Comprehensive Techniques for Measuring Thin Film Thickness
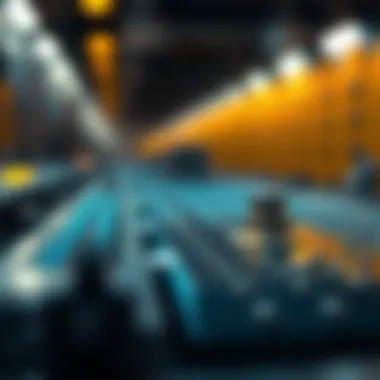
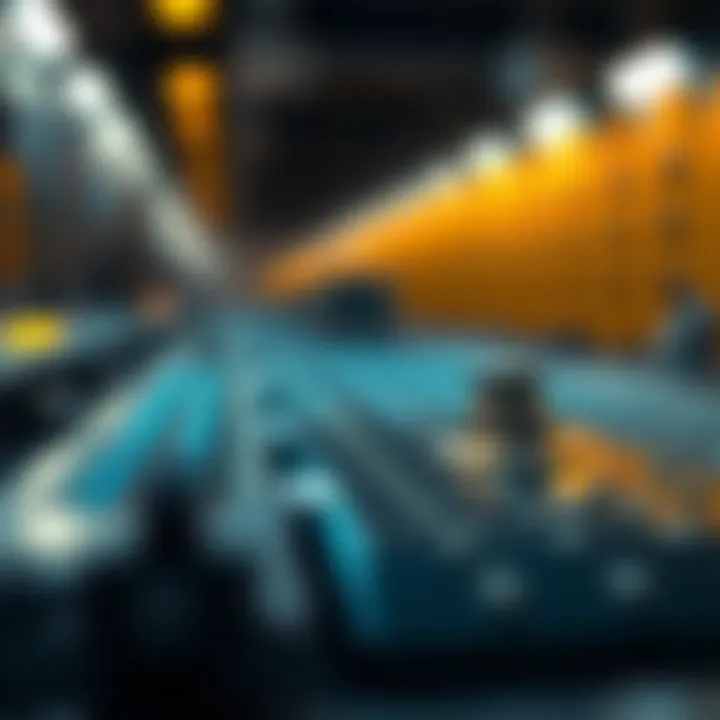
Intro
The measurement of thin film thickness is a nuanced task that plays a pivotal role in various scientific and industrial fields. With the continued advancements in technology and materials science, understanding how to effectively measure these thin layers is crucial. Thin films can range from a few nanometers to several micrometers in thickness, and their properties can differ significantly from bulk materials. In electronics, for instance, thin films are used in semiconductor devices, coatings, and sensors where even slight variations in thickness can lead to noticeable performance differences.
This article aims to dissect several measurement techniques pertinent to thin films, spotlighting the strengths and weaknesses of each. Grasping these nuances will empower researchers and industry professionals alike to optimize their measurement strategies, ultimately leading to advancements in technology and materials performance.
Research Background
Overview of the Scientific Problem Addressed
As industries strive for miniaturization and efficiency, the need for accurate thin film measurements has soared. From solar cells to advanced electronics, precise thickness measurements influence functionality, durability, and manufacturing processes. Without rigorous measurement, the integrity of products may be compromised.
Historical Context and Previous Studies
Historically, the measurement of thin films was a challenging endeavour. Early methods relied on simple mechanical gauges, often leading to inaccuracies due to human error or material inconsistencies. As materials science evolved, so did the techniques. Researchers started to employ optical methods that pivoted from traditional mechanical approaches. Among these, ellipsometry emerged in the 19th century, offering a more precise route to measurement by analyzing the polarization of reflected light.
Studies from the late 20th century set the stage for modern advancements; they highlighted the need for more sophisticated techniques as the demand for nanoscale materials surged. Today's landscape is enriched by innovative methods such as atomic force microscopy (AFM) and X-ray reflectometry (XRR), which have made remarkable contributions to advancing the field.
Intro to Thin Films
The journey into thin films is like peeling an onion; each layer reveals something new and essential to understanding their significance in the modern scientific landscape. Thin films, often measured in nanometers, play pivotal roles in a host of advanced technologies across different industries. From the creation of intricate coatings used in optics to the foundational layers produced in semiconductor devices, their thinness is paramount to their effectiveness. The unique properties that emerge at reduced dimensions can behave quite differently from their bulk counterparts, making it imperative to measure them with precision.
The importance of this segment of the article lies in establishing a framework for understanding what thin films are and why they matter. Delving into this topic offers various benefits: it sharpens awareness of material characteristics, fosters innovation in research applications, and enhances the optimization of manufacturing processes.
When discussing thin films, we need to consider not just their physical thickness, but also how their attributes can change based on that very aspect. For example, the optical interference phenomena observed in thin films pave the way for countless applications in coatings on lenses and mirrors. Thus, the thin film measurement techniques are ultimately about ensuring that these films perform at their best breadth of capabilities.
