Interfering RNA: Mechanisms and Applications in Genomics
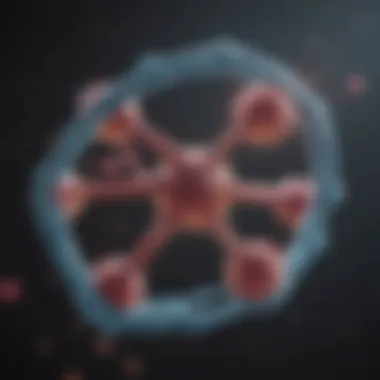
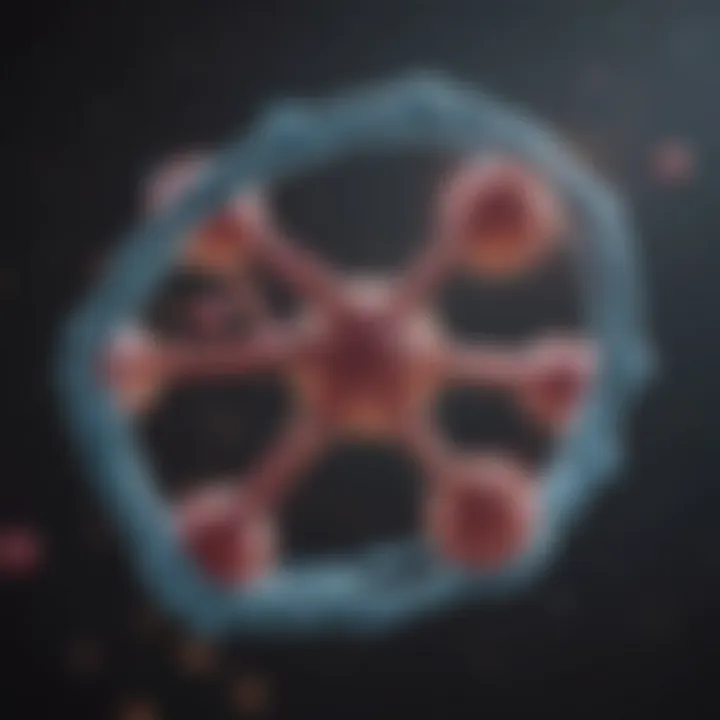
Intro
In recent years, the spotlight has turned towards the fascinating world of interfering RNA, a key player in the regulation of gene expression. With its two main types—small interfering RNA (siRNA) and microRNA (miRNA)—interfering RNA has transformed how scientists approach genomic studies and therapeutics. This tiny but mighty molecule has brought about a paradigm shift in our understanding of genetic regulation and manipulation, showcasing mechanisms that operate on a molecular level, yet hold vast implications for fields such as biotechnology and medicine.
Research Background
Overview of the Scientific Problem Addressed
The dynamic regulation of gene expression is pivotal for maintaining cellular function and response to external stimuli. However, disruptions in these processes can lead to various diseases, including cancer, neurodegenerative disorders, and viral infections. Interfering RNA emerges as a natural solution in the cell's arsenal against these issues, acting as both a safeguard and a potential therapeutic tool. By silencing specific genes, these molecules provide a method to mitigate the harmful effects of aberrant gene expression.
Historical Context and Previous Studies
The journey of interfering RNA began in the early 1990s, when researchers first identified microRNA in the nematode Caenorhabditis elegans. Since then, numerous studies have solidified the role of siRNA and miRNA in gene silencing and regulation. For instance, the discovery of RNA interference (RNAi) as a mechanism to silence genes garnered widespread recognition, earning the Nobel Prize in Physiology or Medicine in 2006 for Andrew Fire and Craig Mello. Their work not only underscored the biological importance of these RNAs but also paved the way for leveraging RNAi in therapeutic applications.
Key Points of Discussion
- The function of siRNA and miRNA in post-transcriptional regulation
- Historical advancements leading to discoveries in RNA biology
- The significant implications for biomedical research and targeted therapies
Findings and Discussion
While the mechanics of RNA interference are robustly documented, the continuous advancements in this field present fresh challenges and opportunities. Current research demonstrates how these interfering RNAs can be manipulated to enhance targeted gene silencing.
Key Results of the Research
Recent advancements have shown that the development of more efficient delivery systems for siRNA and miRNA—such as lipid nanoparticles—has significantly enhanced their therapeutic potential. Additionally, studies have revealed variations in effectiveness, dependent on the specific target, cellular context, and the mode of delivery.
Interpretation of the Findings
The exponential growth of knowledge surrounding interfering RNA points towards its dual nature as both a naturally occurring entity and a therapeutic instrument. On one hand, it presents a sophisticated mechanism that cells utilize to maintain order in gene expression. On the other hand, its applications in therapies offer hope for novel treatments that could specifically eradicate diseases at the genetic level.
"Interfering RNA not only governs cellular regulation but also represents the forefront of genetic therapy innovation."
