Fiber Photometry in Neuroscience: Key Advances and Uses
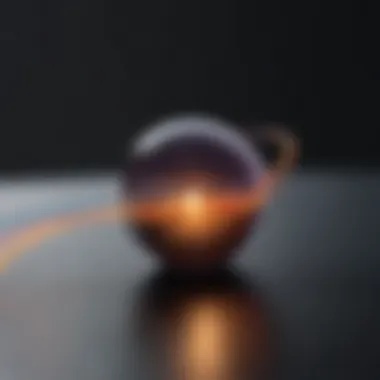
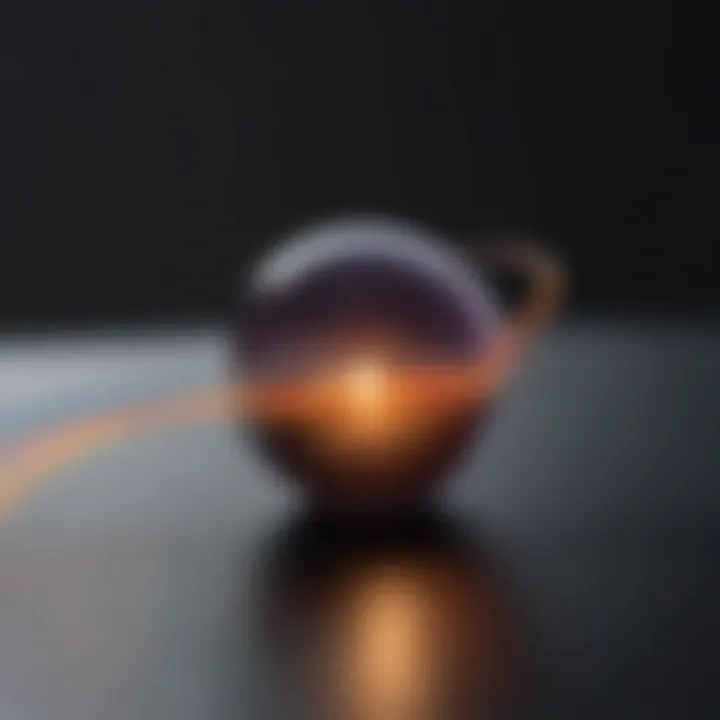
Intro
In recent years, the evolution of technology within neuroscience has spurred groundbreaking methods for observing and understanding the intricacies of neural activity. One such method is fiber photometry, a technique that allows scientists to monitor neuronal behavior in real time with minimal invasiveness. In a field where precision and clarity are critical, fiber photometry stands out by bridging the divide between traditional electrical recording techniques and optical methodologies. This article endeavors to guide you through the deep waters of fiber photometry, showcasing its principles, breakthroughs, and implications for behavioral neuroscience.
To help situate our discussion, this article will first explore the
Research Background
Overview of the Scientific Problem Addressed
Understanding neural circuits and their corresponding behaviors is a complex challenge in neuroscience. Traditional methods, such as electrophysiology, have limitations in terms of spatial resolution and the invasiveness of procedures. As scientists seek to gather more intricate workings of the brain, the demand has grown for approaches that provide clarity without the associated drawbacks of conventional methods. Fiber photometry meets this need by offering a way to observe thousands of neurons simultaneously, providing richer datasets for analysis and interpretations.
Historical Context and Previous Studies
Fiber photometry's roots can be traced back to early optical imaging technologies, which have been evolving since the late 20th century. Initial studies focused mainly on using fluorescence imaging techniques to capture neuronal activity. The introduction of genetically encoded calcium indicators transformed the landscape by allowing researchers to visualize internal cellular events. It wasn't until the advent of fiber optic technology that a more practical, less invasive method emerged.
Key studies in the early 2000s began to lay the foundation for fiber photometry, demonstrating its potential in animal models. As engineers and neuroscientists collaborated, various adaptations of the technique came to light, including miniaturized fiber optic devices and advances in fluorescence detection methods. All of these discoveries contributing to a robust framework for future research, emphasizing the potential of fiber photometry in unraveling complex neural processes.
Findings and Discussion
Key Results of the Research
The influence of fiber photometry on neuroscience research has been tangible. Studies have demonstrated its capability to track calcium signals in neurons during various behavioral tasks. Results have shown a clear link between specific neuronal activities and behavioral outcomes, deepening our understanding of how we process information.
Interpretation of the Findings
Importantly, this methodology does not operate in a vacuum. Instead, it interacts dynamically with other research techniques, creating a more holistic picture of neural operations. The ability to gather large amounts of real-time data across various conditions has proven invaluable, allowing for new revelations about neurotransmitter dynamics, synaptic activity, and their relationship with observable behavior.