In essence, knowledge surrounding thin films is not just an academic pursuit; it forms the backbone for advancements that shape our ever-evolving technological landscape. By understanding the core concepts presented in the following sections, readers will be better empowered to appreciate the nuances of thin film thickness measurement, a key aspect in many fields including materials science, electronics, and nanotechnology.
Measurement Techniques Overview
The realm of thin film technology isn’t just abstract; it’s fundamental in numerous applications across materials science and electronics. Whenever one mentions thin films, often the first thought that comes to mind is how their effectiveness hinges significantly on their thickness.
Measurement techniques are like the unsung heroes in this narrative, quietly ensuring that thin films meet required specifications and expectations. As it turns out, the importance of measuring the thickness of thin films cannot be overstated. Precise measurements play a role in determining the performance and reliability of devices ranging from solar panels to computer chips. It’s the bedrock upon which innovations stand—without accurate data, progress is like sailing a ship without a compass.
Category Classification of Methods
When diving into the category classification of measurement methods, it's as clear as day that no single technique reigns supreme. Each method brings its own strengths and weaknesses to the table.
- Optical Techniques: These methods, like interferometry and ellipsometry, capitalize on the way light interacts with materials. They offer non-destructive and rapid measurements, making them quite handy in various settings.
- Mechanical Techniques: Techniques such as nanoindentation tap into the material properties, providing thickness information through force and displacement measurements. This approach is typically more involved, requiring meticulous calibration.
- X-ray Techniques: Unlike the previous methods, X-ray reflectivity focuses on the interaction of X-rays with electron density profiles in materials. It’s highly accurate but also demands more specialized equipment.
Each of these attributes makes the selection of a measurement technique crucial to achieving reliable and reproducible results in thin film applications.
Factors Influencing Measurement Choice
Several factors dictate the choice of a particular measurement technique. It’s a chess game—every move counts, and understanding the pieces involved —that would be the key to success.
- Material Properties: The optical properties of the film can greatly influence which method is best. For instance, transparent films may lend themselves better to optical techniques.
- Desired Accuracy: Some applications require thin film measurements with an accuracy to the nanometer level. In such cases, advanced techniques like X-ray reflectivity may be necessary.
- Cost and Accessibility: Not every lab has deep pockets, so the cost of equipment and the availability of technical expertise must be considered when selecting a measurement method.
- Sample Size and Environment: The size and environment of the sample can also make or break the choice of technique. Certain methods may struggle with thicker or larger films, while others may only work under specific conditions.
"The choice of measurement technique is dictated by an intricate balance of accuracy, budget, and the specific needs of the application."
Understanding these factors can aid researchers and professionals in making informed decisions, thus refining the precision with which thin film measurements are conducted. Through the lens of these considerations, it becomes easier to appreciate the nuance inherent in the selection of appropriate measurement methods for thin films.
Traditional Measurement Approaches
Traditional measurement approaches for thin film thickness have been widely utilized due to their robust foundation in physics and engineering. These techniques are particularly relevant as they offer time-tested solutions to the challenges researchers face in understanding material properties and behaviors. Their methodologies have an entrenched familiarity among scientists and offer reliable data, making them a preferred choice, especially when the thickness range falls within standard scales. Furthermore, traditional approaches often serve as benchmarks against which newer technologies can be compared.
Interferometry Techniques
Basic Principles
Interferometry is fundamentally based on the principle of wave interference. Light or other electromagnetic waves are split, reflected, and recombined, resulting in a pattern of constructive and destructive interference that provides precise thickness measurements. A key characteristic of this principle is its sensitivity to changes in the optical path length, which is pivotal in determining film thickness effectively. This makes interferometry a beneficial choice for researchers who require fine measurements with minimal sample preparation. However, one downside is that environmental factors, such as vibration and temperature fluctuations, can affect the accuracy of the measurements, making controlled conditions crucial.
Types of Interferometers
There are several types of interferometers, each with unique characteristics tailored for specific applications. For instance, the Michelson interferometer is commonly used due to its simplicity and flexibility, whereas the Mach-Zehnder interferometer is favored for its robustness in various conditions. The versatility of these types makes them a popular choice in laboratories looking for varied application scenarios. A unique feature of these devices lies in their ability to provide real-time feedback on film quality and homogeneity. However, some interferometers can be quite costly and require expertise to operate effectively, which could be a hurdle for smaller labs.
Advantages and Limitations
The advantages of traditional interferometric techniques for measuring film thickness are clear: they provide high accuracy and can cover a broad range of thicknesses, from a few nanometers to several micrometers. Moreover, they are non-destructive, allowing for the preservation of the sample. Yet, their limitations should not be overlooked. For example, they can struggle in multilayer systems where complex light interactions occur, leading to difficulties in robust data interpretation. Additionally, the dependency on coherent light sources can sometimes restrict their use in broader applications.
Ellipsometry
Principles of Operation
Ellipsometry operates on a somewhat different principle compared to interferometry. It measures the change in polarization as light reflects off a film surface. The key characteristic of this method is its reliance on the relative phase shift between p-polarized and s-polarized light, providing insights into the film's thickness and optical properties with high sensitivity. This approach is particularly beneficial for thin films because it can measure sub-monolayer thicknesses quite effectively. However, challenging aspects include the need for well-defined optical models to interpret the data accurately.