Understanding these nuances is crucial for researchers and practitioners in the biological sciences, necessitating a continual exploration into the myriad applications and implications of interfering RNA in contemporary genomics.
Intro to Interfering RNA
Interfering RNA has emerged as a groundbreaking area in genomics, essentially acting as a double-edged sword in the regulation of gene expression. Understanding these tiny yet potent molecules is key for anyone diving into the realm of molecular biology. This part of the text sheds light on why interferin RNA deserves close attention, exploring not just its intricate mechanisms but also the far-reaching implications for health and disease management.
Definition and Overview
At its core, interfering RNA comprises small RNA molecules that serve critical roles in gene suppression and regulation. Small Interfering RNA (siRNA) and MicroRNA (miRNA) are arguably the most well-known categories, each exercising control over gene expression through distinct pathways. siRNA typically silences genes in a sequence-specific manner, while miRNA typically regulates gene expression post-transcriptionally by inhibiting translation. Piwi-interacting RNA (piRNA) takes it a step further, primarily involved in regulating germline development and transposon silencing.
The functionality of interfering RNA is likened to a careful gardener: just as a gardener prunes plants to foster growth and remove unwanted elements, these RNA molecules adjust gene expression levels to maintain cellular homeostasis and response to environmental changes. This delicate balance is vitally important in a range of biological processes, from embryonic development to stress responses.
Historical Context
The story of interfering RNA begins in the late 20th century. siRNA was first identified in 1998 by researchers investigating the phenomenon known as RNA interference (RNAi). Their experimentation with petunias revealed that injecting double-stranded RNA could lead to the silencing of specific genes in a way that was unexpected and groundbreaking. This marked the dawn of a new era in genetic research.
In 2001, the discovery of miRNA added a new layer of complexity to our understanding of gene regulation. Found in various organisms, miRNAs provided evidence that not all gene regulation is straightforward. Instead, this layer of regulation can have nuances that impact everything from pocketing cellular resources to responding to stressors.
As these small RNA molecules captured the imagination of scientists worldwide, extensive research ensued, illuminating various aspects of biogenesis, mechanisms, and functions. By the early 2010s, the therapeutic potential of interfering RNA was being explored vigorously, leading to a flurry of innovations focused on delivering these agents to achieve targeted gene regulation, offering hope for treating diseases previously deemed challenging.
Notably, it wasn't just the scientific community that took notice. Clinical applications have practically opened whole new frontiers in personalized medicine, with researchers now developing treatments tailored to individual genetic profiles, marking an exciting time for the field.
Understanding how these RNA agents work—its definition, significance, and historical journey—paves the way for deeper discussions on their multifaceted roles in organisms and their potential applications in modern genetics. This venture into the world of interfering RNA is not simply an academic exercise; it's a gateway to transformative possibilities in health and pathology.
Types of Interfering RNA
Interfering RNA molecules play a fundamental role in the regulation of gene expression, bringing a new dimension to molecular biology. Understanding different types of interfering RNA is crucial because they each have unique mechanisms and applications, providing a rich tapestry for researchers to explore. This section focuses on three prominent types: small interfering RNA (siRNA), microRNA (miRNA), and Piwi-interacting RNA (piRNA). These molecules are not just mere participants in the gene expression saga; they are potent regulators and are harnessed for both therapeutic and experimental purposes.
Small Interfering RNA (siRNA)
Small interfering RNA (siRNA) is perhaps the most well-characterized among the interfering RNA types. These short, double-stranded RNA molecules typically range from 20 to 25 base pairs. Their primary role centers around the process referred to as RNA interference (RNAi), which is critical for silencing specific genes. The design of siRNAs allows them to be complementary to target mRNA, effectively promoting its degradation through the RNA-Induced Silencing Complex (RISC).
In practical terms, researchers often utilize siRNA for gene knockdown experiments in various organisms. For instance, when budding yeast were subjected to siRNA injections, researchers observed a remarkable reduction in the expression of targeted genes, leading to significant insights into gene function and interaction networks. This mechanism serves as a cornerstone in functional genomics, allowing scientists to explore the impact of specific gene loss on cellular processes.