"Fiber photometry is not merely a tool; it’s a lens through which the complexity of neuronal behavior can be studied and understood, paving the way for innovative research and therapeutic approaches."
Preamble to Fiber Photometry
Fiber photometry stands as a pivotal innovation within the realm of neuroscience, granting researchers the capacity to scrutinize neural activity as it unfolds, all while maintaining minimal encroachment on the subjects under study. This area of inquiry delivers insights that traditional methods might overlook or distort due to their invasive nature. The shift towards using optical techniques has offered an upgrade in understanding complex behaviors, neural dynamics, and pathophysiological conditions.
This section will uncover the historical background of fiber photometry, elucidate its foundational techniques, and highlight the advantages it presents over more conventional methods. Being able to evaluate real-time changes in neural signaling not only enhances our grasp of fundamental brain functions but also significantly aids in deciphering the underpinnings of various neurological disorders. Understanding the fundamentals behind fiber photometry is imperative, as it forms the bedrock for its applications in behavioral analysis and therapeutic advancements.
Historical Context
The origins of fiber photometry trace back several decades, evolving alongside advancements in optical imaging and neurobiology. Initially, traditional electrophysiological techniques dominated the stage for exploring neural circuits and behaviors. However, these approaches often fell short—exhibiting limitations in temporal resolution and spatial accuracy. As the field of neuroscience advanced, new methodologies emerged, with fiber photometry breaking into the spotlight in the early 2000s. The integration of optical fibers within neural studies offered a novel perspective that was previously unimaginable.
Researches began adapting optical imaging techniques originally used in other fields, such as telecommunications, harnessing the precision of light to investigate neuronal activity without overly invasive procedures. As technology progressed, the miniaturization of components enabled more extensive applications, paving the way for a deeper exploration of the brain’s complexity.
Fundamental Techniques
Basic principles of optical imaging
At the heart of fiber photometry lies the essential concept of optical imaging, which revolves around the use of light to capture the dynamics of fluorescent signals emitted by neural indicators, like calcium sensors. These sensors react to various changes in neural environments, illuminating ebb and flow of calcium ions that correlate with neuronal firing patterns. The unique characteristic of optical imaging in this context is its ability to provide real-time data with high temporal resolution.
This method holds distinct advantages, such as the non-invasive approach which permits longitudinal studies on the same subjects, enhancing the reliability of findings. However, the effectiveness of optical imaging can be impacted by factors like light scattering and absorption within biological tissues, which can sometimes complicate data interpretation.
Comparison with traditional methods
When placed side-by-side with traditional electrophysiological techniques, fiber photometry showcases significant strengths and challenges. Unlike standard electrode-based methods, which often provide high spatial resolution at the cost of invasiveness, fiber photometry bridges a gap by ensuring minimal tissue damage while still capturing broad regions of neural activity.
What sets fiber photometry apart is its unique capability to monitor large populations of neurons simultaneously, enabling a holistic view of neural circuits influencing behavior. It also benefits from the ability to correlate optical signals with a variety of physiological states, illuminating the dynamic nature of neural communications. Yet, it is not without its drawbacks; issues surrounding the depth of light penetration in brain tissue pose challenges that are still being addressed through ongoing innovations.
In light of these perspectives, fiber photometry has established itself not merely as a technical advancement but as a derived necessity in the pursuit of deepening our understanding of the brain’s workings. As we proceed, understanding these advancements is not just academic; it is integral to the future of behavioral neuroscience.
Technical Components of Fiber Photometry
Fiber photometry stands at the intersection of biology and technology, an essential development that enables neuroscientists to peer deeper into the workings of the brain. The technical components are instrumental to the efficacy and accuracy of the techniques used. When discussing the advancements and applications of fiber photometry, it is crucial to consider specific elements including optical fibers, light sources, and detection systems, as they significantly influence the quality and reliability of the data obtained.
Optical Fibers
Types of optical fibers used
The variety of optical fibers used in fiber photometry greatly impacts experimental outcomes. Generally, two primary types are utilized: silica-based fibers and polymer optical fibers. Silica fibers are widely acknowledged for their durability and lower attenuation losses, allowing signals to travel longer distances without degradation. On the other hand, polymer fibers are thinner and more flexible, making them ideal for delicate surgeries where ease of manipulation is paramount.
Yet, silica fibers’ robustness might prove advantageous when studying dense neural environments that demand resilience against physical disturbances. Ultimately, the choice between silica and polymer fibers can heavily sway experimental results, with investigators weighing factors like flexibility versus signal quality.