Parameter Extraction
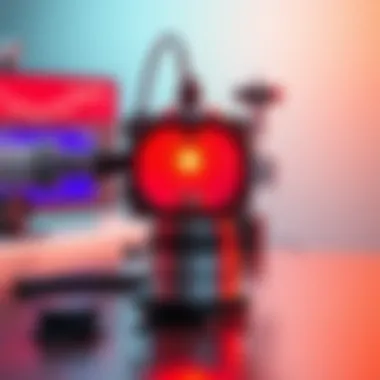
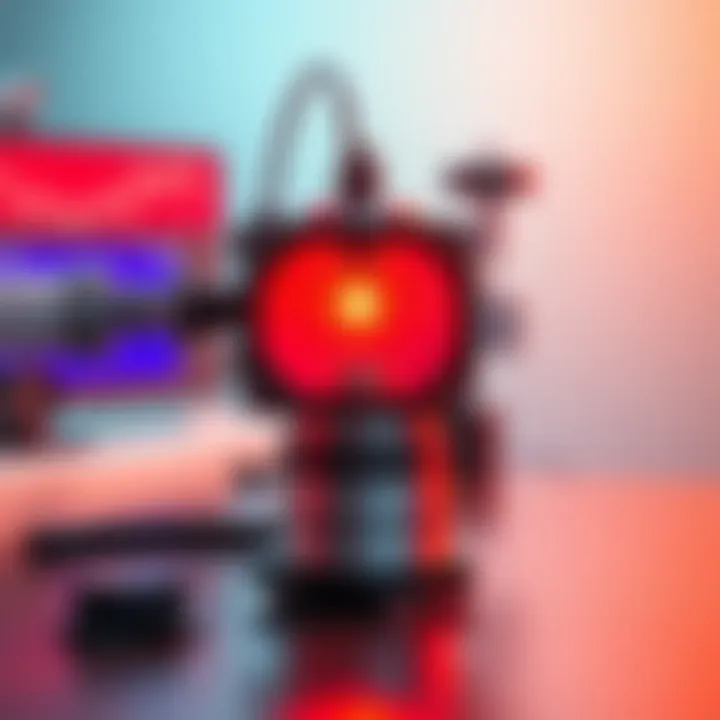
With ellipsometry, extracting parameters like thickness, refractive index, and extinction coefficient is fundamental. The method allows researchers to derive multiple material properties simultaneously from a single measurement, which is advantageous in complex multilayer films. Unique to this technique is its ability to minimize substrate influences, enhancing the accuracy of measurements. Nevertheless, the necessity for sophisticated modeling software and robust mathematical assessments can be seen as drawbacks for teams without the required expertise.
Precision and Accuracy
Precision and accuracy with ellipsometry are outstanding, thanks to the sensitivity of the polarization changes measured. This method typically allows for thickness determinations with deviations as small as a few nanometers. Its robust calibration procedures also ensure high repeatability in measurements. However, the accuracy can be compromised when applied to films with high roughness or when film properties are not uniform. Thus, users must carefully select measurement conditions to secure reliable data.
Spectroscopic Techniques
Spectroscopic techniques play a pivotal role in the realm of thin film thickness measurement, offering precise insights that extend beyond mere thickness evaluation. Their relevance is especially pronounced in applications where thin films must perform under rigorous conditions. These techniques leverage the interaction of light with thin films to extract critical information about their optical properties. This processing can unveil not just thickness, but also refractive index and absorption characteristics, which are vital for various applications such as electronics, optics, and materials science.
Reflectance Spectroscopy
Theory and Functionality
At the heart of reflectance spectroscopy lies the principle that light reflects off surfaces, providing a wealth of information about the material. When a light beam strikes a thin film, a portion of that light reflects back, while the remainder penetrates the film and may reflect off the substrate. This interaction creates interference patterns that can offer insights into the film's thickness. Reflectance spectroscopy is favored because it's non-destructive and can measure thickness with a high degree of accuracy, often in the nanometer range.
Key characteristic: One of its distinct features is the ability to measure multiple layers simultaneously, which is significant in composite materials.
Advantages/Disadvantages: The primary advantage of this technique is its ability to provide real-time data without altering the sample. However, it can be sensitive to the surface condition and environment, which can affect measurement reliability.
Data Interpretation
The interpretation of data obtained from reflectance spectroscopy hinges on analyzing the reflected light spectrum, where shifts in wavelength or intensity reveal information about the film's properties. This aspect is critical as it directly contributes to understanding the performance of thin films in their respective applications.
Key characteristic: The method’s robustness allows it to distinguish subtle changes in refractive index, making it a go-to tool for developers working on advanced materials.
Advantages/Disadvantages: While it provides highly reproducible results, data interpretation may require extensive knowledge of optical properties and modeling, which can be a barrier for less experienced users.
