In-Depth Analysis of Capacitance Detectors
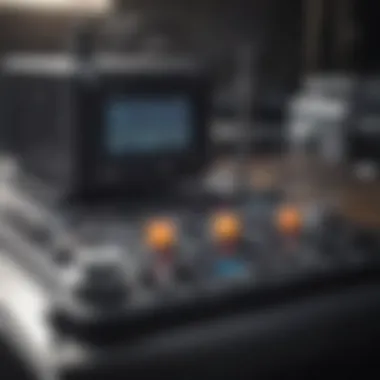
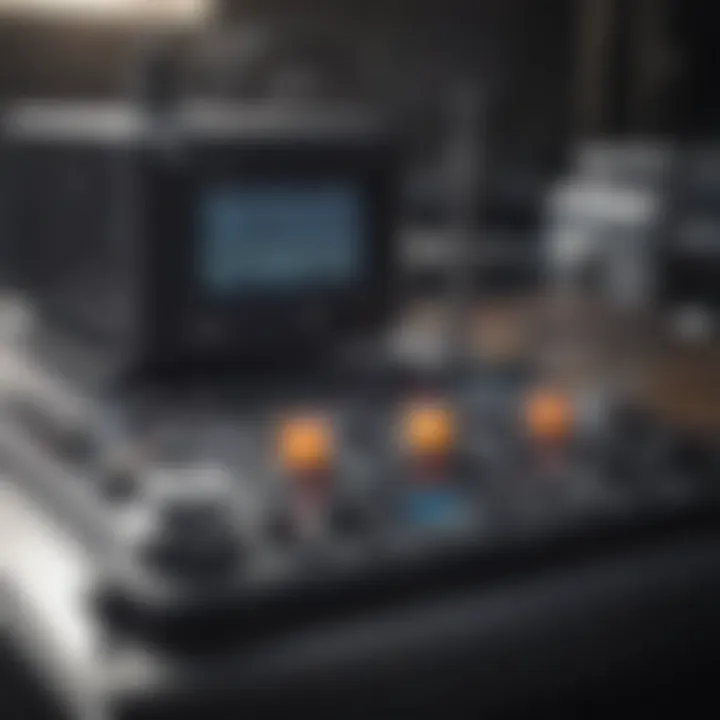
Intro
Capacitance detectors stand at the intersection of advanced scientific measurement and practical applications in various industries. These instruments are designed to gauge electrical capacitance with a high degree of accuracy, making them invaluable tools in multiple sectors. From the characterization of materials to the monitoring of environmental conditions, their versatility is significant across scientific research and industrial settings.
Understanding capacitance detection requires delving into its fundamental principles, examining various types, and appreciating their advantages and limitations. Not only do these factors shape the operational framework of capacitance detectors, but they also influence their adoption in different fields.
This article aims to shed light on the historical context and importance of capacitance detectors, providing a comprehensive overview that will benefit students, researchers, and professionals alike. The insights discussed herein will highlight how these instruments measure capacitance, the technological advancements that have propelled their use, and the impact they have on contemporary applications.
Prelims to Capacitance Detectors
Capacitance detectors play an essential role in various scientific and industrial fields. They provide accurate measurements of electrical capacitance, which is critical in applications ranging from material analysis to advanced sensor technology. Understanding the principles and functionality of these instruments is vital for students, researchers, educators, and professionals who engage with electronic measurement systems.
Definition and Functionality
Capacitance detectors are instruments that measure capacitance, the ability of a system to store an electrical charge. They operate on the principle that capacitance depends on the physical characteristics of the materials involved, as well as their geometric arrangement. In essence, the primary function of these devices is to provide precise readings necessary for evaluating electrical components and systems.
Capacitance is fundamentally defined by the formula:
C = Q/V
where C is the capacitance in Farads, Q is the charge in Coulombs, and V is the voltage in Volts. By measuring capacitance, these detectors can infer information about the dielectric properties of materials, which is crucial in fields like electronics, environmental monitoring, and biomedical applications.
Historical Context
The development of capacitance detectors can be traced back to the early advancements in electricity and electromagnetism. Early capacitors, made from simple materials, laid the groundwork for more sophisticated measuring instruments. Over time, the evolution of these devices has seen significant improvements in design and functionality, leading to the modern capacitance detectors we use today.
