Innovations in Able Bioreactors: A Comprehensive Review
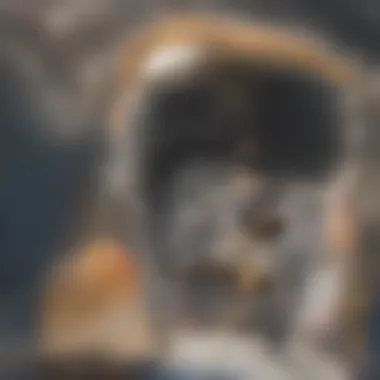
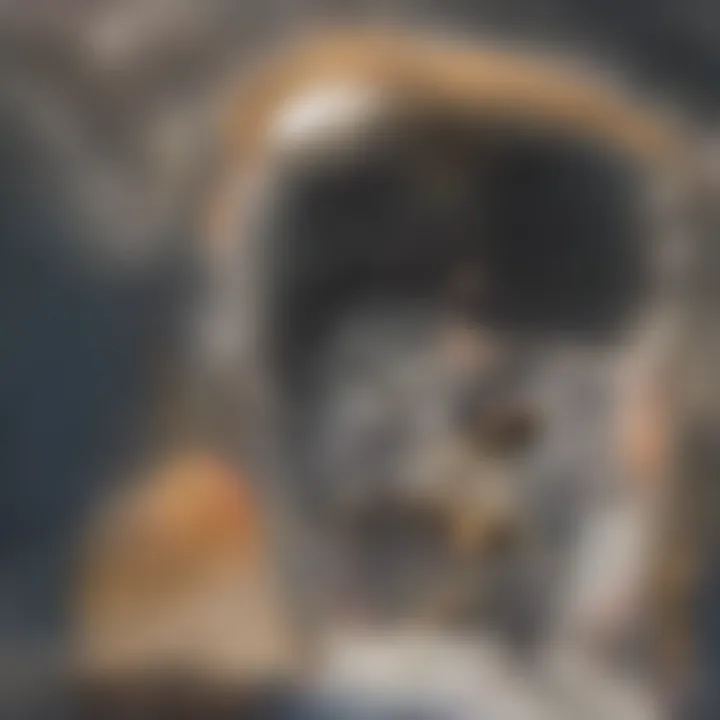
Intro
Able bioreactors serve as pivotal instruments in various fields, from pharmaceuticals to agriculture. Their ability to cultivate microorganisms or cells efficiently makes them essential for producing valuable products. In recent years, advancements in technology have transformed this concept, leading to new applications and improved efficiencies. Understanding the basic principles and applications of able bioreactors is vital for anyone involved in biotechnology, environmental research, or pharmaceutical development. This article aims to explore these bioreactors in depth, focusing on their design, application, and the latest innovations that drive the industry forward.
Defining Able Bioreactors
Able bioreactors represent a significant advancement in biotechnology, enabling the controlled cultivation of microorganisms and cells for various applications. These systems are crucial in sectors such as pharmaceuticals, agriculture, and environmental engineering. Understanding able bioreactors is important as they provide a platform that enhances productivity, efficiency, and sustainability in biological processes.
Characteristics of Able Bioreactors
Able bioreactors possess several key characteristics that distinguish them from traditional bioreactors. They are designed with precision control mechanisms that allow for optimal growth conditions, such as temperature, pH, and dissolved oxygen levels. This precision is vital for maximizing yield and maintaining product quality. Moreover, able bioreactors often incorporate advanced technology for real-time monitoring, which ensures compliance with stringent production standards and reduces the risk of contamination.
In addition, these bioreactors are often made from materials that ensure durability and compatibility with various biological agents. The materials used also support easier sterilization processes and reduce the chances of leaching harmful substances into biological blends. Overall, the characteristics of able bioreactors facilitate a more efficient and reliable production environment compared to their conventional counterparts.
