The Multifaceted Role of Hydrogen in Modern Science
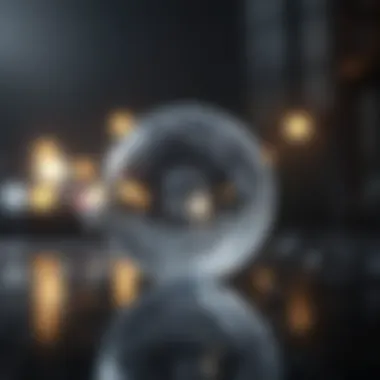
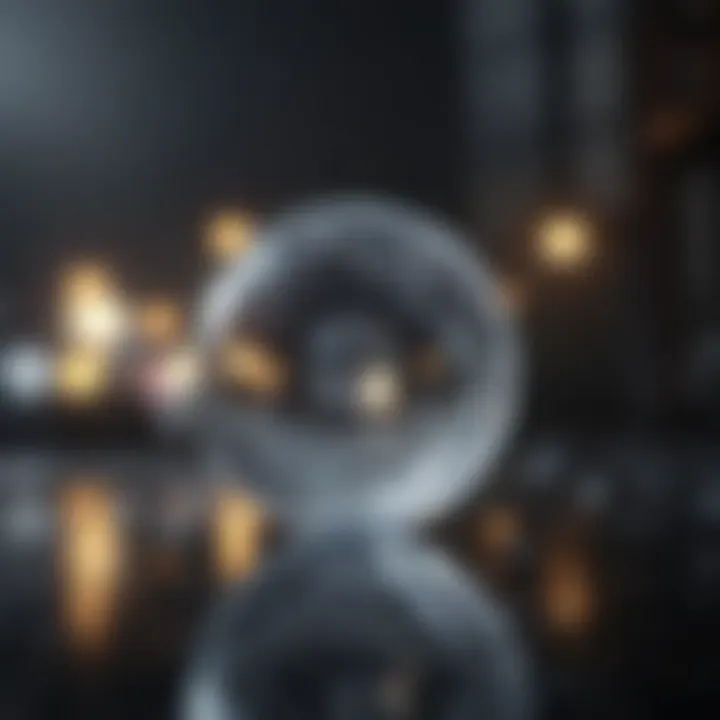
Intro
Hydrogen is often seen as a simple element, but its influence in modern science is extensive and multifaceted. This makes exploring its role essential for those interested in chemistry, energy, and environmental science. Understanding hydrogen, its properties, and applications leads to insights into current scientific challenges and developing solutions that can change how we address energy needs and environmental issues.
Research Background
Overview of the Scientific Problem Addressed
Climate change and energy security are pressing global challenges. Reliance on fossil fuels has resulted in significant greenhouse gas emissions, contributing to environmental degradation. Alternatives are necessary to create sustainable energy solutions. Hydrogen presents an opportunity, offering both clean energy production and potential reductions in CO2 emissions. However, the transition to hydrogen as a primary energy source necessitates significant research and innovation, including production, storage, and distribution methods.
Historical Context and Previous Studies
Historically, hydrogen has been recognized for its unique properties. Its discovery dates back to the late 18th century when Henry Cavendish identified flammable gas, leading to deeper exploration of its chemical behavior. The 20th century witnessed various studies focusing on hydrogen's role in fuel cells and its potential for energy storage. In recent years, significant advancements in technology have reignited interest in hydrogen, particularly in terms of renewable energy integration. The shift towards green hydrogen, produced through electrolysis using renewable energy sources, marks a pivotal point in its development.
Findings and Discussion
Key Results of the Research
- Production Methods: Research highlights several hydrogen production methods, including steam methane reforming, electrolysis, and biomass gasification. Each method has implications for sustainability and efficiency.
- Storage Solutions: Innovations in hydrogen storage, such as high-pressure tanks and cryogenic systems, are crucial for overcoming logistical challenges in hydrogen utilization.
- Industrial Applications: Industries are beginning to integrate hydrogen into processes, notably in refining, ammonia production, and steel manufacturing. These applications show significant potential for reducing carbon footprints.
Interpretation of the Findings
The findings suggest that hydrogen is more than just a fuel source; it is a key player in achieving net-zero emissions. However, barriers still exist, including cost-effectiveness and infrastructure development. As noted in various studies, overcoming these challenges will require collaboration between governmental, academic, and industrial sectors, fostering an environment where hydrogen technologies can flourish.
"The switch to hydrogen energy is not merely an option; it is becoming an essential pathway for sustainable development in energy systems."
Through systematic study and analysis, the research points toward a future where hydrogen plays a critical role in both energy production and storage, with the potential to drive innovation across various industries.
