakes environments.
Enhanced Performance Metrics
The recent developments in MEMS accelerometers have also led to enhanced performance metrics, which directly influence their applications across fields. Key enhancements include:
- Increased Sensitivity and Range: Current models offer higher sensitivity levels that allow for the detection of minute movements. This is beneficial in fields such as health monitoring. For instance, devices now can track subtle changes in activity levels accurately.
- Improved Resolution: Advancements in fabrication techniques have resulted in accelerometers that can produce more precise measurements. Such improvements lead to higher resolution data, which is essential for applications requiring fine detail, like aerial surveillance.
- Lower Noise Performance: Noise can significantly affect sensor accuracy. Recent designs have focused on minimizing noise interference, leading to clearer data output. This is especially important in industrial monitoring where precise measurements can drive decisions.
In summary, the advancements in MEMS accelerometers significantly contribute to their performance in integrated systems and increase their effectiveness across various applications. As technology evolves, these enhancements will likely continue to influence the development and deployment of accelerometer technology.
Future Trends
Exploring future trends in MEMS accelerometers reveals significant advancements that will shape the industry's landscape. These trends are vital not only for researchers but also for practitioners who apply these sensors in various fields. Understanding these developments helps stakeholders make informed decisions and enhances the practical applications of MEMS technology.
Miniaturization and New Materials
The drive for miniaturization in MEMS accelerometers is a response to the demand for smaller devices with enhanced functionalities. Smaller sensors mean less space occupied in electronics, allowing for sleeker designs in products like smartphones and wearables. Additionally, innovations in materials science are playing a crucial role. New materials such as graphene and advanced polymers are being researched for their superior properties. These materials can provide enhanced sensitivity and reduce noise performance, which is critical for accurate measurements.
The following points underscore the importance of this miniaturization trend:
- Space Efficiency: Smaller devices lead to more compact electronic designs.
- Energy Savings: Reduced size often correlates with lower energy consumption, promoting sustainability in technology.
- Enhanced Performance: New materials can lead to improved accuracy and functionality.
AI Integration
Artificial Intelligence (AI) is becoming increasingly significant in the realm of MEMS accelerometers. With more sophisticated data processing capabilities, AI can analyze sensor data in real-time, leading to improved decision-making processes. Incorporating AI enables predictive analytics, which can foresee potential failures or optimize performance based on environmental conditions.
Some key benefits of AI integration include:
- Enhanced Data Interpretation: AI algorithms can sift through vast amounts of data efficiently, identifying patterns that humans may miss.
- Real-time Monitoring: Instant analysis allows for immediate responses to changing conditions in applications such as automotive safety features or industrial process monitoring.
Integrating AI with MEMS accelerometers reflects a collaborative approach between hardware and software, producing systems that are more efficient and reliable. This synergy could lead to innovations that push the boundaries of what accelerometers can achieve in both consumer and industrial markets.
The convergence of miniaturization, new materials, and AI integration positions MEMS accelerometers at the forefront of technological advancement, driving progress across multiple domains.
Culmination
In concluding this exploration of MEMS accelerometers, it becomes clear that these sensors hold distinctive importance in various fields. The compact design and efficient functionality make them an essential element in modern technology. As highlighted, their role spans consumer electronics to automotive applications, showcasing their versatility and broad impact. The advantages of MEMS technology, particularly in terms of size and cost-effectiveness, further amplify their relevance in today’s market.
Summary of Insights
This article presents a comprehensive overview of MEMS accelerometers, detailing their fundamental principles, technical specifications, and diverse applications. Through the discussions in previous sections, several key insights emerge:
- Operational Principles: Understanding the basic mechanics behind these sensors enables better application in real-world situations.
- Technical Specifications: Features like sensitivity and resolution are critical to assess the performance of MEMS accelerometers.
- Applications: Real-world implementations, particularly in consumer devices and automotive systems, underline their significance.
These insights not only enhance our knowledge but also foster a deeper appreciation for the developments in the MEMS sector.
Implications for Future Research
The future of MEMS accelerometers looks promising, yet certain factors merit closer examination. Ongoing advancements such as integration with IoT systems call for new research strategies. Future studies can focus on:
- New Materials: Exploring innovative materials can lead to enhancements in performance metrics and sustainability for MEMS accelerometers.
- AI Integration: Investigating the application of artificial intelligence in sensor data processing could yield significant improvements in accuracy and reliability.
Overall, the exploration of MEMS accelerometers is not just about what they are today but also about where the technology is headed. Continued research in these areas will likely unlock new applications and optimize existing technologies.