Application Examples
Reflectance spectroscopy finds its niche in various fields, from monitoring coatings on optical filters to assessing the thickness of semiconductor layers in integrated circuits. The versatility of this technique allows users to adapt it to a broad spectrum of materials and contexts.
Key characteristic: Its non-contact nature makes it particularly advantageous in sensitive applications, like those in the semiconductor industry, where physical contact could damage delicate layers.
Advantages/Disadvantages: While the method is widely applicable, it might not perform well with highly absorptive materials, requiring careful selection and preparation of samples.
Transmission Spectroscopy
Principles of Measurement
Transmission spectroscopy operates on the straightforward premise of measuring light that passes through a thin film. When a beam of light is shone through the film, the resulting absorption and transmission spectra provide essential information about thickness and material composition. This method is particularly effective for transparent films where reflectance measurements can be misleading due to surface reflections.
Key characteristic: Its simplicity and direct measurement greatly enhance its appeal in specific applications.
Advantages/Disadvantages: However, while it's relatively straightforward, the technique can become complicated for opaquely absorbing films, where the transmitted light may be minimal, thus complicating the analysis.
Challenges Faced
While transmission spectroscopy is valuable, it is not without its hurdles. One primary challenge is the influence of the substrate on measurements. Variations in substrate material can introduce errors if not properly accounted for. Additionally, environmental factors like ambient light can interfere with obtaining accurate readings.
Key characteristic: Recognizing these challenges is crucial for ensuring accurate results, making careful sample preparation and environmental control fundamental in measurements.
Advantages/Disadvantages: Despite these challenges, the benefits include the ability to analyze films that are transparent and the simple setup. Nonetheless, meticulous attention to experimental conditions is required to avoid significant errors.
Use Cases
In practice, transmission spectroscopy is essential for applications involving optical filters, anti-reflective coatings, and even thin polymer films in packaging. Its ability to provide immediate feedback on film characteristics makes it an invaluable tool in manufacturing settings.
Key characteristic: Particularly useful in research and quality control, this method helps manufacturers quickly ascertain film properties without extensive downtime.
Advantages/Disadvantages: On the downside, the method can be less effective for measuring very thin layers or those with complex multilayer structures, where reflections and interferences might lead to erroneous conclusions.
The sophistication of spectroscopic techniques opens new doors in thin film analysis, streamlining processes and enhancing precision in measurements.
Advanced Measurement Techniques
Thin film thickness measurement is evolving, continuously pushing the boundaries of what is possible in technology and research. Among the many methods available today, advanced measurement techniques bring forth precision, versatility, and efficiency, which are crucial in diverse applications—ranging from electronics to nanotechnology. These methods offer a great depth of understanding, enabling professionals to analyze thin films with remarkable detail. In this section, we will explore two notable techniques: Atomic Force Microscopy (AFM) and Scanning Electron Microscopy (SEM). Each of them holds significance for the accuracy and reliability required in research and industry.
Atomic Force Microscopy (AFM)
Operational Mechanism
Atomic Force Microscopy uses a cantilever with a sharp tip that touches the surface of the thin film. When the tip approaches the material, forces between the tip and the sample generate a deflection, which is precisely measured. This mechanism allows for high-resolution imaging, revealing the topography at the atomic level. Such a unique operational trait makes AFM a favored choice in various fields, including material science. One of its key characteristics is the scanning mode: contact, non-contact, and intermittent contact. While the contact mode provides the most detail, it can risk damaging delicate films. Consequently, the ability to select different modes adds to the flexibility of AFM, making it adaptable to a wide range of samples.
Measurement Capabilities
When it comes to measurement capabilities, AFM stands out by providing detailed insights into surface roughness, mechanical properties, and even electrical conductivity at nanoscale resolutions. This proficiency offers an advantage in research, where understanding the nuances of material behavior is paramount. The key strength of AFM lies in its ability to measure forces and interactions at very small scales. Additionally, researchers can perform studies under ambient or vacuum conditions. However, it does comes with some limitations, such as a slower scan rate compared to other methods, making it less suitable for certain high-throughput applications.