Prelude to Hydrogen
Hydrogen is a fundamental element that plays a critical role in various scientific fields. Its simplistic structure and unique properties make it essential for a multitude of applications ranging from energy production to industrial processes. Understanding hydrogen encompasses not just its chemical nature but also its significance in promoting sustainability and advancing technology.
The study of hydrogen starts with its molecular composition. As the lightest and most abundant element in the universe, hydrogen is often referred to as the building block of matter. It has the ability to combine with other elements, making it indispensable in forming complex compounds. This article aims to provide comprehensive insights into hydrogen, emphasizing its historical context and current applications.
"Hydrogen is a key player in the journey towards a sustainable future, influencing diverse sectors like energy, aerospace, and automotive industries."
Historical Discovery
The discovery of hydrogen dates back to the late 18th century. The term 'hydrogen' was coined by the French chemist Antoine Lavoisier in 1783. He recognized hydrogen as a distinct element produced from the reaction of acids with metals. However, the journey to understand hydrogen's properties started earlier with scientists like Henry Cavendish, who first identified hydrogen as a unique gas in 1766. His experiments showcased hydrogen's flammability and how it produces water when burned in air.
These discoveries laid the groundwork for modern chemistry. Over time, researchers built upon this foundational knowledge. The work of John Dalton and Dmitri Mendeleev further advanced our understanding of hydrogen and its relationship with other elements. Ultimately, hydrogen's role in chemical bonding and reactions became pivotal in the study of chemistry.
Chemical Properties
Hydrogen is characterized by its simple atomic structure, consisting of only one proton and one electron. This simplicity belies its versatile reactivity. As the least dense gas, hydrogen is colorless, odorless, and tasteless. It readily forms bonds with other elements, which is critical in various chemical reactions.
In its molecular form (H₂), hydrogen exhibits unique properties:
- Reactivity: Hydrogen can react with many elements and compounds, contributing to the formation of acids, bases, and other substances.
- Energy content: Hydrogen yields a high energy output when combusted, making it an attractive fuel alternative.
- Isotopes: Hydrogen has three isotopes: protium, deuterium, and tritium. Each has distinct properties and applications, especially in nuclear reactions and research.
Understanding these chemical properties is vital for leveraging hydrogen in practical applications, such as fuel cells and industrial synthesis. This foundation supports the ongoing research into hydrogen’s role in addressing global energy challenges.
Hydrogen in Nature
Hydrogen, the simplest and most abundant element in the universe, plays a crucial role in various natural processes and phenomena. Understanding its presence and function in nature provides valuable insights into both the environmental landscape and broader scientific implications. It is fundamental to the formation of stars, the structure of galaxies, and the chemistry of life itself. This highlights the significance of hydrogen not only in scientific exploration but also in practical applications that impact our daily lives and ecosystems.
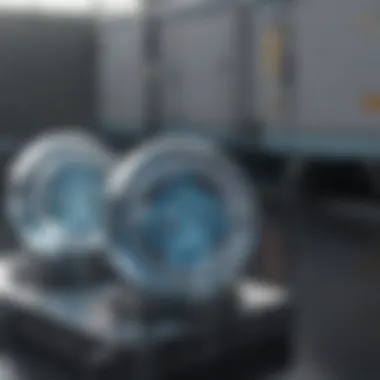
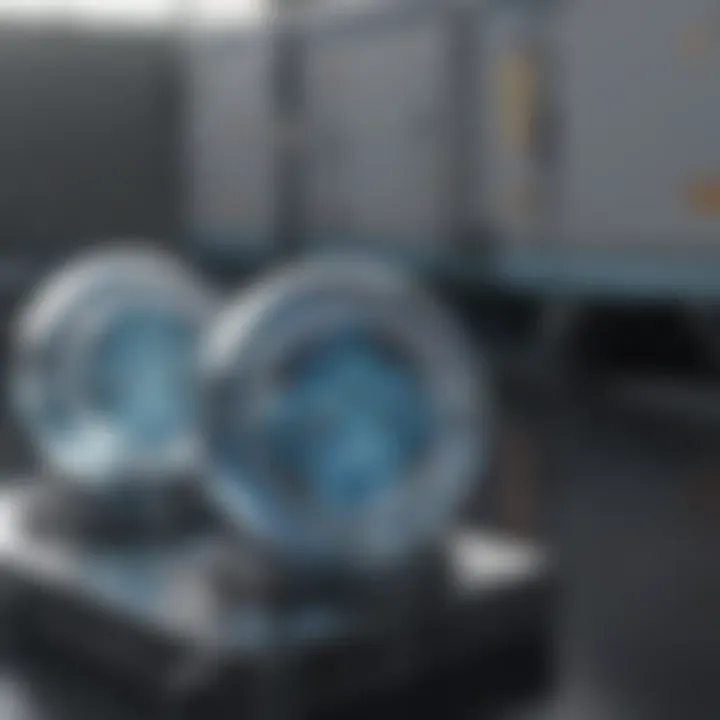
Distribution in the Universe
Hydrogen accounts for nearly 75% of the elemental mass of the universe. It is primarily found in stars, where it undergoes nuclear fusion, forming helium and releasing tremendous energy. The prominence of hydrogen means it serves as the essential building block for more complex elements through nucleosynthesis. This process generates energy that fuels the stars' luminosity and ultimately influences galactic behavior. Furthermore, clouds of hydrogen gas in space, known as molecular clouds, act as the breeding ground for new star formation. Thus, hydrogen's distribution in the universe impacts both stellar life cycles and cosmic evolution.