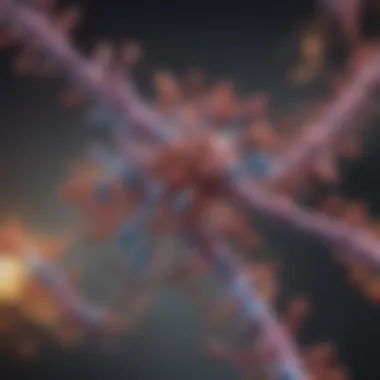
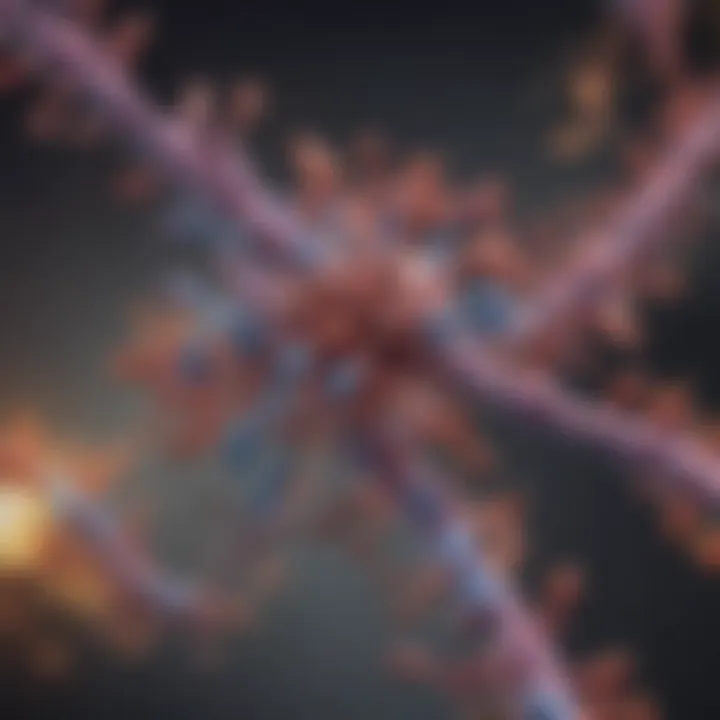
MicroRNA (miRNA)
Conversely, microRNA (miRNA) operates on a slightly different plane. These molecules, approximately 22 nucleotides in length, are crucial players in fine-tuning gene expression. Unlike siRNA, miRNAs are generally derived from longer RNA transcripts that fold into hairpin structures, later processed to yield the active miRNA.
MiRNAs primarily function by binding to the 3' untranslated regions (3' UTR) of target mRNAs, which leads to translational repression rather than degradation. This regulatory function is pivotal in numerous cellular processes such as development, differentiation, and metabolism. A notable example is the role of let-7 miRNA in developmental timing in Caenorhabditis elegans, shaping the organism's growth stages.
Despite their naturally occurring status, miRNAs have been spotlighted in disease mechanisms, including cancer and heart disease. Their ability to regulate multiple genes opens avenues for therapeutic strategies to counteract disease when miRNA expression is dysregulated.
Piwi-interacting RNA (piRNA)
Lastly, we have the Piwi-interacting RNA (piRNA), a class that primarily interacts with Piwi proteins. Distinct in size, piRNAs range from 24 to 30 nucleotides and are integral to germline development. These molecules take on a role in transposon silencing, ensuring the stability of the germline genome during development and preventing the mobilization of transposable elements that could have deleterious effects.
In animals, piRNA pathways provide a safeguard against genetic instability, particularly in reproductive tissues, where the integrity of the genome is paramount. Their involvement marks an essential difference from other small RNAs, serving as guardians of the genome rather than mere regulators of gene expression.
Biogenesis of Interfering RNA
Understanding the biogenesis of interfering RNA is essential for grasping how these molecules function within the intricate web of gene regulation. Biogenesis refers to the biological processes that lead to the formation of these RNA molecules. This topic lays the groundwork for appreciated mechanisms that not only involve their synthesis but also their subsequent roles in various biological processes, from gene silencing to cellular defense systems. The significance of studying biogenesis goes beyond just understanding how these molecules are made; it helps illuminate their potential in therapeutic settings, particularly in combating diseases such as cancer and viral infections.
Transcriptional Origins
The roots of interfering RNA can be traced back to transcriptional origins. This process involves the transcription of specific DNA sequences into RNA. For instance, the transcription of small interference RNA (siRNA) can arise from long double-stranded RNA (dsRNA) precursors, which often originate from viral genomes or structured RNA.
The transcriptional machinery is pivotal. An RNA polymerase enzyme acts on these gene segments, producing primary transcripts that later unfold into different forms of RNA, including miRNA and piRNA. Importantly, the efficiency and accuracy in transcription determine the functional properties of the resultant interfering RNA. The specificity of these sequences ensures targeted action in gene regulation, making transcriptional origins a vital aspect of their biogenesis.
"The transcriptional landscape is not merely the backdrop for RNA production. It's a finely tuned system that dictates the presence and, consequently, the functionality of interfering RNA in the cell."
Dicer and Drosha in Processing
Once the initial RNA transcript is produced, the subsequent processing of this RNA is another critical step in the biogenesis of interfering RNA. Two enzymes, Drosha and Dicer, play key roles in this processing journey.
- Drosha is responsible for the initial stage of processing. It acts in the nucleus, cleaving the primary transcript into smaller precursor molecules, known as precursor miRNA (pre-miRNA). This step is crucial as it ensures that the RNA is appropriately sized for subsequent processing.