Manufacturing processes
The process of manufacturing optical fibers plays a pivotal role in ensuring that they meet the specific requirements of various applications in neuroscience. The fabrication method often includes drawing and coating. Drawing involves heating a preform and pulling it into thin strands, while coating protects the fiber from environmental factors and enhances light transmission efficiency. Such methodologies lead to fibers that can effectively handle light transport and resist degradation over time. Although advanced manufacturing can yield superior fibers, controlling production costs remains a pressing concern for many labs. The delicate balance between quality and affordability often dictates the types of fibers chosen for experiments.
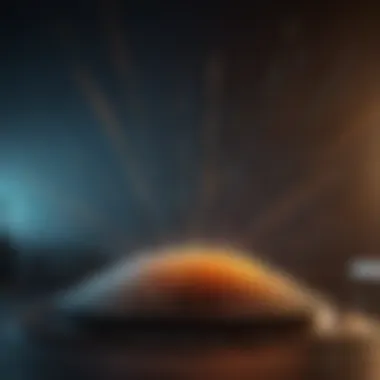
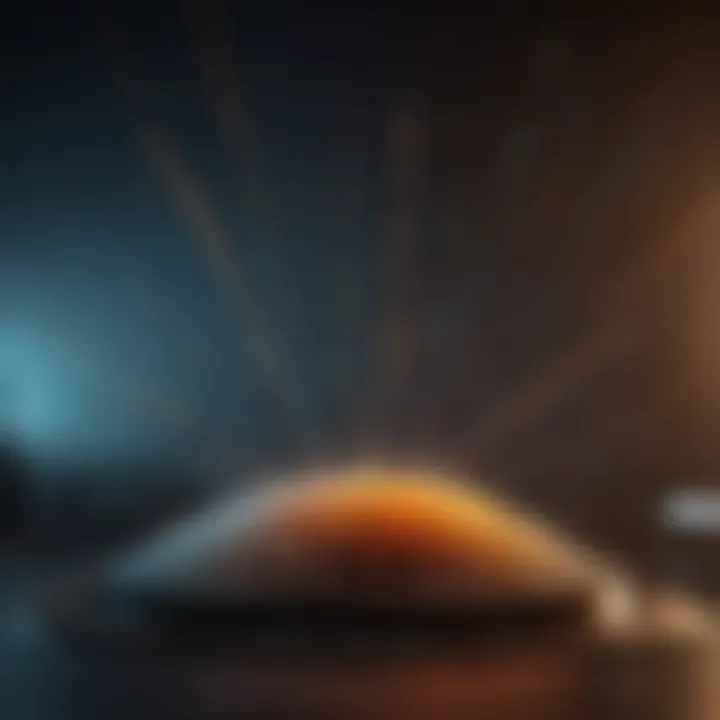
Light Sources
LED versus laser systems
The choice between LED and laser light sources is critical for achieving optimal results in fiber photometry. LEDs, or light-emitting diodes, are favored for their lower costs and longer lifespan. Their flexibility in terms of size and ease of integration make them a popular option, especially for setups requiring multiple light inputs. Conversely, laser systems provide greater light intensity and coherence, enabling researchers to achieve higher resolutions over longer distances.
The trade-offs between LEDs and lasers often revolve around specific experimental needs. For instance, high-resolution imaging might necessitate lasers despite their higher cost, while budget-dependent projects might opt for LEDs.
Wavelength selection
Wavelength selection is another vital aspect that significantly affects experimental outcomes. Specific wavelengths are often more effective for eliciting responses in particular fluorescent indicators or proteins. Researchers usually select wavelengths based on the absorption characteristics of the fluorescent molecules they are observing.
For example, if calcium indicators are being used, one might aim for wavelengths around 488 nm where fluorescence efficiency is maximized. This judicious selection can ultimately determine the sensitivity and accuracy of neural measurements, impacting the overall objectives of the study.
Detection Systems
Photodetectors and sensors
The choice of photodetectors and sensors is paramount in capturing light signals generated during fiber photometry. Photomultiplier tubes (PMTs) and charged-coupled devices (CCDs) are commonly employed due to their high sensitivity and quick response times. PMTs excel in detecting very weak signals, leading researchers to favor them when working with noisy environments. On the other hand, CCDs tend to be more versatile and easier to integrate into various systems.
Both types have their unique strengths, with PMTs often leading in sensitivity and CCDs offering flexibility, allowing scientists to choose based on specific study requirements and budget constraints.
Signal processing techniques
Finally, signal processing techniques play a critical role in interpreting the data obtained from fiber photometry. Effective processing involves noise reduction and signal enhancement, essentially translating raw data into meaningful insights about neural activity. Techniques like Fourier transforms or machine learning algorithms can be employed to enhance signal clarity. Each technique comes with its own pros and cons; while Fourier analysis is useful for frequency domain insights, machine learning algorithms can significantly improve prediction accuracy but may require substantial datasets. Thus, the process of signal interpretation becomes as much about expertise as it is about technique, ultimately guiding researchers toward valid conclusions in their investigations.