Integration with Thin Film Analysis
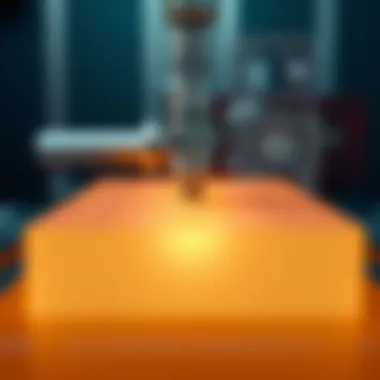
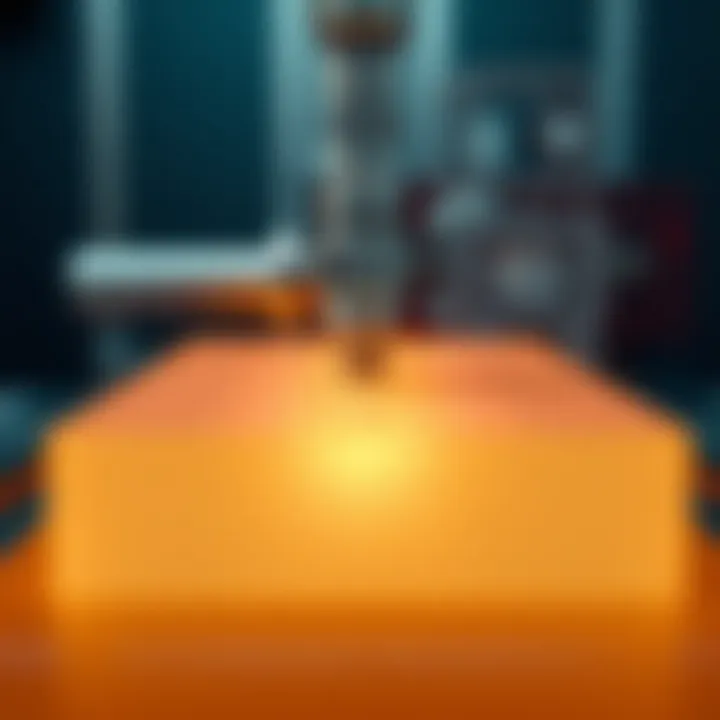
Integrating AFM with thin film analysis leads to a more comprehensive understanding of material properties. It allows for simultaneous observations of topography and other physical characteristics. This integration provides a holistic view of how film thickness influences performance, which is increasingly valuable in developing next-gen materials. One notable feature of this integration is its compatibility with other techniques, such as spectroscopy. While this multi-technique integration enriches the analysis, it may also introduce complexities in data interpretation, potentially complicating the results.
Scanning Electron Microscopy (SEM)
Overview of Techniques
Scanning Electron Microscopy employs a focused beam of electrons to scan the surface of a sample, providing high-resolution images that reveal intricate details of thin films. This method's key characteristic is its ability to attain very high magnifications and depth of field, offering a detailed view of topographic features. The imaging process is relatively straightforward; a detector captures secondary and backscattered electrons emitted from the sample, which helps create a three-dimensional perspective of the surface characteristics. However, SEM requires a vacuum environment, which can sometimes complicate the preparation of samples that are sensitive to atmospheric conditions.
Sample Preparation
Sample preparation is a critical aspect in SEM, as it directly influences the quality of measurements. Generally, it entails coating non-conductive films with a thin layer of conductive material to avoid charging effects during imaging. This step can alter the sample's original properties, which is a key consideration. Proper sample preparation allows for accurate thickness measurement and characterization, making it a necessary yet tricky process. During this phase, researchers must strike a balance between adequate coating and preservation of the sample's integrity.
Image Analysis for Thickness Measurement
Analyzing images captured through SEM is essential for extracting quantitative thickness data. This involves software tools that interpret the contrast and gradation of the film’s layers. One is able to differentiate between various materials present based on how they scatter electrons, providing insights into the film thickness. A defining feature of SEM image analysis is its potential for advanced image processing techniques that can enhance measurement accuracy. Yet, the interpretations rely heavily on user expertise and may require iterative refinement, posing challenges in obtaining unambiguous results.
Nanometer Scale Measurement Techniques
Nanometer scale measurement techniques are central to the field of thin film analysis, particularly when dealing with materials that are only a few nanometers thick. Understanding the techniques at this scale is crucial because as films become thinner, their properties can significantly deviate from bulk materials. The precision needed in these measurements goes beyond mere arithmetic; it requires a tailored approach that accounts for their unique physical and chemical characteristics.
In this section, we delve into two prominent methods—X-Ray Reflectivity and Nanoindentation. Both of these techniques provide vital insights into thin films and help facilitate advancements in various applications, encompassing everything from microelectronics to cutting-edge nanotechnology.
X-Ray Reflectivity
Instrumentation
The instrumentation used in X-Ray Reflectivity is intricate, involving x-ray sources, detectors, and multi-channel analyzers. This combination allows for precise measurements of the film thickness and density, critical parameters that influence the film's performance. One of the key characteristics of X-Ray Reflectivity instrumentation is its ability to produce high-resolution data while ensuring minimal sample damage.
An unique feature of this instrumentation is its capability to non-destructively probe the material, offering a significant advantage over more invasive methods. However, interpreting the data accurately can be challenging and might require sophisticated software, making it a somewhat less accessible choice for newcomers to the field.
Benefits in Thin Film Analysis
The benefits of employing X-Ray Reflectivity in thin film analysis are multi-faceted. It offers unparalleled sensitivity to thickness variations—down to a few nanometers—which is crucial in applications where precision is non-negotiable. Its non-destructive nature and ability to provide depth profiles also position it as a favored technique among researchers.
However, while it excels at measuring thickness, reflected x-ray techniques may struggle with heterogeneous materials where film layers exhibit varying compositions. Balancing these capabilities with the limitations is essential for effective application.
Data Processing Techniques
Data processing techniques associated with X-Ray Reflectivity are central to converting raw data into meaningful insights. These techniques typically involve complex algorithms that help interpret scattering patterns. The sophistication of these processing techniques allows for deconvolution of overlapping peaks, which is essential when analyzing multilayer films.