In the 20th century, innovations in electronics led to the creation of various capacitance measurement devices. The introduction of analog systems paved the way for digital technology that we find prevalent today. Today’s capacitance detectors, equipped with advanced features such as automatic calibration and digital displays, allow for greater accuracy and ease of use in real-world applications.
"The exploration of capacitance not only enriches our understanding of physical phenomena but also drives innovation across multiple sectors, from industrial automation to healthcare research."
Understanding the comprehensive history and evolution of capacitance detectors provides essential insights into their current functionality and applications. It establishes a framework for future innovations that may further enhance the usability and accuracy of these critical instruments.
Basic Principles of Capacitance Measurement
Understanding the principles of capacitance measurement is crucial for grasping how capacitance detectors operate. Capacitance, in basic terms, is the ability of a system to store an electrical charge. In a world increasingly reliant on precise electronic measurements, the importance of this topic cannot be overstated. It sets the foundation for both the functionality and the applications of capacitance detectors across various fields.
Understanding Capacitance
Capacitance is defined mathematically as the ratio of the electric charge stored on a conductor to the voltage across it. The standard unit of capacitance is the farad (F). Though theoretical discussions often refer to ideal capacitors, real-world applications frequently encounter non-ideal behaviors. Factors such as dielectric materials and the physical arrangement of conductors significantly affect capacitance. Understanding these aspects is vital, as it informs the design and optimization of capacitance detection systems.
Key factors in capacitance include:
- Dielectric Material: Different materials influence capacitance significantly. Materials like air, glass, and ceramic have various dielectric constants, affecting their storage capabilities.
- Surface Area: The larger the surface area of the conductive plates, the greater the capacitance. This factor must be considered while designing sensors.
- Distance Between Plates: Reducing the distance between plates will increase capacitance. This is a vital design principle in creating more sensitive detectors.
Measurement Techniques
The measurement of capacitance, despite its apparent simplicity, involves a range of sophisticated techniques to ensure accuracy and reliability. Traditional methods use analog circuitry to measure currents and voltages, while modern techniques are leveraging digital technologies for improved precision.
Common measurement techniques include:
- AC Capacitance Measurement: Utilizes alternating current signals to evaluate capacitance. This method is prevalent due to its high accuracy and sensitivity to low capacitance values.
- DC Bridge Methods: Involves connecting the capacitor to a bridge circuit to measure unknown capacitance against known values. This method is effective for laboratory settings.
- Time Domain Reflectometry (TDR): An advanced technique that involves sending a pulse through a transmission line and analyzing the reflection to determine capacitance values. It is particularly useful in cable tracing and field measurements.
"For accurate capacitance measurements, calibration against known standards is critical to attain reliable readings."
The choice of measurement technique often depends on the specific requirements of the application. Factors such as the expected range of capacitance, environmental conditions, and the presence of noise must be taken into account. Each technique has its benefits and drawbacks, and understanding these can guide users in selecting the most suitable method for their applications.
Types of Capacitance Detectors
The various types of capacitance detectors play a crucial role in understanding how these instruments function effectively across different applications. Each type has unique attributes that make it suitable for specific scenarios. It is essential for researchers and practitioners to recognize these differences to make informed choices when selecting a capacitance detector for their particular needs.
Analog Capacitance Detectors
Analog capacitance detectors have been around for a long time. They use a simple electronic circuit to measure capacitance based on the relationship between voltage and frequency. Their designs often include capacitors, resistors, and operational amplifiers to translate the changes in capacitance into a readable voltage output. The readings are usually displayed on a dial or an analog meter.
These devices are valued for their reliability and ease of use. One major advantage is their ability to provide real-time measurements without requiring complex digital processing. However, analog detectors tend to have limitations in terms of precision and susceptibility to noise, which can affect their readings, especially in environments with high electromagnetic interference.
Digital Capacitance Detectors
Digital capacitance detectors offer several enhancements over their analog counterparts. By utilizing advanced technology, they can provide more accurate measurements and feature digital displays, which improve readability. They typically employ a microcontroller or a similar device to process the signals and convert them into digital values.