Applications in Neuroscience
The impact of fiber photometry in neuroscience cannot be overstated. This technique breathes new life into the traditional approaches of studying neural activity by offering a real-time, minimally invasive look at brain function. Its unique capacity to measure fluorescent signals in living organisms allows researchers to unravel the intricate web of neural circuits, paving the way for profound insights into various neurological phenomena.
Real-Time Monitoring of Neural Activity
Calcium Imaging
Calcium imaging represents a cornerstone of real-time monitoring in neuroscience research. This technique relies on the principle that neural activity is closely tied to calcium ion concentrations within neurons. When neurons fire, there is a spike in calcium levels that fluorescing indicators can visualize. The ability to track this calcium signal offers a window into the dynamic processes of neuronal firing patterns.
The key characteristic of calcium imaging is its capability to capture rapid changes in neuronal activity, which is crucial in understanding how neural networks operate in real-time. Its positional advantage lies in providing insights that traditional methods like electrophysiology may miss by focusing on temporal resolution over spatial accuracy. Notably, calcium imaging also allows for the examination of multiple neurons simultaneously.
However, calcium imaging isn't without its challenges. While the technique does provide an abundance of data, interpreting the signals can be tricky; the relationship between calcium influx and actual firing rates is not a straightforward one. Thus, researchers must tread carefully when making inferences about neural activity.
Optogenetic Applications
Optogenetics introduces a fascinating aspect to the study of neural activity. By embedding light-sensitive proteins into neurons, researchers can precisely control neuronal firing with light. This specificity elevates optogenetics to a level where one can selectively activate or inhibit neurons, facilitating a deeper understanding of their functions in behavioral contexts.
The hallmark of optogenetics is its unrivaled precision. This method goes beyond merely observing activity – it allows for the direct manipulation of neural circuits. Consequently, it can illuminate the specifics of causal relationships between neural activity and behavioral outputs. It’s become a popular choice among neuroscientists for designing experiments related to behavior and cognition.
Despite its strengths, optogenetics does have some drawbacks. The need for genetic manipulation to introduce light-sensitive proteins might limit its applicability to specific model organisms or conditions. Moreover, the requirement of specialized light sources for activation can impose significant technical constraints.
Behavioral Studies
Linking Behavior to Neural Activity
Linking behavior to neural activity is critical in addressing questions about the neural basis of cognition and behavior. Fiber photometry opens pathways to directly correlate behavioral changes with firing patterns in specific neural circuits. In this context, researchers can observe how variations in neural activity translate to behavioral responses, elucidating the complex interplay of brain and behavior.
The distinctive trait of this approach is its ability to create a clearer picture of neuronal function in the context of behavioral data. This makes it an invaluable tool, especially for studying phenomena like learning, memory, and decision-making. Understanding how behavior can be modulated by specific neural signals provides an avenue to delve into foundational principles in neuroscience.
However, challenges exist in establishing these links. Variability in behavioral responses can complicate the interpretation of data, and ensuring proper control in experiments is essential. On the flip side, these studies often require extensive cross-disciplinary collaboration, further enriching the overall understanding of both behavior and neural activity.
Circuit-Level Investigations
Examining neural circuits at a circuit-level is fundamental for an in-depth understanding of brain function. Fiber photometry allows simultaneous monitoring of the activity of multiple neurons within a microcircuit, unveiling a plethora of interactions that form the underpinnings of cognition and action. This analytical focus on circuit dynamics can reveal how neural populations coordinate in response to various stimuli.
The key benefit of circuit-level investigations is their ability to highlight integrative functions within the brain, rather than viewing neural activity in isolation. This holistic perspective is vital, especially in studies related to sensory processing and motor control, where collaborative activity among neurons is crucial. Moreover, this method facilitates creating a narrative around how neural pathways influence behavior.
Despite its promise, circuit-level investigations can be methodologically challenging due to the complexity of brain networks. Furthermore, drawing generalized conclusions about broader brain function from localized observations can sometimes be misleading, making careful interpretation essential.
Pathophysiological Research
Studying Neurological Disorders
The role of fiber photometry extends beyond basic research; it pinpoints insights crucial for understanding neurological disorders. Utilizing this method to observe real-time fluctuations in neuronal activity can illuminate the pathophysiology of conditions such as epilepsy, anxiety, and depression. Furthermore, it allows researchers to dissect how dysregulation of neural circuits contributes to specific clinical symptoms.
A standout aspect of studying neurological disorders with fiber photometry is its capacity to monitor activity over lengthy time frames, providing data on how neural circuits evolve during pathology. This can potentially lead to a better understanding of disease progression and the identification of biomarkers for early detection.