A notable aspect of data processing in this context is the incorporation of statistical methods to improve measurement accuracy. Yet, the challenge remains that these techniques may require a steep learning curve, especially for those who are newer to the field.
Nanoindentation
Measurement Principles
Nanoindentation measures the hardness and elastic modulus of thin films through controlled-indentation tests, where a sharp indenter penetrates the material's surface. This principle combines mechanical testing with precise depth measurements, offering insights into how thin films will behave under different stress conditions. One key characteristic is its ability to utilize very small-scale forces, making it ideal for materials that are too delicate for traditional indentation tests.
One of the unique features of nanoindentation is its capability to provide immediate results on a range of materials, thus positioning it as a favored approach in rapid research environments. Nevertheless, variations in the indenter design and material can sometimes lead to discrepancies in results.
Material Characterization
When it comes to material characterization, nanoindentation shines by offering detailed information about mechanical properties, which is indispensable in materials science. Understanding how different film compositions will behave mechanically informs decisions about their potential utility in applications, whether electronic or optical. The ability to characterize diverse materials rapidly makes nanoindentation a highly favorable choice.
However, the technique has its limitations; for instance, materials that exhibit viscous behavior may not yield consistent results. Identifying these materials is crucial to obtaining reliable data from measurement.
Limitations and Solutions
The limitations of nanoindentation often stem from the complexity of interpreting the resulting data. Due to the diverse behaviors of materials at the nanoscale, results can vary widely depending on the experimentation parameters. Solutions such as refined indenter tip designs and combinations with other characterization methods can help mitigate these challenges.
Moreover, understanding the limitations enables researchers to better calibrate their tests, leading to improved accuracy in future measurements. Ultimately, adapting techniques and improving methodologies based on observed boundaries is a worthwhile approach to unlocking the full potential of nanoindentation in thin film analysis.
In summary, nanometer scale measurement techniques are indispensable in harnessing the full potential of thin films. Whether it's through X-Ray Reflectivity's non-destructive prowess or Nanoindentation's mechanical insights, each method offers unique advantages and certain limitations that need thoughtful consideration.
Comparative Analysis of Techniques
In the realm of thin film thickness measurement, understanding the different techniques available is paramount. This comparative analysis serves as a bridge between theoretical knowledge and practical application. Each method provides unique benefits and drawbacks, which can significantly impact the selection process depending on the context in which they will be utilized. The importance of this topic lies in its capacity to empower researchers and industry professionals to make well-informed decisions depending on their specific requirements.
The various techniques can be contrasted on several fronts: accuracy and precision, cost and accessibility, and time efficiency. Understanding these factors is not simply an academic exercise; it lays the groundwork for effective experimentation, quality control, and ultimately the success of projects in diverse fields.
Accuracy and Precision
Accuracy and precision are often used interchangeably, but they are distinctly important in the context of measurement accuracy reflects how close a measurement is to the true value, whereas precision relates to how close multiple measurements are to each other. When dealing with thin films, which can be only a few nanometers thick, achieving high accuracy and precision can be particularly challenging.
- Technology Variation: Different measurement techniques exhibit varying levels of accuracy. For instance, ellipsometry usually provides a high degree of precision due to its ability to analyze polarized light. However, environmental factors such as temperature and humidity can affect readings and introduce discrepancies.
- Calibration: Regular calibration of measurement instruments is vital for maintaining accuracy. Instruments that drift over time can yield erroneous results, which could be catastrophic in applications like semiconductor manufacturing, where tolerances are extremely tight.
- Measurement Environment: The surrounding conditions during measurement can also impact results. For instance, X-Ray Reflectivity measurements can be sensitive to sample positioning which can affect results which links accuracy and precision.
Incorporating these considerations into measurement practices allows for the development of metrics that reflect true film thickness with fewer uncertainties.