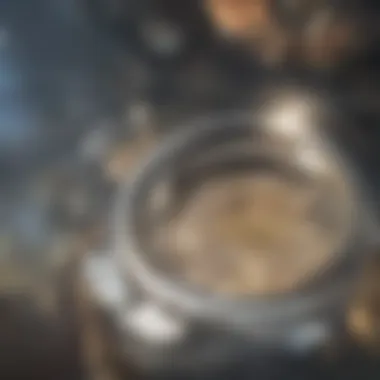
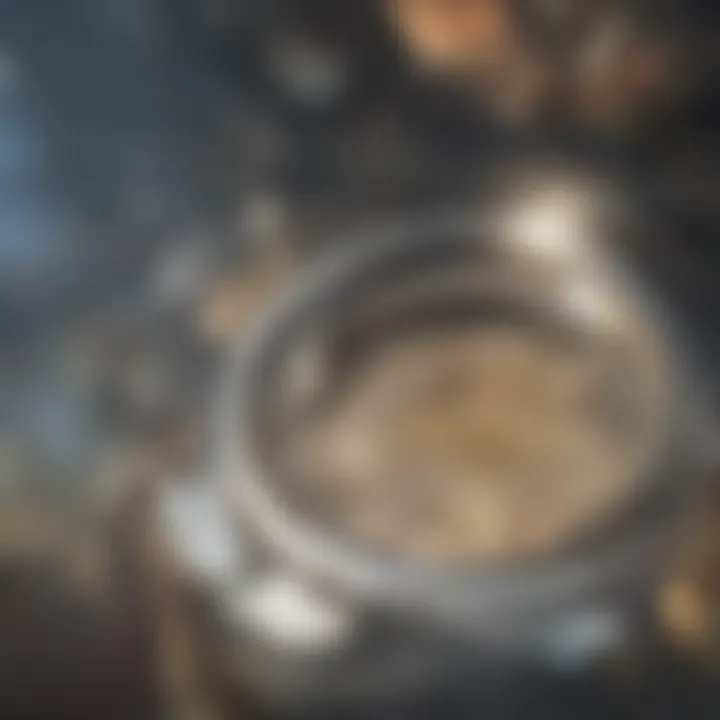
Digital detectors are especially popular due to their automated calibration processes and ability to store multiple measurement results. Furthermore, they often include features like a data logging function, which is invaluable for long-term analysis or experiments. Unlike analog devices, digital capacitance detectors are less affected by noise and environmental factors, thus enhancing their performance and reliability.
Lumped and Distributed Capacitance Sensors
Lumped and distributed capacitance sensors refer to two different measurement approaches. Lumped capacitance sensors are typically designed for localized applications where measurements of small areas or specific points are needed. They operate based on the assumption that the capacitance can be treated as a single entity, which simplifies the calculations.
On the other hand, distributed capacitance sensors measure a larger area or volume. They consider that capacitance changes over the entire sensor length, which allows for more complex and accurate readings, especially in fluid dynamics applications. These sensors are essential in certain research settings where spatial resolution of measurements is critical.
Understanding the differences between lumped and distributed capacitance sensors enables engineers and technicians to select the appropriate tools for their projects effectively.
Applications of Capacitance Detectors
Capacitance detectors play a critical role in various industries, given their sensitivity and versatility. This section will elucidate the importance of capacitance detectors across different fields, emphasizing the specific benefits and operational considerations in three primary categories: industrial uses, environmental monitoring, and biomedical applications.
Industrial Uses
In industrial settings, capacitance detectors are invaluable for measuring the dielectric properties of materials and monitoring various processes. Their high sensitivity to changes in capacitance allows for precise measurements in environments where traditional sensors may fail. For example, capacitance-based level measurement is common in silos and tanks, where it effectively determines the level of granular and liquid substances.
Another application is in the production of electronic devices, where these detectors help in assessing the quality of materials. They can identify defects in dielectric materials used in capacitors, enhancing the consistency of the final products.
In manufacturing automation, capacitance sensors are often integrated into control systems to provide instant feedback on process parameters, allowing for adjustments in real-time. This capability not only improves efficiency but also ensures the safety and reliability of industrial operations.
Environmental Monitoring
Capacitance detectors also serve an essential purpose in environmental monitoring. They measure soil moisture content by detecting changes in capacitance due to water's presence. Understanding soil moisture is key for agricultural practices, as it helps in managing irrigation systems effectively, optimizing water usage, and improving crop yields.