- After Drosha's action, the pre-miRNA is exported to the cytoplasm, where Dicer takes over. Dicer further dices the pre-miRNA into mature miRNA or siRNA, depending on the type of interfering RNA being synthesized. This enzymatic activity is indispensable, as it defines the final structure and function of the interfering RNA.
The efficiency and accuracy of Dicer and Drosha are non-negotiable for the effectiveness of RNA interference mechanisms. Mistakes in the processing stages can lead to non-functional molecules, underscoring the importance of these enzymes in maintaining the integrity of gene regulation.
Mechanisms of Action
Understanding the mechanisms of action of interfering RNA is critical for grasping how these small but mighty molecules function in the genetic landscape. They don't just play an incidental role; they are fundamentally involved in the regulation of gene expression. Delving into this subject reveals layers of complexity, highlighting not only how RNA interference (RNAi) operates but also why it matters for both basic research and applied sciences.
RNA-Induced Silencing Complex (RISC)
The RNA-Induced Silencing Complex, commonly referred to as RISC, is the heart of the RNA interference machinery. Once the small interfering RNA (siRNA) or microRNA (miRNA) is generated, it associates with RISC—a multi-protein complex. This amalgamation is not merely a happenstance; it is a highly orchestrated event that initiates a cascade of gene silencing.
RISC is equipped with proteins such as Argonaute, which is crucial for the binding and subsequent degradation of target mRNA. When the guide strand of the siRNA or miRNA is loaded into RISC, it allows for recognition of complementary mRNA sequences. This specificity is paramount; RISC acts like a finely-tuned sniper, ensuring that only the intended targets are silenced, thereby preventing off-target effects that could lead to unintended consequences.
Furthermore, RISC's role extends beyond just targeting and degrading RNA. It facilitates the binding of effector proteins, leading to further post-transcriptional modifications. This dual functionality enhances gene regulation significantly. Without RISC, the promises of gene knockdown and control of gene expression would remain elusive.
Target Recognition and Degradation
The process of target recognition and degradation is a meticulous ballet, requiring precise matching between the guide RNA and its corresponding mRNA target. The accuracy of this interaction is a linchpin of RNA interference. When the guide strand of the RNA binds to its target, it triggers the slicing activity of the Argonaute protein within RISC.
This cutting action is akin to crafting a fine piece of art—where every stroke must be deliberate and on point. The result? The target mRNA is cleaved into smaller fragments, effectively silencing the gene it once expressed. There's a beauty in this mechanism; it allows for the fine-tuning of protein levels within cells, ensuring that only the right quantities are produced at the right times. This attribute makes RNA interference a powerful tool in both research and clinical settings.
"SiRNA and miRNA don’t just inhibit gene expression—they create a sophisticated feedback loop that regulates cellular responses effectively."
Role in Post-Transcriptional Regulation
Post-transcriptional regulation is another key area where interfering RNAs shine. Beyond simply knocking down specific genes, they initiate broad changes in cellular dynamics, affecting the gene expression landscape as a whole. This adds a layer of complexity to our understanding of how genes interact and influence various biological processes.
Through binding with target mRNAs, interfering RNAs can block translation, alter stability, or even recruit decay machinery to eliminate mRNAs before they can be translated into proteins. The end result is a remarkably intricate network of regulatory mechanisms at work. This regulation is vital not just for maintaining homeostasis but also for allowing cells to adapt to environmental changes.
In sum, the mechanisms of action of interfering RNA enable nuanced control over gene expression, impacting everything from individual cellular behavior to overarching physiological processes. The growing list of applications—from functional genomics to therapeutic innovations—underscores the importance of understanding these mechanisms within both fundamental and applied research contexts.
Functions of Interfering RNA
Interfering RNA plays a critical role in numerous biological functions, fundamentally shaping the landscape of gene regulation. Understanding these functions is key for researchers and professionals who aim to harness the power of RNA for various applications, particularly in the realms of genomics and molecular biology. Interfering RNA is integral to cellular processes, influencing not only the expression of genes but also the very survival of the host under various stressors. This section will delve into three primary functions: gene expression regulation, defense against viral infections, and roles in development and cell differentiation.
