The Evolution and Impact of Silicon Engineering
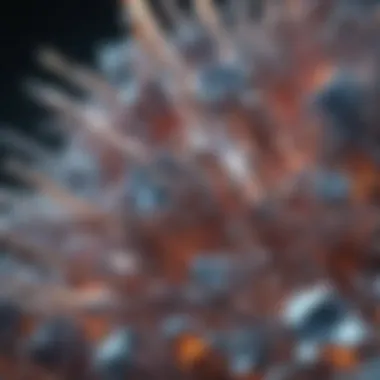
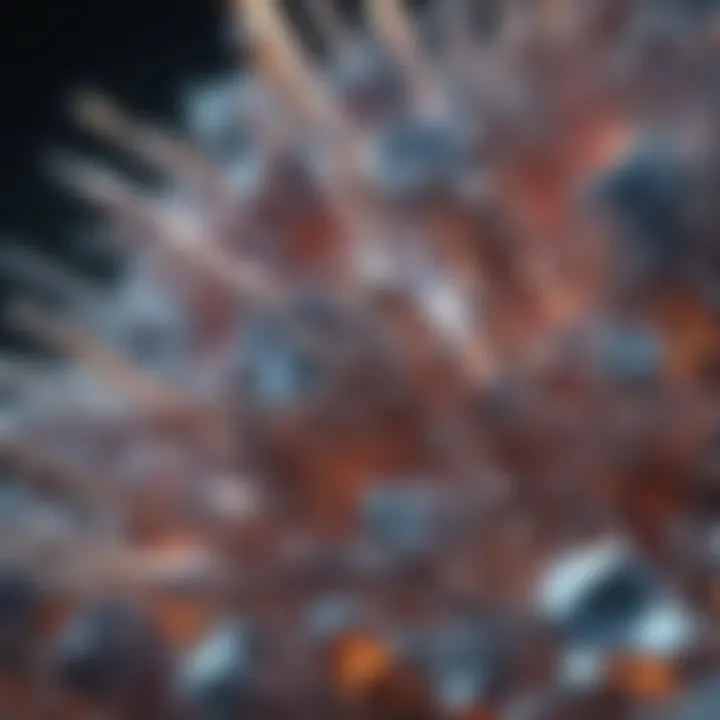
Intro
Silicon engineering represents not just a branch of scientific inquiry, but a fundamental aspect that shapes our technological reality today. This field's significance is interwoven with the very fabric of modern electronics, microprocessors, and energy solutions, making it an area rich for exploration and understanding. To appreciate the magnitude of silicon engineering, one must look beyond technical jargon and delve into both its practical applications and overarching principles.
From smart devices to renewable energy applications, silicon serves as the backbone of countless innovations. Yet, the path to modern silicon engineering is paved with challenges and breakthroughs, making its history just as riveting as its current state. Engaging with silicon engineering thus requires not just a technical background, but a consideration of its impact on society.
Research Background
Overview of the Scientific Problem Addressed
Silicon engineering is unique in that it forms the nexus between theoretical research and practical technology implementation. As researchers grapple with the limits of silicon-based materials, they often encounter a host of problems, such as scaling down devices while improving performance and energy efficiency. The questions surrounding the scalability of silicon components are critical, especially as the demand for faster and more efficient technology accelerates.
Historical Context and Previous Studies
The journey of silicon engineering actually began mid-20th century, with the initial leap into semiconductor technology. Scholars and industry pioneers focused their efforts on enhancing silicon's electrical properties, leading to developments like the bipolar junction transistor. Fast-forward to the 1970s, and the integrated circuit began to take hold, setting the stage for further innovations.
Over the years, numerous studies have explored various dimensions of silicon engineering. For instance, Mooreās Law, positing that the number of transistors on a microchip doubles approximately every two years, underscores the relentless quest to push silicon capabilities to their limits. Such studies not only highlight incremental technological advancement but also raise discussions about the sustainability of silicon-based solutions in a rapidly evolving tech landscape.