Comparison with Traditional Bioreactors
When comparing able bioreactors to traditional bioreactors, there are many distinctions in terms of design, functionality, and operational output. Traditional bioreactors typically operate on fixed parameters and may not adapt to the dynamic needs of the biological processes they support. In contrast, able bioreactors are designed with flexibility in mind, allowing for adjustments in real time based on metabolic requirements.
Another prominent difference is the integration of automation in able bioreactors, which significantly reduces human error and enhances reproducibility. Automated systems can continuously monitor critical parameters and make instant adjustments as necessary. This contrasts with traditional systems that often rely heavily on manual intervention.
"The adaptability and automation seen in able bioreactors denote a monumental shift towards precision biotechnology."
Moreover, the end products produced in able bioreactors often benefit from improved quality and consistency. This is particularly significant in biopharmaceutical applications, where the purity and stability of the product are paramount. Hence, the enhancements in performance, yield, and product quality make able bioreactors a superior option for modern biotechnology.
In summary, the definition and understanding of able bioreactors highlight their potential impact on various industries. By exploring their characteristics and comparing them with traditional systems, one can appreciate their role in driving innovation and efficiency in biotechnology.
Operational Principles
Understanding the operational principles of able bioreactors is essential. These principles define how bioreactors function and directly impact their efficiency and effectiveness. This section looks at two critical aspects: the mechanisms of action and the control systems that govern bioreactors. Each of these elements plays a vital role in maximizing productivity and ensuring optimal conditions for biological processes.
Mechanisms of Action
The mechanisms of action in able bioreactors involve the various processes by which biological materials interact with the reactor environment. These can include biological processes like fermentation, cell culture, or enzyme activity. Each mechanism is designed to promote the desired reaction while minimizing by-products that could hinder productivity.
Key aspects of these mechanisms include:
- Mass transfer: Ensuring that nutrients and gases are efficiently delivered to the microorganisms or cells.
- Temperature regulation: Controlling operational heat levels to create the ideal environment for biological activity.
- Agitation: Mixing the contents to distribute reactants evenly and enhance process kinetics.
- pH control: Maintaining the right acidity or alkalinity to optimize enzyme activity and microbial growth.
These elements work together to create a stable environment that supports robust biological activity. A clear understanding of these mechanisms allows researchers and operators to optimize processes further and could lead to significant efficiency gains.
Control Systems in Bioreactors
Control systems are formidable when it comes to managing able bioreactors. They integrate various tools and technologies to monitor and adjust the operational conditions in real time. Effective control systems contribute significantly to the success of bioreactor applications.
The components within these systems include:
- Sensors: Measure parameters like temperature, pH, and dissolved oxygen. They provide critical feedback for maintaining suitable conditions.
- Actuators: Devices that implement changes based on sensor input, such as adjusting temperature or nutrient flow.
- Control software: Manages the data collected by sensors and directs the actuators to maintain desired conditions.
Effective control systems not only enhance product yield but also improve the reproducibility of the results.
The benefit of having robust control systems cannot be overstated. They lead to increased reliability, reduced operational costs, and enhanced scalability of bioprocesses.
In essence, both operational mechanisms and control systems are critical in ensuring that able bioreactors can meet the demands of various applications. Without these principles, the bioreactor's efficacy would be significantly compromised.
Design Considerations
In the field of bioreactors, design considerations hold significant weight. They shape how effectively these systems can meet their intended purposes. The design must address key features, such as scalability, material choice, and system integration. Each of these aspects influences the operational efficiency and success of the bioreactor in diverse applications. A well-thought-out design can lead to improved productivity and reduced operational costs, making it an essential focus for researchers and industry professionals alike.