Nevertheless, researchers must navigate complexities when generalizing findings from animal models to human conditions. Additionally, establishing causation rather than mere correlation remains a significant concern in these studies.
Potential for Therapeutic Insights
The therapeutic implications of fiber photometry in neuroscience research are substantial. Through detailed observation of how various treatments – be it pharmacological, behavioral, or neuromodulatory – impact neural activity, researchers can glean insights that may lead to innovative interventions. This offers not just observational power, but actionable insights.
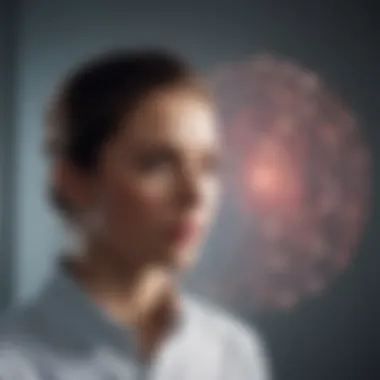
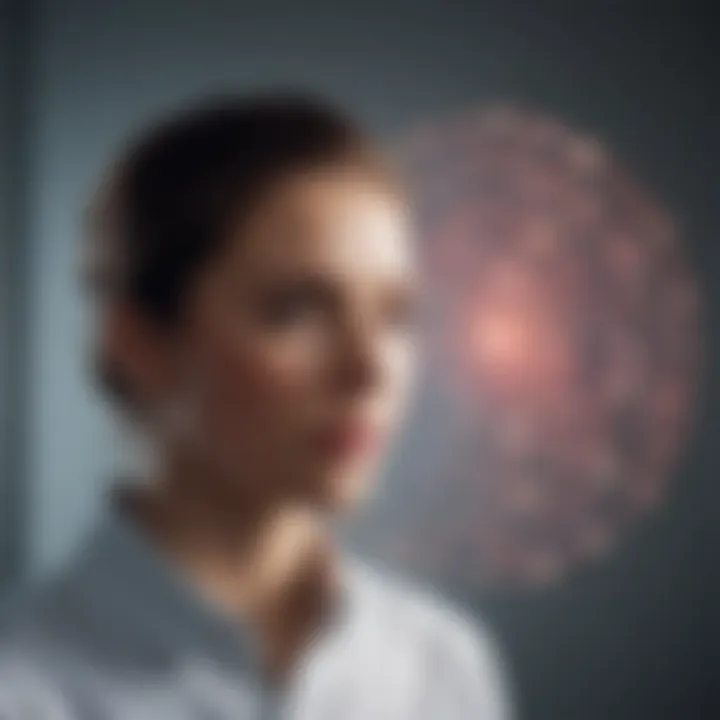
Here, the unique selling point lies not only in efficacy assessments but also in understanding underlying mechanisms of action. For instance, therapies targeting specific circuit dysfunctions can be tested through real-time activity monitoring, paving the way for precision medicine approaches in treatment.
The downside, however, is the inherent challenge in translating findings from animal models to human applications. As with studying neurological disorders, discrepancies in neurobiology between species can complicate the therapeutic landscape, necessitating a cautious approach while considering human applications.
"Fiber photometry not only illuminates the mysteries of neural behavior, but also brightens the path towards therapeutic advancements in treating neurological disorders."
In summary, fiber photometry's applications in neuroscience provide a powerful lens through which researchers can probe neural mechanisms and their implications for behavior and pathology. As technology continues to voyage forward, the prospects for both understanding and intervention become even more promising.
Methodological Considerations
The realm of fiber photometry isn't just about sophisticated equipment and flashy results; it's rooted deeply in the methodology underpinning experiments and their outcomes. Understanding methodological considerations is crucial for researchers focused on how and why neural mechanisms work. This section dives into vital elements that affect the success of fiber photometry, including experimental design, data interpretation, and the integration with other techniques. These facets enrich the experimental framework and allow for more nuanced findings.
Experimental Design
Choosing the right parameters
At the heart of any experiment lies the selection of appropriate parameters. In fiber photometry, this step is fundamental. The right parameters dictate the quality and depth of the data collected. A key characteristic is that the choice of wavelength and excitation sources can significantly influence the resolution of neural signals. Selecting parameters tailored to specific biological questions not only improves signal clarity but can also enhance the overall granularity of the data.
In practical terms, this means optimizing the light intensity to avoid bleaching while ensuring ample signal detection. The unique feature here is the balance researchers must strike; too much light can drown out critical signals, while too little may lead to insufficient data. It's a balancing act that requires careful consideration, making this choice both beneficial and often tricky.
Data collection protocols
Data collection protocols in fiber photometry require meticulous attention to detail. These protocols guide how information is gathered under varying conditions. Highlighting uniformity and reliability, they serve as the backbone for reproducibility in research. By adhering to established data collection protocols, researchers can ensure consistency across multiple trials, leading to more credible comparative analyses.