Barryās work on silicon photonics explored the use of light in data transfer, providing intriguing insights into future prospects. Moreover, recent studies have focused on alternative materials, questioning if silicon can remain the dominant player in varied applications. Much of this ongoing discourse is vital for academics, industries, and policy-makers interested in the future of technology.
Importance of Silicon in Current Technology
Understanding silicon's role in contemporary advancements gives clarity to existing challenges and solutions. Following are some significant areas highlighting silicon's relevance today:
- Microelectronics: Essential for creating smaller, faster, and more efficient devices.
- Renewable Energy: Silicon solar cells are at the forefront of solar technology, showcasing effective energy transference.
- Medical Devices: Innovations in implantable devices capitalize on siliconās biocompatibility.
"Silicon's reliability has propelled it to the forefront of technology, making it a magnet for researchers eager to leverage its potential for future innovations."
Findings and Discussion
Key Results of the Research
Recent findings point to an increasing interest in hybrid systems that integrate silicon with alternative materials, pushing the boundaries further. Additionally, considerable efforts are directed towards developing more efficient silicon-oxide layers for improved conductivity. This evolution signals that silicon, once thought of as a static entity, is undergoing a renaissance of sorts, enabling greater versatility in applications.
Interpretation of the Findings
As silicon technologies evolve, the implications stretch into various domains. Industries are keenly monitoring the material progress, and academia is enriching the discourse by exploring better design methodologies. The interplay among education, research, and industry has created an ecosystem ripe for innovationāa quintessential hallmark of modern silicon engineering.
In summary, the landscape of silicon engineering is not static but is continually responding to the challenges posed by a tech-savvy world. The understanding and mastery of silicon's properties offer a canvas for future generations of engineers and researchers alike.
The Foundation of Silicon Engineering
Silicon engineering is much more than just a specialized field within semiconductor physics; it is the backbone that supports a vast array of technological advancements in our world today. Understanding the foundation of silicon engineering offers insight into how siliconās unique properties have influenced technology from the conception of the first transistors to the complex integrated circuits we rely upon. Recognizing its importance helps grasp the intricacies of how silicon shapes, not only consumer electronics, but also sectors such as healthcare, renewable energy, and communication.
Historical Context
To truly appreciate the significance of silicon engineering, one must explore its historical journey. The transition from early vacuum tubes to solid-state devices in the mid-20th century marked a pivotal moment. Silicon found its footing in the 1950s as a semiconductor material, thanks to its abundance and suitable electronic properties. As stories go, the story of silicon is often intertwined with that of innovators like Jack Kilby and Robert Noyce, who paved the way for integrated circuits. It was a time when engineers were not just grappling with materials, but learning how to harness siliconās potential through careful experimentation and design.
One could say that silicon engineering was catalyzed by necessity; it filled a void by offering solutions that vacuum tubes could not, paving the path to miniaturization and improved efficiency. This shift laid the groundwork for the silicon revolution, placing silicon squarely at the heart of modern electronics.
Basic Principles of Silicon
At the crux of silicon engineering lies an understanding of the basic principles that govern silicon as a material. Silicon is a group IV element, encapsulated in the periodic table, symbolized as Si. What sets silicon apart from other elements is its ability to conduct electricityānot too much and not too little. This property makes it a semiconductor, meaning it can perform as an insulator, conductor, or a mix of both, depending on conditions.