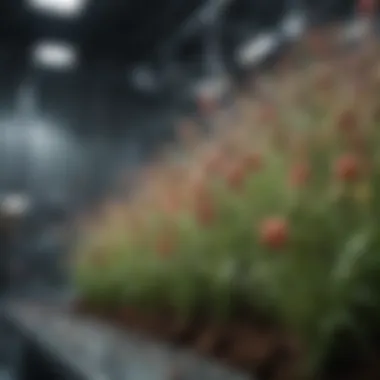
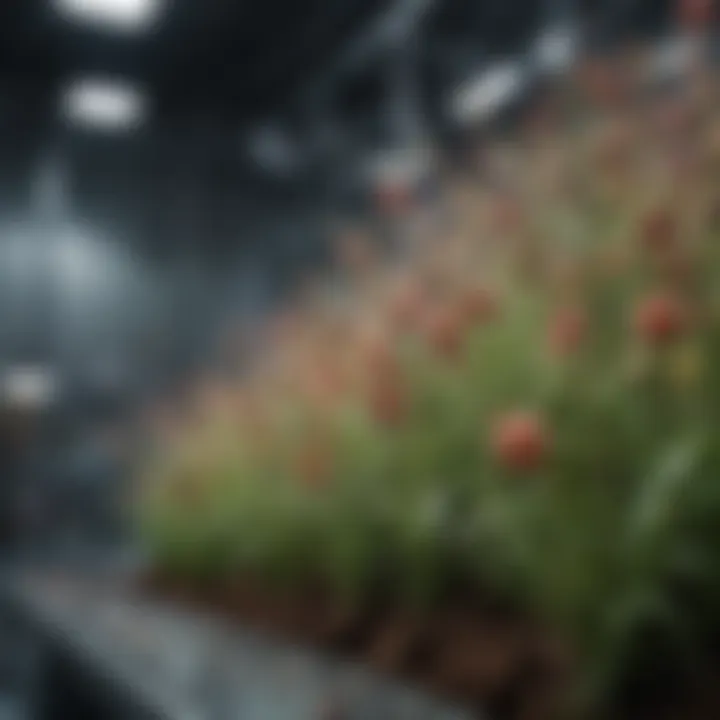
Material Selection
Material selection is a fundamental element in bioreactor design. The materials used must be compatible with the biological processes taking place within the reactor. For example, stainless steel is commonly chosen for its durability and sterility, especially in pharmaceutical applications. However, glass may also be used for smaller-scale experiments due to its ease of cleaning and transparency.
When selecting materials, factors like temperature resistance, pressure tolerances, and chemical stability are critical. Additionally, the material should not interfere with the biological processes, ensuring optimal growth conditions for the cultures involved.
Scale-Up Techniques
Understanding scale-up techniques is crucial for transitioning bioprocesses from laboratory to industrial scale. Scaling affects not only the physical dimensions of the bioreactor but also the biological and mechanical parameters necessary for efficient operation.
Successful scale-up involves a thorough grasp of mass transfer dynamics, mixing patterns, and heat transfer characteristics. These principles must be replicated or adjusted in larger systems.
Several strategies can facilitate effective scale-up:
- Empirical Scaling: Utilizing data from smaller designs to inform the construction of larger systems.
- Mathematical Modeling: Applying mathematical models to predict behavior in various scales.
- Pilot Studies: Conducting pilot studies using intermediate sizes ensures that the system operates within acceptable parameters before full-scale implementation.
In summary, both material selection and appropriate scale-up techniques are vital to the success of able bioreactors. By addressing these design considerations, practitioners can enhance the performance and efficiency of bioreactor systems across multiple applications.
Applications in Pharmaceuticals
The application of able bioreactors in pharmaceuticals is paramount. These systems provide a controlled environment for the cultivation of cells and the production of proteins and other biologically active compounds. Their importance cannot be overstated due to the increasing demand for biopharmaceuticals and the complexity of the production processes involved.
One of the primary advantages of using able bioreactors in this field is their ability to facilitate large-scale production. This is essential for biopharmaceutical manufacture, where the biological products must be produced consistently and in sufficient quantities to meet regulatory requirements and market demand.
Moreover, the control features of able bioreactors allow for an optimization of growth conditions. Specific temperature, pH, and oxygen levels can be meticulously maintained. These features ensure that cell lines yield high-quality products, enhancing the efficacy of pharmaceuticals.