One unique aspect of data collection protocols in fiber photometry is the use of real-time data logging, which captures dynamic changes in neural activity. This feature provides invaluable insights during experiments. However, it also presents challenges, as the sheer volume of data can become cumbersome to analyze. Ultimately, while robust data collection protocols enhance the integrity of findings, they do demand a significant commitment in analysis time.
Data Interpretation
Quantification of signals
The quantification of signals is where art meets science in fiber photometry. Translating raw signal data into meaningful metrics allows researchers to make informed conclusions about neural dynamics. A standout characteristic of this process is the application of mathematical models and algorithms to distill large datasets into comprehensible patterns. This not only helps in framing hypotheses but also supports the development of predictive models about neural activities.
However, the unique feature of quantifying signals often lies in the variability inherent to biological systems. While quantification is crucial for drawing conclusions, interpreting these numbers requires a deep understanding of the biological context. This aspect serves as both a benefit and a drawback; it opens the door to new discoveries but also introduces room for misinterpretation.
Challenges of ambiguity
In the world of data interpretation, challenges of ambiguity loom large. This aspect entails unraveling complex neural signals, which frequently overlap or exhibit noise. A key characteristic here is the difficulty faced when attempting to interpret unclear signals. This makes drawing unequivocal conclusions challenging and adds a layer of complexity to the research.
The standout feature related to this is the interplay between noise and genuine signals. Isolating true neural activity from extraneous factors can be an uphill battle. While addressing these challenges often introduces more robust analytical frameworks, it can also lead to potential misrepresentations if not managed judiciously.
Integration with Other Techniques
Combining with electrophysiology
The integration of fiber photometry with electrophysiology stands as a significant advancement in neuroscience research. This approach allows researchers to harness the strengths of these complementary techniques. Combining electrophysiological readings with optical data can paint a richer picture of neural function. A fundamental aspect of this synergy is that it provides simultaneous monitoring of electrical and optical signals, giving researchers a thorough understanding of both the dynamics and location of neural activity.
Moreover, the unique strength lies in the enhanced resolution of temporal data that electrophysiology offers. However, tapping into this potential also comes with its challenges; coordinating these two techniques requires specialized training and knowledge to navigate their intricate operations successfully.
Synergistic approaches
Shifting towards synergistic approaches emphasizes the collaborative nature of modern neuroscience. This perspective encourages researchers to employ multiple methodologies to address complex questions about brain function. The key characteristic here is the ability to triangulate findings across diverse platforms—filing detailed insights from various angles.
What makes this fascinating is that synergistic approaches foster innovation. Researchers can uncover insights that may not have been visible through single-method studies. Yet, balancing different methodologies can introduce logistical hurdles, including resource allocation and training requirements. While the advantages highlight a richer comprehension of neural mechanisms, the potential drawbacks necessitate careful planning and execution in experimental settings.
Limitations and Challenges
The discussion of limitations and challenges in fiber photometry is crucial in comprehending its role and efficacy in neuroscience. While the technology represents a leap forward in observing neural activity, it comes with certain constraints that researchers must navigate. Recognizing these obstacles allows for a more informed application of fiber photometry and encourages innovations that may address them.
Technical Limitations
Spatial resolution concerns
One major aspect is the spatial resolution concerns. This is all about how precisely we can pinpoint where in the brain the signal is coming from. Fiber photometry often sacrifices higher spatial resolution for the sake of being less invasive and more accessible. The key characteristic here is that, while fiber optics can gather signals from a wide area, they aren't particularly suited to distinguishing activities from neighboring neurons. In many cases, this broad coverage can blur the lines of our understanding.
This limitation is a popular topic because it directly affects the accuracy of the data gathered. For example, if multiple neurons are firing in close proximity, the signal can become muddled. A unique aspect of spatial resolution issues is that it necessitates a careful balancing act: researchers must consider whether they need detailed images of activity or if a broader overview will suffice.
The disadvantage of this lack of precision means that conclusions drawn from data could sometimes lead to misconceptions about the actual neuronal events occurring.
Signal-to-noise ratio issues
Signal-to-noise ratio issues also present significant challenges. This refers to the ability to differentiate the actual neural signals from other background noise that can interfere with the readings. A strong signal-to-noise ratio is essential for reliable data collection. High noise levels can arise from various sources, including thermal fluctuations and fluctuations in light sources.