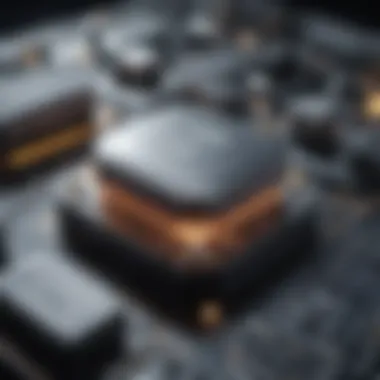
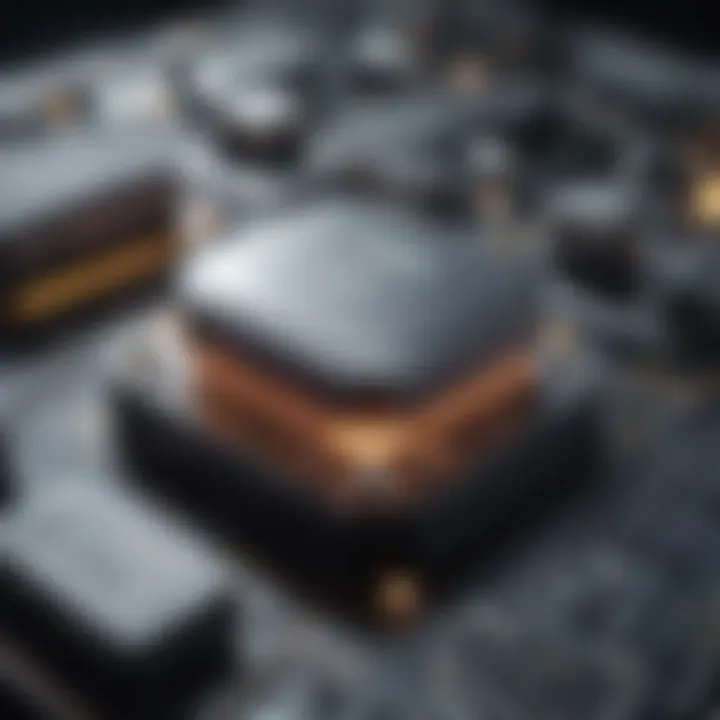
In this interplay of electrical properties, doping is a key mechanismāessentially the practice of introducing impurities into silicon to modify its conductivity. When elements such as phosphorus or boron are introduced, the silicon lattice is altered, either increasing or decreasing the flow of charge carriers.
Thus, understanding these principles allows engineers to design intricate electronic devices, from simple diodes to complex microprocessors.
Chemical Properties and Behavior
Diving into the chemical properties and behavior of silicon reveals why it has ascended to such heights in the realm of engineering. Silicon naturally bonds with oxygen, forming silicon dioxide (SiO2), a compound that plays a critical role in semiconductor fabrication. SiO2 serves as an insulating layer in various devices, thereby providing stability and preventing unwanted electrical interactions.
Additionally, silicon exhibits fascinating thermodynamic qualities. It retains heat effectively, allowing devices to operate within various temperature ranges, yet it can also be manipulated to behave differently under specific conditions. For instance, energy gaps can be precisely tailored during the doping process, enabling tunable electronic performances that are essential for todayās technological demands.
"The versatility of silicon is akin to clay in the hands of a potter; with the right techniques and knowledge, incredibly complex forms can emerge."
As we wrap our heads around these fundamentals, it becomes clear that the foundation of silicon engineering is not just about getting the mechanics right; it is about establishing a deeper relationship with a material that has become a cornerstone of innovation. The journey through siliconās past, principles, and inherent behaviors hints at the incredible potential it holds for future technological endeavors.
Silicon in Semiconductor Technology
Silicon plays a pivotal role in semiconductor technology, acting as the heart of numerous electronic devices that shape our modern world. From smartphones to computers, the impact of silicon is profound. Not only does it serve as a fundamental building block for integrated circuits, but its unique properties also allow it to efficiently conduct electrical current, enabling the miniaturization of components. This efficiency leads to faster, smaller devices with increased battery life, ultimately transforming consumer electronics.
The significance of silicon in semiconductor technology extends beyond mere functionality. The material's abundance and lower cost relative to alternatives make it a favored choice for manufacturers. Additionally, its thermal stability and flexibility in processing lend themselves to various applications, thus driving innovations in microelectronics. As we delve deeper into this realm, a closer examination of how silicon integrates with microelectronics will shed light on its critical aspects.