When discussing the applications of able bioreactors in pharmaceuticals, two critical areas stand out: the production of biopharmaceuticals and cell culture techniques.
Production of Biopharmaceuticals
Biopharmaceuticals represent a significant advancement in medicine, often derived from biological sources. Able bioreactors play a crucial role in the production of these therapies. They are utilized for the large-scale manufacture of monoclonal antibodies, vaccines, and recombinant proteins.
The success of these processes is largely attributed to the versatile design of able bioreactors, which can be configured to support a variety of cell types, including mammalian, yeast, and bacterial cells. Each of these types may require specific growth conditions. Bioreactors can be adjusted to accommodate these variables, leading to robust production methods.
Key points regarding the production of biopharmaceuticals include:
- Scalability: Able bioreactors can easily transition from research to large-scale production.
- Quality Control: Continuous monitoring ensures product consistency and regulatory compliance.
- Cost-effectiveness: With efficient design and operation, production costs can be reduced, making drugs more accessible.
Cell Culture Techniques
Cell culture techniques underpin the production of many biopharmaceuticals. Able bioreactors enhance these techniques by optimizing the micro-environment for cell growth.
In a bioreactor, cells can be cultivated in suspension or attached to surfaces, depending on their growth requirements. This flexibility means that bioreactors can support a broader range of applications, from basic research to commercial production. Additionally, advancements in automation technologies enable real-time monitoring and adjustments in the culture conditions, which can improve productivity and cell viability.
The application of able bioreactors in cell culture techniques provides tangible benefits like:
- Increased yield: Tailoring conditions for specific cell lines can expand production capacity.
- Enhanced cell viability: Optimized conditions lead to healthier cells and higher quality products.
- Streamlined processes: Automation reduces labor costs and increases precision.
The integration of able bioreactors in biopharmaceuticals marks a significant step forward in the efficiency and quality of drug production, addressing global health needs with innovative solutions.
In summary, the applications of able bioreactors in pharmaceuticals are multifaceted. They are essential for both the production of biopharmaceuticals and the implementation of advanced cell culture techniques. Their ability to adapt to various production needs makes them a backbone of modern medical manufacturing.
Applications in Agriculture
The applications of able bioreactors in agriculture represent a critical intersection of biotechnology and sustainable practices. This section elucidates the various functions of these systems, emphasizing both bioremediation processes and sustainable fertilizer production. These two areas are pivotal as they not only contribute to agricultural productivity but also address environmental concerns.
Bioremediation Processes
Bioremediation using able bioreactors focuses on the use of microorganisms to alleviate soil and water pollution. Contaminants such as heavy metals, pesticides, and hydrocarbons pose significant risks to agricultural output and ecosystems. Utilizing able bioreactors, tailored microbial communities can effectively degrade these pollutants. This technology can be advantageous in several ways:
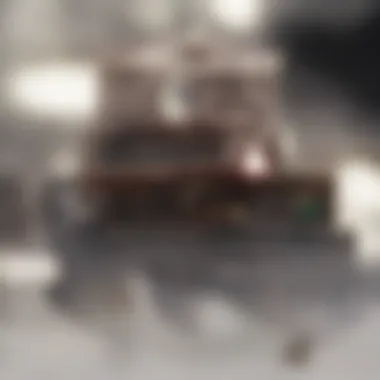
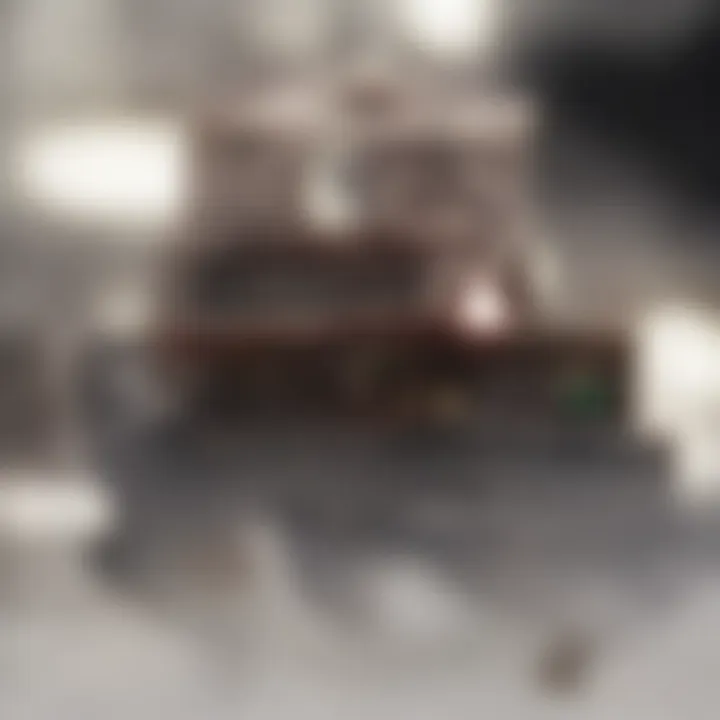
- Efficiency: Able bioreactors enhance the growth conditions for specific microorganisms, enabling them to break down contaminants rapidly.