Gene Expression Regulation
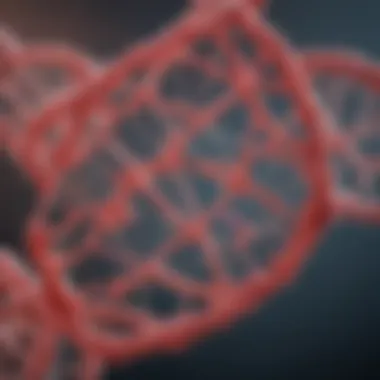
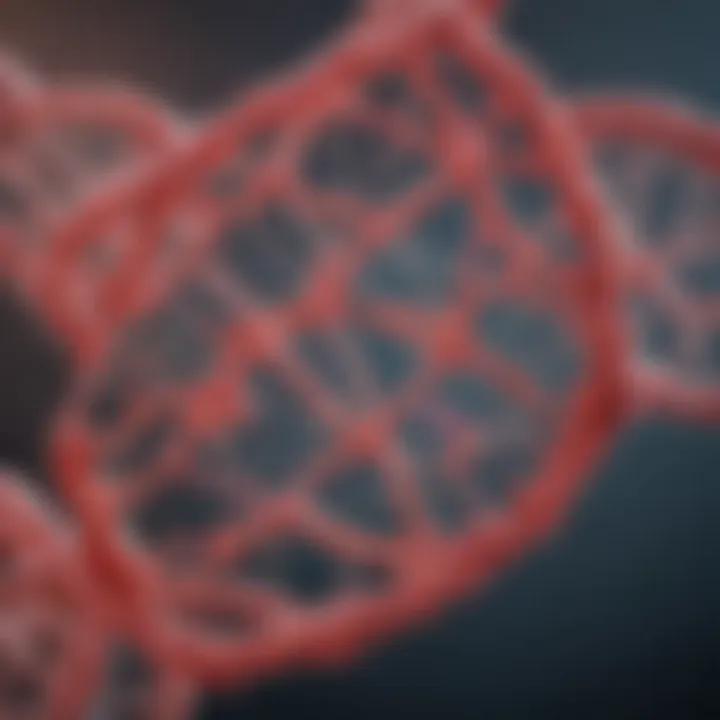
One of the most profound functions of interfering RNA is its ability to regulate gene expression. In this capacity, RNA molecules like small interfering RNA (siRNA) and microRNA (miRNA) bind to messenger RNA (mRNA), either promoting its degradation or inhibiting its translation. This regulation is vital for maintaining cellular homeostasis and responding to environmental changes.
When a cell perceives a signal, whether from external stimuli—such as the presence of a toxin—or from internal changes, the expression of specific genes may need to be adjusted. Interfering RNA steps in here to fine-tune this process. For example, a study highlighted that in plants, miRNAs are crucial for xylem development by targeting genes involved in hormone signaling—showcasing the direct link between gene regulation and physiological adaptation.
Moreover, this gene silencing can be purposefully exploited in research settings. Scientists often utilize siRNAs to knock down genes of interest, enabling a better understanding of their function. By selectively silencing specific genes, researchers can determine the resultant phenotypic effects and interactions within cellular pathways.
Defense Against Viral Infections
Interfering RNA also plays a significant role in the innate immune responses against viral infections. This function is particularly relevant in the context of small interfering RNA (siRNA), which can effectively target viral RNA. In fact, the discovery of RNA interference (RNAi) pathways shifted the focus on how certain small RNA molecules can offer viral defense.
For instance, during a viral attack, cells can generate siRNAs from double-stranded RNA (dsRNA) intermediates produced by viral replication, then load these siRNAs into the RNA-induced silencing complex (RISC). RISC subsequently seeks out complementary viral RNA sequences and initiates degradation. This mechanism serves not only as an immediate line of defense but also highlights a fascinating evolutionary arms race between viruses and their host.
"Interfering RNA is not just a player on the field; it's often the referee, deciding who gets to play in the game of gene expression."
Role in Development and Cell Differentiation
Another intrinsic function of interfering RNA is its role in development and cell differentiation. Variations in the expression of certain genes facilitated by miRNA have been shown to guide critical processes such as stem cell differentiation and tissue formation.
Research indicates that miRNAs can orchestrate complex signaling networks during development. For example, in mammals, specific miRNAs are known to regulate the processes of neurogenesis, influencing the fate of neural progenitor cells. They act to ensure that the right cellular pathways are activated to give rise to various cell types, helping form a functional organism from a single fertilized egg.
In this context, the dysregulation of miRNAs could lead to significant developmental issues or contribute to diseases such as congenital disorders. Therefore, understanding their precise mechanisms becomes vital, offering insights into potential therapeutic applications and interventions in developmental biology.
In summary, the functions of interfering RNA are diverse and far-reaching, touching upon gene regulation, viral defense, and developmental processes. By illuminating these roles, scholars contribute to an ever-expanding vista of genomics, paving the way for innovative applications and therapeutic strategies.
Applications in Research
In the fast-evolving field of molecular biology, the applications of interfering RNA have become increasingly significant. Researchers are exploring these applications to enhance our understanding of gene regulation mechanisms and their intricate roles in various biological processes. The techniques developed around interfering RNA, such as small interfering RNA (siRNA) and microRNA (miRNA), have become foundational pillars in the arsenal of genetic tools. They allow scientists to dissect gene functions and study complex cellular interactions, driving innovations in both fundamental research and therapeutic interventions.
The evolution of gene silencing technologies signifies not only a leap in methodology but also a shift in how we understand and manipulate biological systems. With the ability to selectively silence target genes, researchers can more accurately model diseases, assess gene functions, and validate targets for drug development. Furthermore, conducting these studies opens avenues for precision medicine, where treatments can be tailored to the unique genetic make-up of individuals, promising better outcomes and fewer side effects.