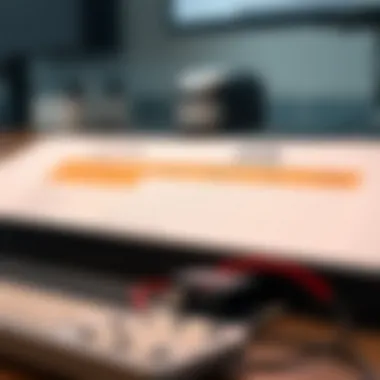
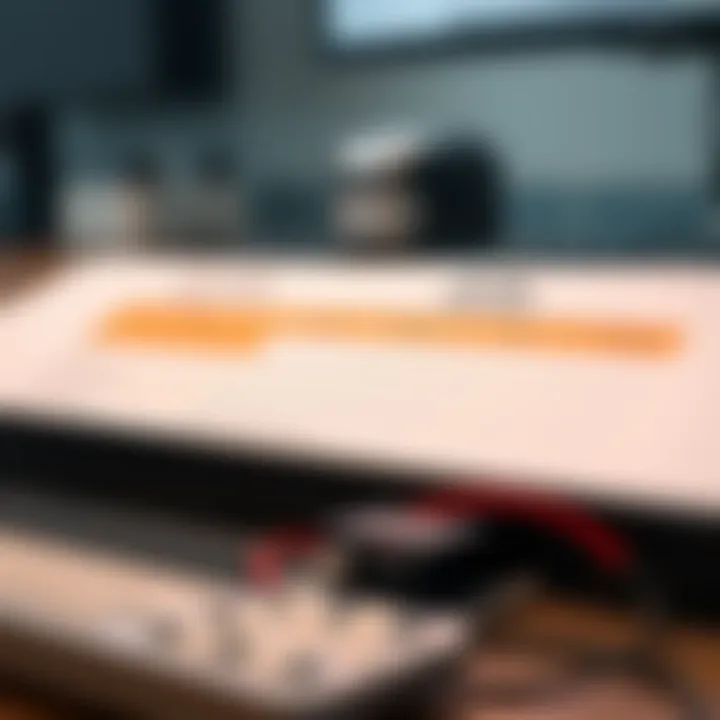
Cost and Accessibility
Cost is another crucial factor when selecting a measurement technique. This can encompass initial investment, maintenance, and operational costs and ultimately affect the feasibility of selecting certain methods, particularly in educational contexts or smaller labs.
- Instrumentation Costs: Advanced systems like Scanning Electron Microscopy often come with a hefty price tag. The initial investment can deter small firms or academic labs from adopting these advanced techniques.
- Running Costs: Some techniques, such as nanoindentation, require specialized environments and may incur additional operational costs from maintenance and support staff, which can quickly add up.
- Accessibility: The skills required to operate complex systems can be a significant barrier. Researchers may need extensive training to effectively use more advanced techniques, which can hinder accessibility for some institutions or regions.
Thus, careful consideration of budget constraints and personnel capabilities is essential when deciding on a suitable measurement technique.
Time Efficiency
The speed at which thickness measurements can be obtained can have substantial implications, particularly in high-throughput environments like semiconductor fabrication or nanofabrication.
- Measurement Speed: Some methods offer rapid measurements. For instance, Reflectance Spectroscopy can provide data in a fraction of the time compared to more complex methods such as Atomic Force Microscopy, which may require detailed sample preparation and scanning.
- Data Processing: Time isn’t just about measurement; it also relates to the time it takes to process the results. Some techniques provide direct readouts, while others, like transmission spectroscopy, may require extensive data analysis to interpret results, slowing down the overall process.
- Workflow Integration: Finally, the time taken to integrate measurement techniques into existing workflows can be a limiting factor. Techniques that require significant changes to workflow can interrupt production schedules and create inefficiencies.
Effective and accurate thickness measurement techniques are the cornerstone of innovation in material science, electronics, and nanotechnology, impacting everything from product development to safety standards.
Practical Applications and Case Studies
Electronics and Semiconductors
In the electronics sector, precision is the name of the game. Thin films are foundational in the production of semiconductors. For instance, when crafting light-emitting diodes (LEDs) or solar cells, the thickness of the semiconductor layer directly affects the device's efficiency. If the layers are too thin, electron mobility suffers, leading to subpar performance. Conversely, excessive thickness can reduce the device's capability to emit light or absorb photons effectively.
Utilizing ellipsometry, engineers are able to measure film thickness down to the atomic scale. This method is preferred because it not only offers accuracy but also provides detailed insights into the layer’s optical properties. A well-documented case is the development of advanced microchips, where tight control over film thickness contributes to improved signal speeds and reduced power consumption.
"In semiconductor applications, a deviation as minor as five nanometers can have significant implications for performance."
Optical Coatings
The realm of optical coatings showcases another critical application for measuring thin film thickness. These coatings enhance product capabilities, especially in devices like eyeglasses, camera lenses, and mirrors. For anti-reflective coatings, the effectiveness is directly influenced by thickness. The design must achieve a specific film thickness to ensure that destructive interference occurs for particular wavelengths of light.
Techniques like reflectance spectroscopy are key to evaluating these coatings. They help to manage quality control in manufacturing processes, ensuring that products meet strict optical standards. For example, in aerospace engineering, precise thin films are employed to create protective coatings on satellite components that must endure harsh environments. Any misstep in measurement could spell disaster for these high-stakes applications.
Nanotechnology Innovations
In the ever-evolving domain of nanotechnology, thin films play a pivotal role. Measuring their thickness is not just a matter of precision but also about understanding material behavior at the nanoscale. A common illustration is in the creation of nanoparticle-based sensors, where the thickness of the sensing layer must facilitate optimal interaction with target molecules for effective detection.
Using techniques such as atomic force microscopy, researchers have pushed the envelope in developing drug delivery systems or wearable sensors where the passive layer's thickness directly influences the system's responsiveness. The case of fabricating nanoscale coatings that improve bacterial resistance in medical devices stands out, demonstrating a tangible benefit to meticulous measurement in thin films. Without effective measurement, such innovations could face developmental roadblocks, slowing advancements in healthcare technology.