- Cost-effectiveness: This system offers a less expensive alternative compared to conventional remediation methods, such as excavation or chemical treatments.
- Environmental sustainability: Employing biological methods minimizes the introduction of harmful chemicals into the environment.
Bioremediation processes not only clean up polluted sites but also restore soil health. Cleaner soils can lead to improved crop yields and better food safety. In addition, by managing contaminants, agriculture can continue to thrive without compromising the environment.
"Bioremediation sits at the core of sustainable agriculture, proving essential in revitalizing degraded land."
Sustainable Fertilizer Production
The production of sustainable fertilizers through able bioreactors is gaining traction as a viable alternative to chemical fertilizers. This process often uses organic waste materials, such as agricultural residues or food waste, to create biofertilizers. The benefits of this approach include:
- Resource recycling: Repurposing waste reduces environmental burden while providing essential nutrients for crops.
- Enhanced soil fertility: Biofertilizers contribute to soil microbial diversity and improve soil structure, leading to healthier plants.
- Reduced chemical reliance: Using bio-based fertilizers can decrease dependency on synthetic fertilizers, which are often associated with soil degradation and water pollution.
Implementing these biofertilizer systems in agricultural practices supports a circular economy model. Sustainable fertilizer production not only meets the nutrient needs of plants but also aligns with modern societal aspirations to produce food sustainably.
These applications of able bioreactors in agriculture illuminate the path forward in both innovation and sustainability. They represent an essential movement towards practices that are both profitable and environmentally responsible.
Environmental Impact Assessment
In the context of able bioreactors, the Environmental Impact Assessment (EIA) serves as a crucial framework for analysing the potential effects of bioreactor systems on the environment. As industries strive for sustainability, understanding these impacts is essential. The significance of EIA lies in its capacity to identify, predict, evaluate, and mitigate adverse effects before they occur. This proactive approach helps to ensure that bioreactor operations align with environmental regulations and broader sustainability goals.
EIA integrates various components of the bioreactor lifecycle, promoting more sustainable practices. These components include resource consumption, waste generation, emissions, and potential ecological footprints. Artists of clean technology should consider assessments to minimize ecological degradation. A rigorous assessment can lead to benefits such as enhanced public trust and improved operational efficiency.
Lifecycle Analysis of Bioreactor Systems
Lifecycle analysis (LCA) is a systematic approach to evaluate the environmental aspects of product systems throughout their lifecycle, from raw material acquisition to disposal. In the case of able bioreactors, LCA assesses the impact of bioreactor systems on environmental sustainability.
- Raw Material Sourcing: The selection of materials for bioreactor construction significantly affects its environmental profile. Sustainable sourcing minimizes resource depletion and lowers carbon footprints.
- Energy Consumption: Bioreactors require energy for various functions, including mixing, temperature control, and aeration. Analyzing energy use helps identify opportunities for efficiency improvements.