Role in Microelectronics
Silicon is the backbone of microelectronics. Its properties allow for the creation of transistors, the tiny on-off switches that govern electronic signals. When silicon is doped with other elements, like phosphorus or boron, it becomes a semiconductor, which is key for forming these transistors. This seemingly simple modification has cascaded into an explosion of modern technology. Without silicon, the limitations of microelectronic devices would be significant, resulting in larger, less energy-efficient systems.
The growing demand for compact electronic devices has led to an increasing reliance on silicon-based technology. Devices like smartphones heavily depend on silicon to support various functionalities in a tightly packed interior. As the trend towards smaller, faster gadgets continues, understanding silicon's role in enhancing microelectronics will be crucial in future developments.
Fabrication Techniques
Fabrication techniques are essential in translating siliconās potential into practical microelectronic applications. The methods involved are intricate and innovation-driven, each contributing to different aspects of silicon processing.
Photolithography
Photolithography is a key technique in silicon fabrication. In simple terms, it is a method used to transfer geometric shapes onto a silicon wafer. This is done using light to project patterns of a mask onto a photoresist application on the wafer. The ability to create microscopic patterns with high precision makes photolithography indispensable in the semiconductor industry.
- Key characteristic: High resolution and precise patterning.
- Unique feature: It can handle very small features, down to nanometer scales, essential for modern applications.
- Advantages: Enables mass production of devices at a lower cost compared to older methods, supporting high yields in semiconductor manufacturing.
- Disadvantages: As devices shrink, limitations on resolution and alignment can present challenges.
Doping Processes
Doping processes involve the introduction of impurities into silicon to alter its electrical properties, thus enhancing semiconductor function. This technique allows for control over electrical conductivity, making silicon ideal for various components.
- Key characteristic: Tailoring electrical properties by infusing specific doping agents.
- Unique feature: Doping can create regions of n-type (negative) and p-type (positive) silicon, essential for forming p-n junctions, a building block of many electronic devices.
- Advantages: Facilitates the production of more efficient and miniaturized electronic components.
- Disadvantages: The precision required in doping levels can complicate production processes.
Etching Methods
Etching methods play a crucial role in defining the structures on silicon wafers. During this process, unwanted material is removed to create intricate designs needed for microelectronics.
- Key characteristic: Removal of specific patterns with high precision.
- Unique feature: Etching can be done using chemical or plasma techniques, allowing versatility based on the desired outcome.
- Advantages: Essential for achieving the required miniaturization in complex circuits.
- Disadvantages: The etching process can introduce defects if not carefully monitored, leading to potential failure in the final product.
Advancements in Semiconductor Designs
The landscape of semiconductor designs is continuously evolving. Advances in silicon technology lead to smaller, faster, and more efficient devices. Innovations, such as the development of 3D chip structures and system-on-chip integrations, further exemplify the versatility and ongoing relevance of silicon.
Understanding these advancements, in conjunction with the techniques discussed, provides valuable insights into the inevitable evolution of technology and its dependencies on silicon semiconductor engineering.