Gene Knockdown Studies
Gene knockdown studies are at the heart of research involving interfering RNA. They enable scientists to reduce the expression of specific genes, which helps in understanding the function and necessity of those genes within a biological context. This approach can break down into several critical aspects:
- Mechanisms of Action: Through gene knockdown, researchers can probe into the physiological roles of genes by observing cellular responses when their expression is inhibited. This can reveal essential pathways and interactions that govern various biological phenomena.
- Pathology Models: By silencing genes associated with particular diseases, scientists can create better models for studying conditions like cancer, diabetes, and other genetic disorders. For instance, by knocking down oncogenes, researchers can investigate tumor behavior and response to therapies.
- Therapeutic Development: Gene knockdown not only aids in basic research but also paves the way for discovering new therapeutic targets. By identifying critical regulatory mechanisms disrupted in diseases, researchers can develop novel treatments targeting specific pathways.
"The power to switch off genes has revolutionized our approach to understanding biology and disease, unlocking doors previously thought impossible to explore."
Functional Genomics
Functional genomics encompasses a broader framework that integrates findings from gene knockdown studies, pushing the envelope on our understanding of the genome’s roles. This field aims to characterize functions of genes and their products in a holistic manner. Key points include:
- High-Throughput Screening: Advances in technology allow researchers to conduct large-scale screens, identifying the function of thousands of genes simultaneously. These screenings employ interfering RNA to systematically knock down genes across various conditions, providing insights into their roles in diverse biological contexts.
- Systems Biology Approaches: With the data obtained from functional genomics, researchers can apply systems biology theories to understand how individual genes contribute to the functioning of biological networks. It gives rise to computational models that predict how changes in gene expression might ripple throughout a biological system.
- Drug Discovery: Functional genomics feeds directly into pharmaceutical research. By identifying genetic targets involved in disease processes, scientists can prioritize drug candidates more effectively, potentially leading to faster and more efficient drug development pipelines.
The integration of interfering RNA applications in research not only enhances our molecular toolkit but also continuously reshapes our understanding of genetics, leading to potential breakthroughs in treating diseases and improving health outcomes.
Therapeutic Implications
Interfering RNA holds vast potential in the therapeutic landscape, paving new pathways in the treatment of various diseases. Its implications stretch far beyond basic scientific research. Here, the usefulness of RNA interference in developing precise therapies will be examined, along with the considerations that come with such advanced techniques.
RNAi-based Therapeutics
RNA interference (RNAi) has gained traction as a method to fine-tune gene expression, and it shows remarkable promise in treating myriad conditions. One significant advantage of RNAi-based therapeutics is the ability to design specific siRNAs or miRNAs tailored to silence specific genes responsible for diseases. For instance, when it comes to cancer, certain oncogenes can be targeted effectively, letting researchers shut down the aggressive growth of cancerous cells.
Moreover, RNAi-based treatments have been explored for their ability to address viral infections. In this scenario, the application of small interfering RNA can help combat viruses like the Hepatitis C virus by targeting viral RNA directly, inhibiting its replication within host cells. This precision is crucial because it minimizes off-target effects and increases the efficacy of treatment.
Further enhancing the application of RNAi-based therapies are recent advancements in delivery systems. Techniques like lipid nanoparticles or exosomes allow for better delivery of RNA molecules, increasing their stability and efficiency in reaching target cells. Potentially, these innovations might allow RNAi to transition from the lab bench directly to the clinic—something that is eagerly awaited in the medical community.
Challenges in Clinical Applications
Despite the groundbreaking advances, RNAi-based therapeutics face significant hurdles on the road to clinical implementation. One of the notable challenges is the delivery of these molecules to target tissues. While certain delivery mechanisms work in controlled environments, translating that success into full-fledged clinical settings remains tricky. For example, systemic delivery of siRNAs can lead to unintended outcomes if they affect non-target tissues, escalating safety concerns.
Another obstacle involves designing the RNA molecules themselves. For instance, the degree of specificity is crucial; if a therapeutic RNA is not entirely specific to the target, it could silence unrelated genes, leading to adverse effects. Furthermore, stability remains a concern because the RNA molecules need a suitable environment to survive before they reach their intended targets.
Regulatory challenges also loom large. The approval processes for new therapies can be lengthy and stringent, imposing a delay on promising treatments that could otherwise benefit patients. Stakeholders in the healthcare industry must navigate the complex balance of innovation, safety, and efficacy to bring these therapies into widespread use.
Whether dealing with cancers or viral infections, the potential of RNAi in therapy is undeniable, but getting these treatments from concept to clinic is a journey fraught with challenges.