- Waste Management: Proper management of by-products generated during bioreactor operation is vital. LCA can identify waste treatment options that reduce landfill contributions and enhance recycling efforts.
- End-of-Life Considerations: Understanding how bioreactors can be decommissioned responsibly is important for minimizing long-term impacts.
By conducting a thorough lifecycle analysis, industries can gain insights into the environmental performance of their bioreactor systems. This, in turn, lends itself to better decision-making in design and operational practices.
Regulatory Compliance and Standards
Regulatory compliance is paramount for any technology, and able bioreactors are no exception. The compliance landscape comprises various national and international regulations governing environmental, health, and safety standards.
- ISO Standards: Many organizations aim to meet ISO standards related to environmental management systems. Adhering to these standards helps ensure that best practices are followed, contributing to both reliability and trust.
- Legislation: Laws regarding waste management, emissions limits, and resource usage exist to protect environmental and public health. Compliance with such regulations is not only a legal obligation, but fosters corporate responsibility.
- Industry Best Practices: Aligning with industry norms can enhance credibility and competitiveness. Participating in relevant certifications showcases commitment to sustainability and operational excellence.
Effective compliance not only addresses regulatory needs but can also provide an avenue for innovation and operational improvements.
Technological Advancements
Technological advancements in able bioreactors represent a critical area of development. These innovations not only enhance efficiency but also allow for better monitoring, control, and overall productivity. As the demands in sectors such as pharmaceuticals and agriculture evolve, integrating advanced technology is essential. The future of bioreactor functionality heavily relies on these technological strides.
Automation and Monitoring Technologies
Automation has become a cornerstone in the efficient management of able bioreactors. The application of automated systems minimizes human error and enhances reproducibility. Through the use of real-time monitoring technologies, operators can gain insights into various operational parameters such as pH levels, temperature, and dissolved oxygen. This data plays a significant role in optimizing the growth environment for cultures.
Additionally, the integration of advanced sensors and control systems results in a seamless operational flow. These sensors can respond instantaneously to fluctuations in environmental conditions, enabling adjustments on-the-fly. The ability to monitor these parameters continuously has led to increased productivity and reduced downtime. Here are some benefits of automation in bioreactors:
- Increased Efficiency: Automation allows for the optimization of processes, leading to higher yields.
- Enhanced Data Accuracy: Real-time data collection improves decision-making.
- Reduced Labor Costs: Minimizing the need for manual oversight frees up valuable human resources.
- Improved Safety: Automated systems can manage dangerous conditions without putting human operators at risk.
Innovative Design Approaches
The design of able bioreactors is evolving with innovative approaches that focus on enhancing performance and sustainability. Modular designs are gaining traction. This allows bioreactors to be easily scaled or adapted to different production needs, eliminating the need for entirely new systems. Collaborative robots, also known as cobots, can work alongside human operators, further enhancing productivity while ensuring a flexible workspace.
Moreover, the utilization of sustainable materials in bioreactor construction is a growing trend. These materials not only reduce environmental impact but also enhance the bioreactor's lifespan.