Silicon Applications in Modern Devices
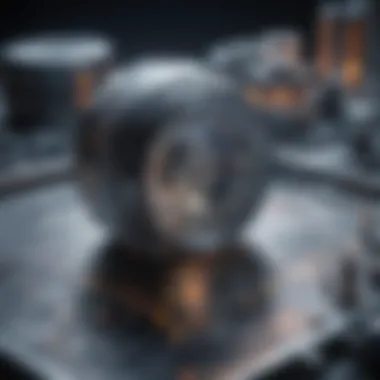
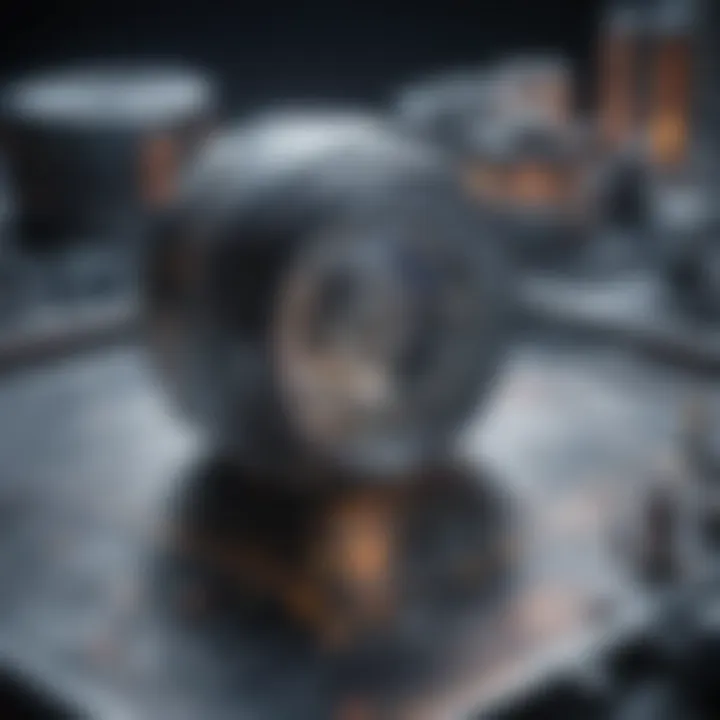
Silicon plays an integral role in our daily lives, functioning behind the scenes in myriad devices we often take for granted. As we explore silicon applications in modern devices, it becomes clear that the relevance of this material extends far beyond its use in semiconductor technology. Siliconās versatility and efficiency make it an essential component in developing various electronic and communication devices, demonstrating undeniable benefits in performance and sustainability.
Silicon-based Sensors
Silicon-based sensors are at the forefront of technological advancement. These sensors are used widely in everything from smartphones to industrial equipment. One major feature of silicon sensors is their ability to convert physical phenomena into electrical signals, enabling monitoring and analysis of environmental conditions.
These sensors offer a few noteworthy advantages, such as high sensitivity and the ability to withstand high temperatures, which is crucial for automotive and aerospace applications. The adaptability of silicon fabrication processes also means these sensors can be mass-produced, keeping costs down while meeting diverse industry needs. However, it's important to consider their limitations, including vulnerability to physical damage and the need for calibration under varying conditions.
Silicon Photovoltaics
Silicon photovoltaics represent a cornerstone in renewable energy technology. The primary advantage of silicon solar cells is their efficiency in converting sunlight into electricity. In contrast to other materials, silicon has a well-established production process and is readily available, making it a preferred choice for solar panel manufacturing.
Furthermore, advancements in silicon-based photovoltaic technology have led to the development of bifacial solar cells which can capture sunlight from both sides, enhancing energy output. On the downside, challenges remain in terms of the recycling of silicon panels and the environmental impact of manufacturing processes. Nonetheless, their contribution to reducing carbon footprints and promoting sustainable energy is significant and worthy of emphasis.
Silicon in Communication Technologies
Fiber Optics
Fiber optics has revolutionized the field of telecommunications, and silicon plays a pivotal role in enhancing this technology. Silicon photonic devices facilitate the integration of light-based mechanisms with traditional electronic circuitry. This integration allows for faster data transmission rates, which is essential in serving our ever-increasing demand for bandwidth.
The key characteristic of fiber optics is its ability to transmit data over long distances with minimal signal loss. This property is incredibly beneficial for internet and telecommunications infrastructures, as it reduces the need for signal boosters and repeaters, lowering overall costs. However, challenges such as the fragility of optical fibers and installation costs do exist.
"Silicon photonics represents a convergence of two critical technologies, promising advancements in communication bandwidth."