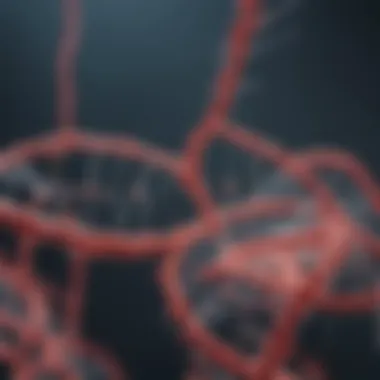
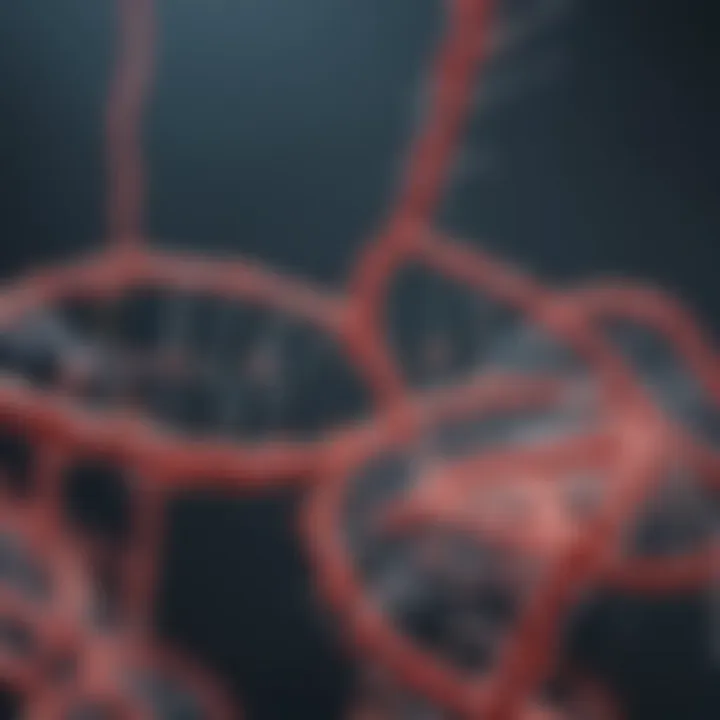
Interfering RNA in Disease Mechanisms
The exploration of interfering RNA within the context of disease mechanisms unlocks a treasure trove of insight into how cellular processes can influence various health conditions. Interfering RNA, prominently siRNA and miRNA, is integral not just in normal physiological regulation, but with aberrant systems that lead to disease. As we peel back the layers of this complex interaction, it becomes clear that understanding these mechanisms offers significant potential for new therapeutic strategies.
The implications extend to understanding pathophysiology, where misregulation of RNA can lead to diseases such as cancer and neurodegenerative disorders. By examining these pathways, researchers may grasp how interventions can be developed that target these small RNA molecules to correct their dysfunctional profiles. This is not just about silencing unwanted genes; it’s about steering the ship towards a healthier state by harnessing the potential of RNA to modulate gene expression effectively.
Cancer Research and Treatment
Cancer, being a multifaceted disease characterized by uncontrolled cellular proliferation, presents a unique battleground for the application of interfering RNA strategies. The relevance of siRNA and miRNA in the context of cancer is staggering, with studies indicating that certain miRNAs can either promote tumorigenesis when overexpressed or act as tumor suppressors when downregulated.
Key aspects in cancer research involving interfering RNA include:
- Targeted Gene Silencing: Using siRNA to disrupt the expression of oncogenes, thereby potentially thwarting cancer progression.
- Biomarker Discovery: Certain miRNAs are being explored as biomarkers for early detection and prognosis, offering a clearer picture of individual disease states.
- Therapeutic Agents: Therapeutics such as MRNA-1273, which leverage these small RNA mechanisms, are ushering in a new era of precision medicine.
The RNAi (RNA interference) mechanism can be exploited in various cancer treatment regimes. For instance, introducing synthetic siRNAs into cancer cells that target specific oncogenes can significantly slow down tumor growth or even enhance the effectiveness of existing therapies. Moreover, certain studies highlight that restoring the function of lost tumor-suppressive miRNAs has shown promise in reducing tumor viability and improving patient survival rates.
Neurodegenerative Disorders
The landscape of neurodegenerative disorders, such as Alzheimer's and Parkinson's disease, is complicated by a web of genetic and environmental factors. Interestingly, the role of interfering RNA in these conditions is gaining traction, as accumulating evidence suggests that dysregulation of miRNAs can contribute to the pathological processes that characterize neurodegeneration.
In the realm of these disorders, the following points underscore the significant role of interfering RNA:
- Regulatory Control: MiRNAs modulate gene expression involved in neuronal health, synaptic plasticity, and neuroinflammation.
- Potential Biomarkers: Specific miRNAs have been identified as potential biomarkers for early diagnosis, offering means for timely interventions.
- Therapeutic Innovations: Research is underway to develop therapeutic strategies aimed at reintroducing or inhibiting specific miRNAs, positing that restoring equilibrium could alleviate symptoms or delay disease progression.
"The manipulation of interfering RNA holds remarkable potential, not only for understanding disease mechanisms but also for pioneering therapeutic strategies that challenge current treatment paradigms."