Key considerations in innovative design include:
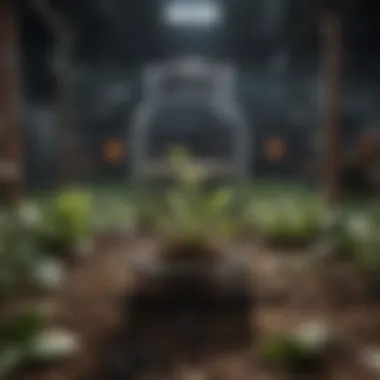
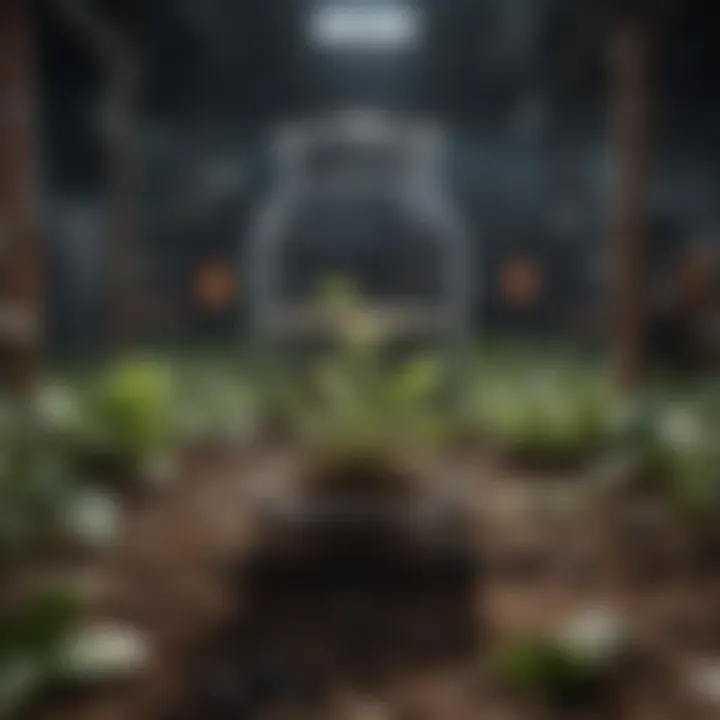
- Flexibility and Scalability: The ability to adapt systems for various applications.
- Sustainable Practices: Using eco-friendly materials to reduce waste and energy consumption.
- Replication of Conditions: Designing systems to mimic natural environments as closely as possible allows for enhanced culture growth.
Challenges in the Field
The challenges in the field of able bioreactors represent critical considerations for stakeholders involved in their design, operation, and application. Understanding these challenges is essential not only for ensuring optimal functionality but also for fostering innovation in an evolving biotechnological landscape. Addressing these challenges can lead to improved efficiency, reduced costs, and enhanced performance across various applications.
Cost Efficiency and Investment
Cost efficiency plays a prominent role in the successful adoption of able bioreactors. The initial investment for setting up advanced bioreactor systems can be substantial. Organizations must weigh the costs against potential benefits. Here are some key points to consider:
- Capital Expenditure: The purchase of bioreactors and associated equipment can demand significant capital. This cost must be justified by projected returns on investment.
- Operational Costs: Ingredients, maintenance, and energy consumption comprise ongoing costs that can affect overall efficiency. Organizations need to evaluate strategies to minimize these expenses.
- Economic Viability: The market for bio-based products is rapidly evolving. Companies that invest wisely in able bioreactor technologies can position themselves advantageously in this market.
Because of these considerations, organizations are looking for ways to enhance the cost-effectiveness of bioreactor deployment. This can include integrating automation systems to reduce labor costs, optimizing resource usage, and employing predictive maintenance techniques to prevent costly downtime.
"Investing in advanced technologies can seem daunting, but the potential for returnβboth financial and sustainableβcan be transformative."
Scaling Limitations
Scaling limitations are another significant challenge facing the effective use of able bioreactors. Transitioning from laboratory-scale systems to industrial-scale operations involves numerous complexities. Here are some aspects to consider:
- Process Reproducibility: Maintaining consistency in processes during scale-up is challenging. Variations can lead to reduced yield and performance.
- Mixing and Mass Transfer: As bioreactor volume increases, the efficiency of mixing and mass transfer may decline. This can negatively impact the cultivation of cells or microorganisms.
- Heat and Oxygen Transfer: Large-scale bioreactors must efficiently manage heat and oxygen transfer. Inadequate control in these areas can result in suboptimal growth conditions for biological cultures.
In summary, addressing scaling limitations requires innovative engineering solutions and thorough planning. Stakeholders must focus on maintaining process integrity while adapting to larger systems. This will ensure that the advantages of able bioreactors can be fully realized across diverse applications.