Signal Processors
Signal processors, particularly those based on silicon, are essential for interpreting data signals in various applications, from consumer electronics to complex industrial systems. They handle tasks like processing audio and video signals, which are indispensable in enhancing user experiences in devices we rely on daily.
A standout feature of silicon-based signal processors is their capability to scale. This allows manufacturers to develop processors that can cater to different performance levels, from low-power chips for mobile devices to high-performance units for servers. However, as silicon technology advances, ensuring signal integrity and managing power consumption becomes increasingly challenging.
To wrap it up, silicon applications in modern devices not only showcase the exceptional utility of this element but also demonstrate the continual strides we are making in technology. The landscape of silicon engineering is ever-evolving, offering rich terrain for future discoveries and applications.
Innovations Driven by Silicon Engineering
Silicon engineering is not just about creating microchips; it serves as a catalyst for diverse innovations that drive modern technology. The exploration of this field reveals transformative materials, methods, and concepts that shape our digital landscape. Understanding these innovations is crucial for industry professionals and researchers as they adapt and evolve to meet the challenges of tomorrow. In this section, we delve into two emerging materialsāgraphene and silicon carbideāand how they are reshaping the industry. We also highlight the integration of nanotechnology and the intriguing intersections with quantum computing, each pointing to a future rich with possibilities.
Emerging Materials
Graphene and its Variants
Graphene, a single layer of carbon atoms arranged in a two-dimensional honeycomb lattice, has taken the engineering world by storm. This material boasts an incredible combination of lightness, strength, and electrical conductivity, making it a standout contender in silicon engineering. The key characteristic that separates graphene from other materials is its extraordinary strengthāover 100 times that of steel, yet it is incredibly lightweight.
One of the crucial advantages of graphene is its flexibility and transparency, which opens doors to applications in flexible electronics and advanced optics. Recent studies have shown its potential in revolutionizing battery technologies, promising faster charging times and higher energy efficiency. However, itās not all smooth sailing. The scalability of graphene production remains a significant hurdle. Despite its promise, the manufacturing processes are often prohibitively expensive. This creates a landscape where its benefits must be weighed against practical constraints in production and application.
Silicon Carbide
Silicon carbide (SiC) is another remarkable material that has found its place in silicon engineering. Known for its high thermal conductivity and electrical resistivity, SiC plays a crucial role in power electronics, particularly in high-voltage and high-temperature environments. It excels in applications such as electric vehicles ( EVs) and renewable energy systems, where efficiency and thermal performance are vital.
A unique feature of silicon carbide is its ability to operate at temperatures exceeding 200 degrees Celsius without significant performance loss, a feat that silicon alone struggles with. This quality has deemed SiC indispensable for the development of next-generation power devices. However, as with graphene, challenges in manufacturing processes persist. The cost involved in synthesizing high-quality SiC crystals can be substantial, posing a barrier to wider adoption despite its benefits.
Integration with Nanotechnology
The intersection of silicon engineering and nanotechnology represents a frontier filled with potential. As devices shrink and demands for performance elevate, integrating nanotechnology into silicon engineering allows for more precise and miniaturized components. Applications are blossoming in areas such as drug delivery systems and ultra-sensitive sensors, thanks to nanoscale alterations in silicon structures. Research into nanoscale transistors has shown that they could drastically reduce power consumption while enhancing chip performance.