Thus, as we continue on this investigative path, the nexus between interfering RNA and disease mechanisms presents a promising area of study, potentially transforming how we approach treatment and management of complex diseases. The fusion of genomics and small RNA molecules is poised to reshape therapeutic landscapes, making this an exciting frontier in medical research.
Recent Innovations and Future Directions
As the field of genomics continues its rapid evolution, examining the transformative developments surrounding interfering RNA becomes essential. This section sheds light on advancements that not only enhance our understanding of RNA mechanisms but also pave the way for practical applications in medicine and biotechnology.
Advancements in Delivery Systems
Delivery systems for interfering RNA are pivotal for ensuring effective and targeted application in therapies. The innovative techniques being developed focus on the safe and efficient transfer of RNA molecules into cells. A variety of nanoscale vehicles are employed, including lipid nanoparticles, exosomes, and polymeric micelles.
The approaches aim to overcome barriers like cellular membranes and to minimize degradation by nucleases. For example, recently engineered lipid nanoparticles are showing great promise in delivering mRNA without triggering unwanted immune responses. Additionally, viral vectors are adapted for sustained delivery to specific tissues, crucial for treating chronic conditions.
Here are some notable advancements in this area:
- Targeted RNA Delivery: Advances like antibody-conjugated delivery systems enhance tissue specificity, ensuring that the therapeutic agents reach the intended cells.
- Smart Nanocarriers: These systems release their RNA cargo in response to specific stimuli, such as pH changes, leading to precisely timed therapeutics.
- Guided Therapeutics: Technologies such as CRISPR allow for highly targeted interventions in gene expression, expanding options for cancer treatment and genetic disorders.
The development of these delivery systems signifies a shift toward molecular tailoring that aligns precisely with the needs of patients, showcasing a burgeoning area ripe with potential for further exploration.
Ethical Considerations and Regulatory Challenges
As innovative applications of interfering RNA emerge, ethical implications cannot be set aside. The potential for unintended consequences—such as off-target effects or unforeseen changes in gene expression—raises significant questions. Regulatory bodies must wrestle with how to ensure the safety and efficacy of treatments while keeping pace with rapid advancements in genetic technologies.
Key considerations include:
- Informed Consent: Patients must be fully informed of the potential risks associated with RNA-based treatments, particularly in clinical trials.
- Genetic Privacy: The use of genomic data in developing these therapies necessitates stringent safeguards to protect personal information.
- Equitable Access: As these technologies advance, it's vital to address disparities in access not only among different populations but also between high-income and low-income countries.
Regulatory frameworks must adapt, focusing on flexibility and responsiveness to ensure patient safety and ethical use of RNA interference technologies. Moreover, encouraging collaborative discussions among scientists, ethicists, and regulatory officials will be crucial in navigating the complexities surrounding these innovations.
“Navigating the future of interfering RNA therapies requires balance: innovation must include consideration of ethical boundaries, ensuring safety without stifling progress.”
Culmination
In wrapping up our discussion on interfering RNA, it’s clear that this field holds immense potential in genomics and molecular biology. The mechanisms by which RNA molecules like siRNA and miRNA function have illuminated new pathways in gene regulation, enabling researchers to explore biological processes with unparalleled precision. This article highlights various aspects that underscore the significance of interfering RNA in modern science.
Summary of Key Points
The examination of key points throughout the article reveals several critical factors:
- Diverse Types: Different forms of RNA, such as siRNA, miRNA, and piRNA, each have unique roles in gene silencing and regulation, showcasing the complexity of cellular mechanisms.
- Biogenesis Insights: Understanding the biogenesis of these RNA molecules, specifically through enzymes like Dicer and Drosha, helps clarify their function and significance in RNA interference pathways.
- Mechanisms of Action: The RNA-Induced Silencing Complex (RISC) plays a formidable role in how these RNA types achieve their gene-silencing effects, emphasizing the importance of target recognition.
- Clinical Application: The therapeutic implications discussed highlight not just the promise of RNA-based therapies but also the challenges that come with clinical applicability.
- Future Directions: Innovations in delivery systems and considerations related to ethics and regulation suggest a thriving future for RNA research.
"Interfering RNAs are not just tools for silencing genes; they're changing our fundamental understanding of genetic expression itself."
Final Thoughts on the Potential of Interfering RNA
The promise of interfering RNA is nothing short of revolutionary. As researchers continue to dissect its complexities and find innovative ways to utilize its capabilities, the implications for health and disease management are vast. With increased understanding comes the potential for groundbreaking therapies, providing hope in the realms of cancer treatments and neurodegenerative disorders.
However, navigating the challenges also requires careful thought about ethical standards and regulatory frameworks. It’s essential for the scientific community to strike a balance between innovation and the responsibility that comes with it. The journey of interfering RNA is still unfolding, yet its potential impact is already palpable. These RNA molecules may just redefine the future of genetics and therapeutics.