Case Studies of Able Bioreactor Implementations
Case studies of able bioreactor implementations provide critical insights into how these technologies function in real-world applications. By examining specific instances, we can better understand the practical benefits and challenges associated with able bioreactors. The analysis of these examples is vital for both current practitioners and future researchers in the field.
Successful Industry Applications
In various sectors, able bioreactors have demonstrated significant advancements. Their successful applications highlight the benefits of using advanced bioreactor technologies. One notable example is the use of able bioreactors in the production of insulin. Companies like Genentech have implemented these systems to enhance yield and reduce production times. The improvement in efficiency is crucial not only for economic factors but also for meeting increasing healthcare demands.
Another industry using these bioreactors effectively is biopharmaceuticals. Successful implementations include monoclonal antibody production. The application of able bioreactor systems allows for better control over the growth conditions of mammalian cell cultures. This results in higher purity and yield of the desired product.
Research Driven Innovations
Research is propelling the development of able bioreactors, leading to innovations that further enhance their capabilities. For example, a recent study investigated the combination of CRISPR technology with able bioreactor designs. This interdisciplinary approach aims to improve cell engineering processes. Such innovations can facilitate the production of more complex biomolecules, which are often challenging to produce using traditional bioreactors.
Additionally, researchers at MIT are developing hybrid reactor systems that incorporate both biological and synthetic methodologies. These systems aim to optimize metabolic pathways, allowing for greater production efficiency. The insights gained from these research-driven innovations help to shape future designs and operational criteria within the industry.
"The translation of laboratory findings into commercially successful applications is the key to advancing bioreactor technologies."
By analyzing these case studies, stakeholders can identify trends and best practices. This further allows them to grasp the dynamic landscape surrounding able bioreactor technology. From improving existing processes to exploring new frontiers, case studies illuminate the ongoing evolution of this critical field.
Future Directions
As the field of able bioreactors continues to evolve, the exploration of future directions holds significant importance. This section discusses new opportunities to enhance bioreactor technology, focusing on emerging trends and the potential for interdisciplinary research. Recognizing these future directions is essential for pushing the boundaries of what can be achieved in biotechnology and environmental management.
Emerging Trends in Bioreactor Technology
Emerging trends in bioreactor technology showcase a shift towards smarter, more efficient systems. Notable developments include:
- Integration of Artificial Intelligence: AI is playing a pivotal role in the optimization of bioprocesses within bioreactors. By analyzing historical data and real-time metrics, AI algorithms can fine-tune conditions to maximize yield.
- Microbial Fuel Cells: These systems convert chemical energy into electrical energy, harnessing specific microbes' natural processes. Their application in bioreactors can enhance sustainability.
- Modular Bioreactor Designs: Companies are increasingly adopting modular approaches that allow for easy scaling and customization. This adaptability caters to varied production needs without extensive re-engineering.
- Bioreactor Automation: The shift towards autonomous operation is gaining traction. Automated monitoring systems can adjust parameters with precision, reducing the need for manual intervention and minimizing human error.
These trends not only signify advancements in technology but also emphasize sustainability and efficiency. As methods progress, industries must adapt to leverage these innovations effectively.
Potential for Interdisciplinary Research
The potential for interdisciplinary research in bioreactor applications cannot be overstated. Collaboration across various fields can produce novel solutions and improve bioreactor efficiency. Some key areas of exploration include:
- Biotechnology and Engineering: Combining biological insights with engineering principles can lead to the development of more robust bioreactor designs capable of handling complex biological reactions.
- Environmental Science and Chemistry: The integration of chemical analysis within bioreactor systems can enhance bioremediation techniques, allowing for more effective treatment of pollutants.
- Data Science and Analytics: Harnessing data analytics in bioprocessing can result in more predictive models, leading to improved outcomes in bioproduction and enhanced decision-making.
Research collaboration can bridge knowledge gaps, fostering innovative advancements in bioreactor technology. It invites various professionals to share their insights, driving the field forward.
Interdisciplinary approaches are critical for breaking new ground in bioreactor technology, promoting shared knowledge and innovative practices.