Moreover, these detectors are used in assessing the quality of water and air. In this context, capacitance-based sensors can detect variations in the dielectric constant of liquids, indicating contamination levels in water bodies. Similarly, they can sense humidity and other atmospheric conditions crucial for environmental studies.
In essence, the non-invasive nature of capacitance measurements makes them suitable for long-term monitoring without disturbing the physical environment, which is vital for sustainability efforts.
Biomedical Applications
In the biomedical field, capacitance detectors are emerging tools for various diagnostic procedures. They are instrumental in bio-sensing applications, where the presence of biological substances induces changes in capacitance that can be measured. For instance, these sensors can evaluate changes in tumor tissues, providing insights during cancer diagnostics.
Capacitance detection is also relevant in wearable health monitors. These devices utilize capacitance measurement to track physiological parameters like heart rate and motion activity. This information can be vital for personalized healthcare.
Overall, the adaptability of capacitance detectors across such a broad range of applications illustrates their significance in advancing technology and improving the quality of life. Their presence in industry, environmental science, and medicine marks a continuous evolution and highlights their ongoing relevance.
Advantages of Using Capacitance Detectors
Capacitance detectors offer multiple benefits that enhance their utility in a variety of applications. Understanding these advantages is essential for anyone looking to utilize these devices effectively. In this section, we will explore two prominent advantages: high sensitivity and precision, as well as non-invasiveness, which make capacitance detectors a preferred choice in many settings.
High Sensitivity and Precision
One of the foremost advantages of capacitance detectors is their remarkable sensitivity and precision. These detectors can measure even the slightest changes in capacitance, making them exceptionally valuable in both research and industrial processes. The sensitivity allows for the detection of minute variations in physical parameters such as pressure, displacement, and humidity. In various applications, this high sensitivity translates into more accurate data collection, which is critical for decision-making and analysis.
Capacitance detectors operate based on changes in the electrical capacitance due to the presence of a dielectric material or change in distance between electrodes. Because of this principle, they tend to yield higher precision than other types of sensors. For instance, in applications like level measurement in tanks, small changes in the dielectric constant can be detected, providing accurate readings that guide operational adjustments.
Non-invasiveness
Another significant benefit of capacitance detectors is their non-invasive nature. This aspect is particularly advantageous in situations where the integrity of materials or biological samples must be preserved. For example, in biomedical applications, capacitance detectors can monitor biological processes without the need to alter or disturb the subject being studied.
Non-invasive measurement techniques minimize potential contamination and disruption, leading to more reliable results. This is especially important in delicate environments like laboratories or within human bodies. The ability to gather data without intrusive methods enables researchers to conduct experiments more ethically and accurately. Moreover, this feature proves beneficial in various industrial settings where intrusive methods could lead to product degradation or safety hazards.
"Non-invasive measurement techniques are crucial for preserving the integrity of sensitive samples while obtaining accurate results."
In summary, the advantages of using capacitance detectors—high sensitivity and precision along with non-invasiveness—present compelling reasons for their employment across diverse fields. These attributes not only enhance accuracy but also ensure that the integrity of samples and processes is maintained.
Limitations of Capacitance Detectors
Understanding the limitations of capacitance detectors is crucial for researchers and practitioners in various fields. While these devices offer numerous benefits, acknowledging their constraints ensures that users can effectively utilize them. The limitations can significantly impact measurement accuracy and reliability, especially in sensitive applications.
Temperature and Humidity Sensitivity
Capacitance detectors often exhibit sensitivity to environmental factors such as temperature and humidity. Changes in temperature can alter the dielectric properties of materials surrounding the capacitive sensors. For instance, increasing temperature can lead to thermal expansion, potentially affecting the distance between electrodes. This adjustment results in variations in capacitance readings, compromising measurement consistency.
Similarly, humidity can introduce error in readings. Moisture in the environment can change the dielectric constant of materials, leading to inaccurate readings. In industrial settings, controlling environmental conditions may require additional investment in climate control systems, further affecting the overall operational costs.