In summary, as we journey through practical applications and case studies in thin film thickness measurement, it becomes abundantly clear that precision does not just enhance performance; it is an essential element that determines success across various industries. Embracing innovative measurement techniques opens the door to new possibilities, shaping the way we approach electronic devices, optical products, and cutting-edge nanotechnology.
Future Directions and Innovations
In the rapidly advancing field of thin film technology, the future is as intricate as it is exciting. This section examines the pivotal innovations on the horizon, emphasizing their significance and potential impact within various sectors.
The landscape of thin film thickness measurement is on the brink of massive transformation. Emerging technologies are making it possible to achieve higher precision and speed, alongside reductions in material usage and energy consumption, which are all critical considerations in today’s industry. As the demand grows for thin films in electronics, optics, and nanotechnology, the development of robust measurement tools becomes increasingly vital.
Emerging Technologies
A vast array of new techniques is beginning to reshape the methods used for thickness measurement. Among the notable innovations are:
- Optical Coherence Tomography (OCT): This technique harnesses the principles of light interference to provide high-resolution images of thin films. It enables non-invasive measurement and has shown promise for real-time monitoring.
- Plasmonic Sensors: Capitalizing on the excitation of surface plasmons, these sensors can detect minute changes in thickness by measuring shifts in resonance wavelengths. The precision they offer is a game changer for applications requiring real-time feedback.
- Quantum Dots and Quantum Computing Techniques: As quantum technology evolves, it is paving the way for unprecedented accuracy in thickness measurements, potentially leading to breakthroughs in device performance and material characterization.
- Machine Learning in Data Analysis: The application of machine learning algorithms in processing measurement data can help discern patterns and optimize measurement protocols, leading to quicker and more reliable results.
These advancements do not come without challenges. The integration of new technologies demands rigorous testing, validation, and likely significant investments into research and development to refine these techniques for widespread usage.
Integration of Measurement Techniques
A noteworthy trend is the synergy between different measurement methods. No longer are researchers confined to using one solitary technique; they are combining multiple approaches to gain a comprehensive understanding of thin film properties.
Benefits of Integration include:
- Increased Accuracy: By cross-validating measurements across different techniques, researchers can achieve more reliable thickness estimations.
- Enhanced Interpretation of Results: Different methods provide varied insights. For instance, ellipsometry informs about optical properties while X-ray techniques reveal structural dimensions. Merging these insights paints a more complete picture.
- Cost-Effectiveness: Employing combined methodologies can sometimes circumvent the need for excessive iterations using one singular, possibly costly method.
Despite these advantages, researchers must consider considerations on the practicality of integrating several techniques. Compatibility of equipment, data processing demands, and the expertise required to handle the resultant complexity could hinder immediate application.
"In the realm of thin films, the next big leap is not in a singular, standalone approach but lies in the amalgamation of techniques harnessing their collective strengths."
End
The discourse surrounding thin film thickness measurement holds substantial significance within the realms of materials science, electronics, and nanotechnology. Accurate measurement of these films is not merely a matter of precision; it schemes the boundaries of technological advancement and innovation. This article offers an in-depth exploration of diverse measurement techniques, each with unique advantages and limitations. By understanding these subtleties, researchers and industry professionals can make informed choices tailored to their specific needs.
One salient aspect emphasized throughout the article is the interplay between technique selection and application requirements. The choice of measurement method can influence outcomes in various applications, from optical coating design to semiconductor fabrication. For instance, while ellipsometry provides high precision and is well suited for optical properties, basic interferometry may still hold precedence in certain cost-sensitive applications. Therefore, weighing technical benefits against budgetary constraints remains a critical undertaking.
Furthermore, the advancement and integration of new technologies herald exciting possibilities for the future of thin film measurement. Techniques such as atomic force microscopy and nanoindentation not only refine measurement capabilities but also open pathways for new applications. In a world that increasingly demands higher precision with reduced costs, familiarity with these evolving methods is crucial.
"The future of thin film analysis resides in a balance between innovative measurement approaches and the practical aspects of implementation."
Conclusively, the findings of this article lay the groundwork for a deeper appreciation of thin film measurement methodologies. As technology progressively wades through the terrain of smaller dimensions and enhanced functionality, the importance of adhering to rigorous measurement standards cannot be overstated. Each measurement technique discussed entails a practical nuance, inviting ongoing exploration and multidisciplinary collaboration.
Summary of Key Findings
- Diverse techniques ranging from traditional to advanced, each suited for specific applications.
- Importance of understanding the operating principles for effective selection of measurement methods.
- Emerging technologies are reshaping the landscape of thin film analysis.
Recommendations for Further Reading
- Thin Film Phenomena by W. D. Callister, Jr. - A foundational text that explores the principles of thin films in materials science.
- Fundamentals of Photonics by B. E. A. Saleh and M. C. Teich - Offers insights into photonic measurement techniques used in thin film technology.
- Online resources like Wikipedia on Thin Films can provide quick references for basic concepts and phenomena.
- Peer-reviewed journal articles available on JSTOR or ScienceDirect delve deeper into specific case studies and recent advancements.