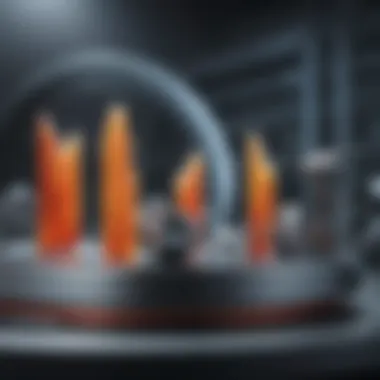
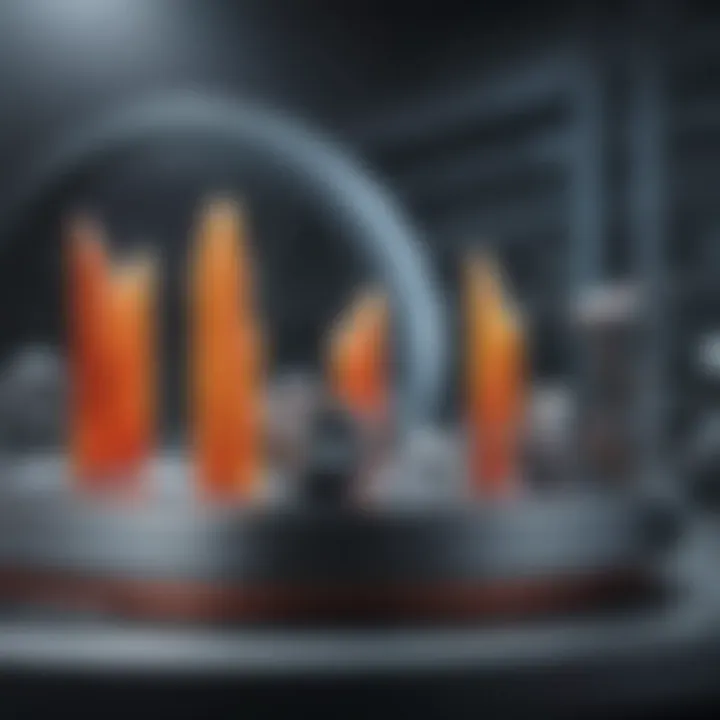
Nanotechnology doesnāt just push the limits; it redefines whatās achievable.
Quantum Computing and Silicon
Quantum computing stands as one of the most exciting fields intertwined with silicon engineering. Siliconās established framework in semiconductor technology makes it an appealing candidate for developing qubits, the building blocks of quantum computers. Researchers are experimenting with various methods to harness silicon's properties to create scalable quantum systems.
The promise of quantum computing lies in its ability to solve complex problems significantly faster than traditional computers. Potential applications include drug discovery, optimization problems, and cryptography. Nevertheless, the field faces challenges such as decoherence and the requirement for extremely low temperatures to maintain quantum states, which complicates practical implementation.
With innovations like integrated quantum circuits, the future of silicon in this realm looks promising, yet it requires extensive collaboration and research to overcome existing limitations.
Challenges and Considerations in Silicon Engineering
In the evolving world of engineering, particularly in the realm of silicon, the challenges and considerations stand as pivotal points for progress. These challenges are not merely hurdles but serve as catalysts for innovations that can change the landscape of technology and its application. Recognizing them is essential for students, researchers, educators, and professionals alike, simply because addressing these issues leads to sustainable development and broader economic growth.
Environmental Impacts
Silicon engineering, while at the forefront of technology, carries significant environmental concerns. The extraction of silicon, which occurs primarily through mining, poses threats to ecosystems. In areas where sand is harvested for silicon production, habitat destruction can spiral out of control. Moreover, during the manufacturing process, chemicals used can lead to waste that, if not managed properly, can cause pollutants to seep into the ground and waterways.
Consider, for instance, the energy consumption required in high-temperature processes, a key part of silicon chip creation. This energy often comes from non-renewable sources, compounding the carbon footprint of silicon manufacturing. To mitigate these impacts, thereās a push for more sustainable approaches, like utilizing renewable energy sources in production facilities and recycling old silicon-based products.
"Sustainable practices in silicon manufacturing not only help the environment but can also drive down costs in the long run."
Economic Factors
Silicon engineering and economics are inextricably linked. The demand for silicon-based technologiesāthink smartphones, computers, and solar panelsādrives the market. However, fluctuations in the global economy can impact prices and availability. The costs associated with silicon extraction, production, and purification are steep. As these costs rise, so too can the prices of consumer electronics, influencing purchasing behavior on a wide scale.