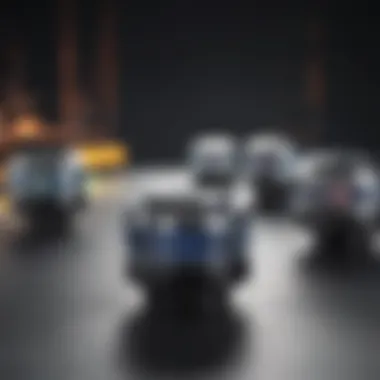
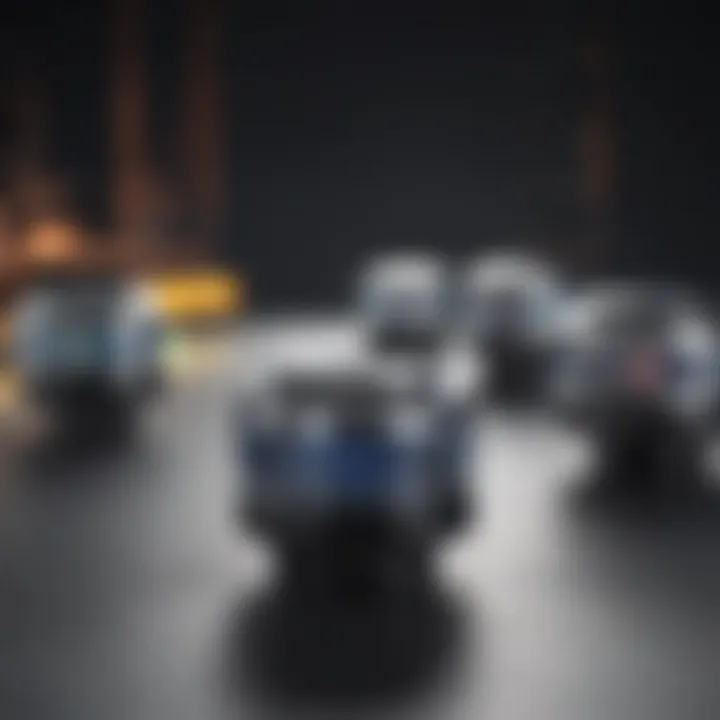
"Accurate environmental controls are vital to maintain precision in capacitance measurements."
Calibration Challenges
Calibration is another significant challenge affecting capacitance detectors. Regular calibration is essential to maintain accuracy and reliability. However, the complexity of the calibration process can vary depending on the device and its application. For instance, some capacitance detectors may require specific calibration procedures that can be time-consuming and resource-intensive.
If a device is not calibrated properly, it can lead to erroneous measurements. This situation can be critical in sectors where precision is paramount, such as biomedical applications or environmental monitoring. Furthermore, the calibration must be repeated periodically to ensure that the measurements remain accurate over time. In some cases, inconsistencies in calibration results may arise due to user error or equipment drift, necessitating further investigation and potential recalibration.
In summary, while capacitance detectors are valuable tools, users must remain cognizant of their limitations. Temperature and humidity sensitivity, along with calibration challenges, are significant considerations that can affect the devices' performance. For effective application, it is important to develop strategies to mitigate these challenges.
Advancements in Capacitance Detection Technologies
Capacitance detection technologies have evolved significantly, pushing the boundaries of measurement accuracy and application versatility. These advancements address the limitations of older sensors, offering innovative solutions that improve precision and functionality. The major advancements in this field include innovations in sensor design and integration with Internet of Things (IoT) systems. Exploring these aspects is crucial for understanding how capacitance detectors can meet the demands of modern applications.
Innovations in Sensor Design
The design of capacitance sensors has seen remarkable changes in recent years. Modern sensor designs now utilize materials and manufacturing techniques that enhance sensitivity and reduce noise. For instance, piezoelectric and organic materials are increasingly used, allowing for lighter and more responsive sensors. These advancements translate to improved measurements in complex environments where traditional sensors struggled.
Furthermore, miniaturization is a key theme. With the development of micro-electromechanical systems (MEMS), sensors can now fit into smaller spaces without compromising their performance. This has opened doors to new applications, especially in wearable technology and portable devices. To adapt to a wider range of environmental conditions, some new designs incorporate temperature compensation mechanisms, ensuring their reliability.
These innovations not only improve performance but also enable researchers to explore new domains. For example, in food safety and quality monitoring, enhanced capacitance sensors can detect moisture levels and chemical compositions more accurately.