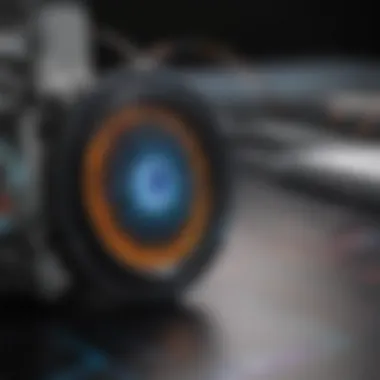
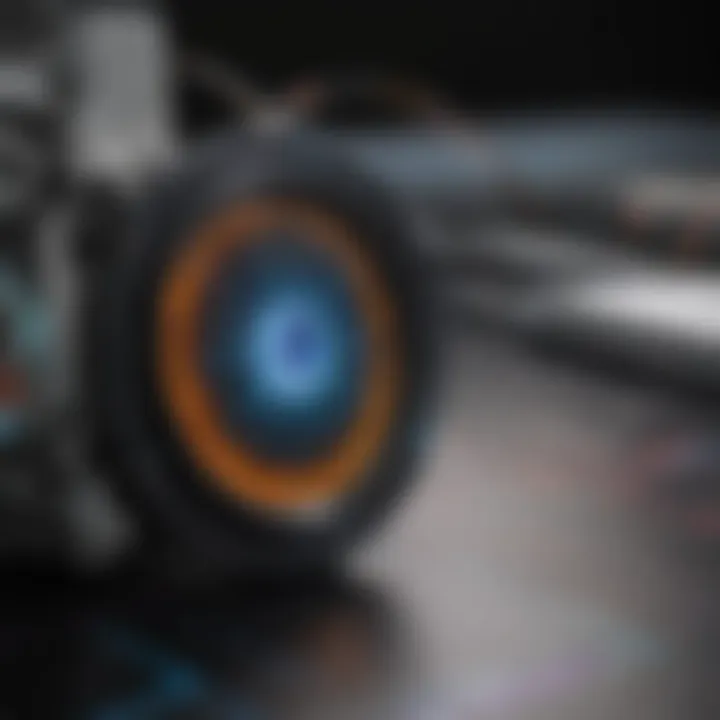
The key characteristic here is that these noise sources can heavily affect the reliability of the collected data, making the interpretation of results difficult. This situation is highly relevant for this article, as the integrity of findings can hinge on this ratio.
One unique feature of addressing signal-to-noise ratio issues is that researchers often need to refine their photodetectors and optimizing data processing algorithms. Despite the push for clarity in readings, the challenge remains: enhanced signal quality over a noisy backdrop can be as elusive as catching shadows. The ramifications of poor signal quality may lead to undervalued findings or even misguided interpretations.
Biological Variability
Variances in expression levels
The biological variability aspect plays a crucial role in how fiber photometry is applied. Variances in expression levels of the genetic indicators used can lead to discrepancies in data interpretation. Different animals or even different cells within the same animal can express the indicators at varying intensities, impacting the reliability of the measurements.
The key characteristic here is that those expressions may not always correlate directly with neural activity, leading to potentially misleading results. Variability becomes a crucial point of discussion since it not only complicates experiments but can also influence the conclusions drawn. The unique feature of this variability is that it emphasizes the need for carefully structured controls to account for differences in baseline expression levels.
On the downside, accounting for expression variances can complicate data analysis, leading to prolonged study timelines and the necessity to interpret results carefully.
Influence of environmental factors
Lastly, the influence of environmental factors cannot be understated. This aspect encompasses external conditions such as light, temperature, and even the animal's behavior, which can all sway experimental outcomes. The key characteristic here is the multitude of ways in which environmental factors may interact during the collection process. For instance, variations in light conditions may affect how well the optical fibers can deliver and receive signals.
This influence can also play a beneficial role when examining how neural activity reacts to different stimuli, revealing insights into behavioral responses. However, the unique feature of this influence is that it overwhelms data with outside variables, making it challenging to pinpoint core reactions.
The disadvantage is obvious; if researchers don't account for these factors, they risk misinterpreting the neural dynamics, leading to flawed conclusions.
Understanding these limitations and challenges equips researchers to use fiber photometry smarter, shedding light on how to navigate the complexities of this evolving field.
Future Directions in Fiber Photometry
As we look ahead in the field of neuroscience, the advancements in fiber photometry offer promising avenues for research and application. This section delves into how fiber photometry not only enhances the comprehension of complex neural activities but also indicates substantial future potential for the technology. The emphasis is placed on technological innovations and expanding applications that stand to reshape the way we understand brain function.
Technological Innovations
Developments in miniaturization
One pivotal trend in fiber photometry is miniaturization, which essentially means shrinking down the tools and devices used in these experiments. Advancements in this area allow researchers to access delicate regions in the brain that were previously challenging to study. The beauty of miniaturization lies in its ability to facilitate experiments in a variety of settings, from behavioral studies to in vivo trials. With smaller devices, researchers gain the flexibility to move freely, allowing for experiments that can closely mimic natural environments for the subjects.