Moreover, regions heavy with silicon manufacturing often rely on this industry for their economic stability. An economic downturn, or significant changes in demand, can drastically affect local communities. Therefore, understanding the economic implications is critical. On the flip side, investments in research and development can stimulate job growth and technological advancements, thus challenging economists to find a balance between market supply and sustainable growth.
Technological Limitations
Despite its prominent role, silicon is not without its technological limitations. When seeking to push the boundaries of performanceāsuch as in the realms of quantum computing or advanced microprocessorsāsiliconās physical attributes can hinder progress. For instance, as devices shrink, silicon's electrical properties begin to degrade, leading to efficiency issues.
Developers and researchers are often faced with the daunting challenge of finding suitable alternatives or solutions to silicon's limitations. Some explore exotic materials, like gallium nitride or indium phosphide, which promise better performance at smaller scales but come with their own set of complications, such as cost and scalability.
In summary, although silicon engineering plays a vital role in the evolution of technology, it is not without its complications. Addressing environmental implications, navigating economic fluctuations, and overcoming technological constraints are essential for building a sustainable and innovative future. By tackling these issues, the engineering community can forge a path that not only advances silicon technologies but also respects the boundaries of our environment and economy.
The Future of Silicon Engineering
The future of silicon engineering holds a promise steeped in innovation and continuous transformation. As technology rapidly advances, silicon remains at the forefront, adapting to meet emergent demands across diverse sectors. This section paints a picture of where silicon engineering is headed, focusing on significant trends, collaborative efforts, and its vital role within a broader innovation ecosystem.
Trends to Watch
A look at trends shaping the future of silicon engineering reveals several pivotal shifts. For instance, miniaturization remains a dominant force. As devices become smaller, the quest for efficient, compact components continues. Additionally, thereās growing interest in enhancing energy efficiency, particularly with the rise of renewable energy technologies. Silicon-based materials, alongside silicon carbide, hold potential for better performance in energy applications.
Here are some key trends to keep an eye on:
- Flexible Electronics: More devices are moving towards flexibility, enabling new applications in wearables and adaptable displays.
- Advanced Packaging: Techniques such as chip stacking are on the rise, pushing boundaries on space and thermal management.
- Integration with AI: The interplay between silicon engineering and artificial intelligence is making chips smarter and more capable of processing vast amounts of data instantly.
"The continuing evolution in silicon design parallels advancements in our understanding of materials science and engineering, offering exciting possibilities for the next generation of technology."
Interdisciplinary Collaborations
Moving forward requires breaking down silos. Collaborations across disciplines are becoming essential in silicon engineering. For example, partnerships between material scientists, electrical engineers, and computer specialists are enabling breakthroughs that wouldn't emerge in isolated environments. In such collaborations, knowledge sharing and skill mixing facilitate innovative problem-solving approaches.
Additionally, efforts linking silicon technology with biomedical sectors have sparked interest in bioelectronic devices. These devices can monitor health and convey data in real time, positioning silicon as a crucial asset in personalized medicine. Just as importantly, collaboration with environmental scientists focuses on developing sustainable practices in silicon production, addressing eco-friendly materials and methods for a greener future.
Silicon Engineering in an Innovation Ecosystem
Silicon engineering does not operate in a vacuum; it is part of a complex innovation ecosystem. This landscape encompasses various industries, academia, and research entities that collectively drive progress. For instance, partnerships between startups and established firms often yield cutting-edge applications, enabling quick pivots to market needs. In addition, regulatory bodies and educational institutions also contribute to this ecosystem by shaping standards and training the next generation of engineers.
Moreover, the ongoing digital transformation across all sectors signals greater demand for silicon-based solutions. Whether thatās in cloud computing, the Internet of Things, or smart cities, silicon engineering is set to play a significant role in not just enhancing current offerings but also in creating future possibilities.