Integration with IoT Systems
The rise of the Internet of Things has significantly influenced the evolution of capacitance detectors. By connecting these sensors to IoT systems, real-time monitoring becomes possible. This integration brings forth several advantages, such as automation and remote data access. In industrial settings, capacitance detectors can be part of larger systems that track production processes, ensuring that standards are maintained.
Moreover, data gathered from capacitance detectors can be analyzed to provide valuable insights. For example, predictive maintenance can be implemented in manufacturing environments, reducing downtime and operational costs. IoT-enabled capacitance sensors can alert operators to irregular capacitance changes that may indicate equipment anomalies.
"The integration of capacitance sensors with IoT technologies marks a significant shift in how data is utilized, allowing for smarter and more efficient operations."
Additionally, the assimilation of capacitance sensors into smart environments means they can interact with other devices. This could enhance capabilities in sectors such as healthcare, where continuous monitoring of patient conditions is vital.
Comparison with Other Types of Detectors
In understanding capacitance detectors, it is essential to compare them with other sensor technologies. Such comparisons shed light on their unique strengths and weaknesses. Capacitance detectors operate on principles distinct from resistive and inductive sensors. By examining these differences, one can appreciate their specific applications and performance metrics.
Resistive versus Capacitive Measurements
Resistive measurements involve detecting changes in resistance due to the material or environmental changes. This technique works best in scenarios where the properties of the material remain stable and predictable. On the other hand, capacitive measurements focus on the relationship between capacitance and electrical charge storage. Factors such as distance between conductive plates and dielectric properties play a significant role here.
Some key differences include:
- Sensitivity: Capacitive sensors often offer higher sensitivity compared to resistive sensors, detecting minute changes in capacitance effectively.
- Response time: Capacitive measurements provide faster response times. They can be particularly advantageous in applications requiring real-time data, such as environmental monitoring.
- Influence of Medium: Resistive detectors can be influenced by impurities in the medium. In contrast, capacitive sensors may show less variability in readings due to external contaminants, provided they are calibrated correctly.
Advantages of Capacitance over Inductive Sensors
Inductive sensors, while effective in certain niche applications, have limitations that capacitance detectors overcome. Inductive sensors work by generating electromagnetic fields to detect changes in inductance. This method can encounter issues with surrounding metal and electromagnetic interference, affecting accuracy.
Several advantages of capacitance detectors over inductive sensors include:
- Non-contact Measurement: Capacitance detectors can measure without needing to touch the object. This method allows for the examination of delicate or hazardous materials without direct interaction.
- Less Susceptible to Noise: Capacitance sensors usually exhibit less interference from environmental variables, making them reliable in fluctuating conditions.
- Wider Applicability: They are suitable for a broader range of applications, from industrial machinery to biomedical devices, thanks to their versatility and reliability.
"Understanding the strengths of capacitance detectors in contrast to resistive and inductive sensors provides a clearer view of their application landscape, enhancing decision-making in technology choices."
Case Studies Utilizing Capacitance Detectors
The exploration of case studies utilizing capacitance detectors reveals their critical role in real-world applications. These studies demonstrate how capacitance technology is applied across various sectors, showcasing both effectiveness and innovative uses. Engaging with these case studies can provide insight into the benefits and challenges tied to capacitance measurements.
Industrial Process Control Enhancements
Capacitance detectors are essential in industrial settings, where precise measurements are paramount. They help in controlling processes such as fluid level detection, material thickness monitoring, and quality assurance.
In industries like petrochemical, for example, capacitance sensors can measure the level of liquids in storage tanks efficiently. The sensors' ability to provide continuous level measurement enhances operational safety and reduces the risk of overflows.