A key characteristic of miniaturization is portability. Compact devices enable researchers to gather data without the overwhelming constraints imposed by bulky equipment. This not only benefits lab work but also opens doors for field studies, where traditional gear would be impractical. However, a downside is the potential loss of some detection sensitivity or spatial resolution that larger instruments might offer.
Advancements in multiplexing
Another exciting development is advancements in multiplexing, which refers to the ability to measure multiple signals at once. This technology allows researchers to observe several neuronal activities simultaneously rather than sequentially. It marks a significant leap from traditional methods, potentially enriching the datasets one can analyze. The key characteristic here is efficiency, saving valuable time while enhancing the breadth of information gleaned from experiments.
Multiplexing not only elevates the amount of data collected but also aids in understanding the interactions between different neural circuits. Such a capability can lead to deeper insights into how various regions of the brain cooperate or compete in real-time. Nonetheless, engineering a system capable of effective multiplexing can come with complexities, complicating the analysis of data. Still, the advantages of gathering comprehensive datasets cannot be overstated.
Expanding Applications
Exploring new brain regions
The evolution of fiber photometry inevitably leads researchers towards exploring new brain regions. This exploration is vital; different areas of the brain engage in a host of functions, and understanding how these regions operate can illuminate pathways for tackling neurological disorders. Fiber photometry, with its minimally invasive nature, allows for insights into brain regions that would otherwise remain in the dark.
The appeal of investigating new areas predominantly lies in uncovering previously unexamined neuronal links and interactions. Researchers can track not just where activity is occurring, but also correlate that with behavioral responses and environmental stimuli. A challenge resides in the complicated architecture of the brain, where pinpointing signals can become overbearing, yet the rewards from rich, untouched data accelerate the need for further exploration.
Cross-species comparisons
Cross-species comparisons provide another layer of depth to the applicability of fiber photometry. By observing how various species exhibit similar or distinct neural patterns, scientists gain crucial insights into evolutionary biology and behavior. The crucial trait of this approach is its universality, as findings in one species might help to inform studies in another, ultimately enhancing our understanding of neurological processes across the animal kingdom.
However, the challenge is ensuring that the interpretations remain valid across species. Variances in brain anatomy and function can sometimes lead to misleading conclusions. Therefore, a nuanced understanding is required. Yet, the potential for identifying conserved neural strategies and mechanisms across species adds a compelling rationale for continued efforts in this field of study.
"As fiber photometry advances, it bridges gaps in our understanding of neural dynamics across various contexts and species, offering a promising horizon for neuroscience.”
In summary, the future directions in fiber photometry appear both bright and multifaceted. The technological innovations such as miniaturization and multiplexing promise to expand how researchers gather and interpret neural data. Concurrently, expanding applications to include new brain regions and cross-species analyses allow for a richer understanding of brain function and evolution.
Closure
In wrapping up the discourse on fiber photometry, it’s evident that this technique has carved a significant niche in modern neuroscience research. Its ability to provide real-time insights into neural activity while minimizing invasiveness fosters a style of research that's not only ethical but also rich in detail. As we dive into the discussion, it’s crucial to acknowledge a few key elements that underlie the impact of fiber photometry.
Summary of Key Insights
The advantages brought forth by fiber photometry are manifold. This technology allows for:
- Effective neural monitoring: With its capability of imaging at depths previously unreachable, researchers can investigate previously obscured brain regions.
- Integration of behavior and biology: The link between observed behaviors and underlying neural mechanisms is illuminated, providing a holistic understanding of how brain activity relates to actions.
- Reduced invasiveness: Compared to traditional recording methods, fiber photometry reduces the physical toll on subjects, thereby opening doors for studies on sensitive or vulnerable populations.
This seamless blend of optical and electrical methodologies brings forth a new era in neuroscience research.
Moreover, the advances in miniaturization and multiplexing bring fiber photometry closer to everyday applications in clinical and research settings. The dual capability of measuring calcium responses in conjunction with subjective behaviors equips scientists with a sharper toolkit for understanding neural circuits.
Implications for Future Neuroscience Research
Looking ahead, the importance of fiber photometry in neuroscience research cannot be overstated. As technology strides forward, this method is likely to evolve and adapt in several ways:
- Expanding the scope of research: The ability to explore new brain regions could lead to novel insights into areas like memory, decision-making, and even psychiatric disorders.
- Cross-species comparisons: The method’s versatility to function in various animal models allows for comprehensive studies that could bridge gaps in our understanding of comparative neurobiology.
- Potential therapeutic avenues: With increased understanding of neural pathways comes the possibility to develop targeted therapies for neurological disorders, thus impacting treatment protocols in a tangible manner.