Here are some key aspects to consider:
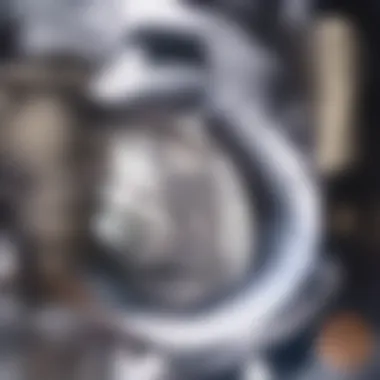
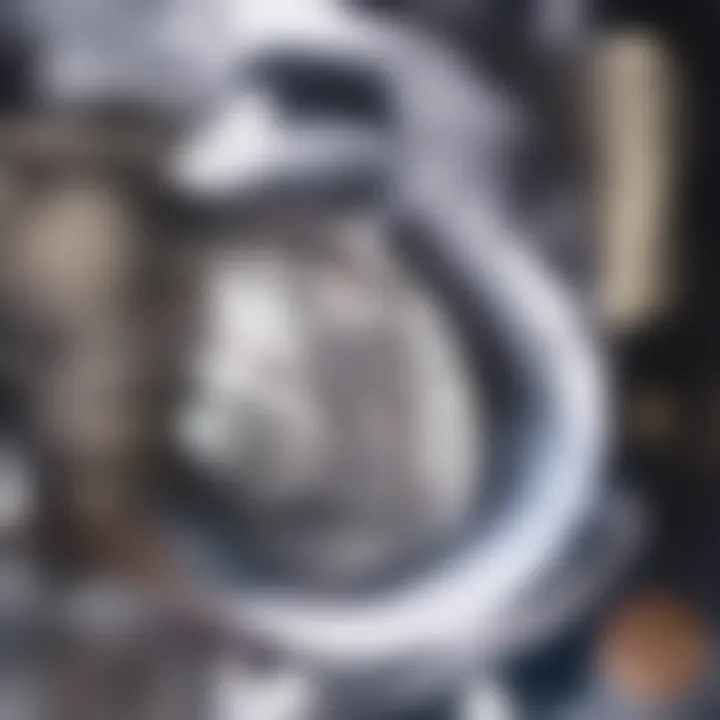
- Reliability: Capacitance detectors offer steady performance even in harsh environments.
- Cost-Efficiency: By improving process control, companies can save significant costs associated with material waste and unsafe conditions.
- Data Integration: These detectors can integrate seamlessly with digital systems, which allows for real-time monitoring and control.
Their usage extends to other industrial applications, refining manufacturing processes, and ensuring consistent product quality. As seen in various case studies, the reliability of capacitance measurement translates into enhanced productivity.
Research Initiatives in Material Science
In the realm of material science, capacitance detectors facilitate detailed analysis and characterization of materials. Researchers utilize these detectors to study dielectric properties, which is crucial for developing new materials. Innovations in polymer and composite materials can be traced back to precise capacitance measurements.
The following points highlight the significance of such case studies:
- Material Characterization: Understanding dielectric constants assists in the development of better insulating materials.
- Testing Under Various Conditions: Capacitance detectors allow researchers to assess material performance under different temperatures and environmental conditions, leading to more robust designs.
- Supporting Theoretical Models: Empirical data collected through capacitance sensors help to validate or challenge theoretical predictions regarding material behavior.
In summary, the application of capacitance detectors in industrial processes and research initiatives demonstrates their versatility and critical importance in advancing technology. By studying practical examples, one can appreciate not only the functionality of these detectors but also their transformative impact across various fields.
Future Trends in Capacitance Detection
As the fields of electronics and measurement science evolve, the role of capacitance detectors adapts to meet new challenges and application needs. Understanding future trends in capacitance detection is essential for researchers and professionals alike. This section will discuss emerging technologies, potential research directions, and the overall implications of such advancements.
Emerging Technologies
The integration of advanced technologies with capacitance detection systems is becoming a more prominent trend. Some key technologies to watch include:
- Miniaturization: Devices are becoming smaller, which enhances portability and ease of integration into various applications. Miniaturized capacitance detectors can monitor capacitance in tight spaces where traditional sensors are impractical.
- Smart Sensors: With the rise of Internet of Things (IoT), capacitance detectors are evolving into smart sensors. These devices are able to collect data and communicate wirelessly, providing real-time monitoring and data analytics. They can be applied in environments such as smart homes and industrial automation.
- Enhanced Materials: The development of advanced materials for sensor fabrication allows for increased sensitivity and better performance under diverse conditions. New composite materials and nanostructures are being explored to improve the properties of capacitance detectors.
These emerging technologies not only provide benefits in accuracy and efficiency, but also expand the potential applications of capacitance detectors beyond traditional boundaries.
Potential Research Directions
Future research in capacitance detection can focus on several promising areas:
- Data Integration: Research should explore the integration of capacitance data with other sensor data. This can enhance the understanding of complex systems by providing a more comprehensive dataset.
- Adaptive Algorithms: Developing algorithms that can adapt based on the measured environment may optimize the performance of capacitance detectors. This can be particularly valuable in variable environments where external conditions fluctuate.
- Durability Testing: As devices become smaller and smarter, rigorous testing for durability and reliability under different operational stresses will be crucial. Research must address long-term stability and resistance to environmental factors.
- Cross-disciplinary Approaches: Engaging perspectives from fields like material science, computer science, and biology can yield innovative uses for capacitance detectors. Such interdisciplinary collaboration can push the boundaries of what is possible in sensor technology.
By advancing these research directions, the full potential of capacitance detectors can be realized, driving better solutions across industries.
"The future of capacitance detection not only lies in improving the sensors themselves but also understanding how they interact with the evolving technological landscape."
The trends in capacitance detection highlight the ongoing transformation and create pathways for sustained innovation. As research continues and new technologies emerge, the landscape for capacitance detection will undoubtedly expand into new domains and applications.
Finale
In summarizing the critical theme of this article, the conclusion serves as a vital synthesis of the information presented regarding capacitance detectors. These devices are integral in various scientific and industrial applications. Their ability to provide accurate measurements of electrical capacitance is essential for numerous fields, including material science, environmental monitoring, and biomedical applications.
Summary of Key Insights
This article has detailed the definition, functionality, and various types of capacitance detectors. Key points include:
- Types of Detectors: Both analog and digital capacitance detectors have unique applications and benefits. Understanding their distinctions is important for effective selection in practice.
- Applications in Industry: There are numerous applications across industrial processes, enhancing efficiency and precision in measurements.
- Technological Advancements: The integration of IoT systems represents a significant leap towards the future of capacitance detection technology. This trend allows for real-time data collection and monitoring, offering unprecedented insights.
The combination of these elements enhances the understanding of capacitance detectors and prepares the grounds for further innovative applications.
Implications for Future Research
The discussion around future trends in capacitance detection technology indicates several pathways for exploration. Specifically:
- Emerging Technologies: New materials and sensor technologies will likely lead to enhanced performance. This includes research into nanomaterials that could improve sensitivity.
- Interdisciplinary Approaches: Collaborations between fields such as engineering, physics, and data science could foster innovative applications of capacitance detectors in new domains.
- Long-term Reliability: Future studies could focus on the long-term effects of environmental conditions on capacitance measurement, addressing the current challenges in calibration and accuracy.
In essence, the conclusion highlights the growing importance of capacitance detectors in various applications while paving the way for future research that could unlock further potential in this field. Their role is undeniably pivotal in pushing the boundaries of current scientific and industrial capabilities.
Citations of Key Studies
In this section, we explore several pivotal studies that have shaped the understanding and development of capacitance detectors. Each study brings unique insights, methods, and findings that contribute to the broader field. The following is a brief overview of several foundational papers:
- C. D. Drazin et al. (1983) showed how environmental conditions affect the precision of capacitance measurements.
- M. J. Kahn (1998) discussed the impact of material properties on capacitance sensing, opening avenues for later research in biomaterials.
- L. H. Chen and R. P. Ranganathan (2010) focused on integrating capacitance sensors with IoT systems, proving essential for modern applications.
These studies greatly enhance the narrative woven throughout this article by grounding it in rigorous academic research.
Recommended Reading
The following texts are excellent resources for anyone interested in delving deeper into the topic of capacitance detectors. They offer a mix of theoretical fundamentals and practical applications. They are listed to enhance understanding and guide further study:
- “Electronic Sensors” by Simon Williams explores the principles behind various sensor technologies, including capacitance-based devices.
- “Capacitance Measurement Techniques” by Rachel Greene offers a comprehensive guide on methods used in capacitance measurement and the associated challenges.
- “Data Collection in Environmental Monitoring” by John Martin discusses the role of sensors in environmental applications, including detailed sections on capacitance sensors.
These texts are invaluable for students, professionals, and anyone eager to expand their knowledge in this technological domain.