Abundance on Earth
On Earth, hydrogen is abundant in various compounds, most notably water, which comprises about 71% of the planet's surface. This element exists in hydrocarbons, acids, and as part of biological molecules, including proteins and carbohydrates. Hydrogen plays a core role in maintaining the balance of biogeochemical cycles. For instance, in the water cycle, hydrogen atoms transition between different states, facilitating the processes of evaporation, condensation, and precipitation. Moreover, hydrogen's presence in organic matter makes it vital for many life forms. Its abundant availability and its role in numerous chemical reactions emphasize its importance in sustaining life and shaping Earth’s geology.
Hydrogen is not just an element; it is integral to the processes that sustain and govern our planet's systems.
Hydrogen Production Methods
The production of hydrogen is a cornerstone for its increasing applications in modern science and technology. It serves multiple purposes, ranging from energy generation to industrial processes. Understanding the various methods for hydrogen production is essential due to the implications they have on efficiency, sustainability, and economic factors. This section will detail three primary methods for producing hydrogen: electrolysis, steam methane reforming, and biological production. Each method has its specific advantages and challenges, influencing the broader adoption of hydrogen technologies.
Electrolysis
Electrolysis is a pivotal method for hydrogen production, where water is split into hydrogen and oxygen using an electric current. This process can be achieved through different types of electrolysis technologies, such as alkaline electrolysis and proton exchange membrane (PEM) electrolysis. The significance of electrolysis lies in its ability to produce hydrogen from renewable energy sources. When powered by solar or wind energy, electrolysis can offer a clean and sustainable method for hydrogen generation.
One notable benefit of electrolysis is its flexibility. Electrolyzers can be scaled to suit small or large applications, adapting to varying electricity supplies and demands. However, the efficiency of electrolysis can be impacted by several factors including the cost of electricity and the technology used. As renewable energy becomes more economical and accessible, the prospects for large-scale adoption of electrolysis increase significantly.
Steam Methane Reforming
Steam methane reforming (SMR) is currently the most common method for hydrogen production, accounting for a large percentage of global hydrogen supply. In this process, methane—typically extracted from natural gas—is reacted with steam under high temperatures to produce hydrogen and carbon dioxide. While SMR is efficient and cost-effective, it is also criticized for its carbon emissions.
The importance of steam methane reforming stems from its established infrastructure and lower production cost compared to other methods. However, this method raises concerns about sustainability and its environmental impact. As the focus shifts towards cleaner energy solutions, there is an urgency to develop carbon capture and storage technologies alongside SMR, to mitigate the associated emissions.
Biological Production
Biological production of hydrogen refers to methods that utilize living organisms to produce hydrogen. This includes fermentative hydrogen production from organic substrates and photobiological methods where algae or bacteria harness sunlight to generate hydrogen through photosynthesis. The potential of biological production is still being investigated, but it carries the promise of low energy input and a high degree of sustainability.
The relevance of biological production lies in its environmental benefits and the capacity to convert waste materials into hydrogen. Although the current methods tend to have lower hydrogen yields compared to electrolysis and SMR, ongoing research aims to enhance the efficiency and productivity of biological systems. This could transform biological production into a viable option for renewable hydrogen generation in the future.
"Hydrogen production methods are essential not only for energy transition but also for developing sustainable practices in diverse industries."
In summary, exploring various hydrogen production methods is crucial in understanding the multifaceted role of hydrogen in science and technology. Each method offers unique benefits and challenges, shaping the future of hydrogen as a key energy carrier.
Hydrogen as an Energy Carrier
Hydrogen's role as an energy carrier is pivotal in the transition from fossil fuels to cleaner energy sources. Utilizing hydrogen as an energy carrier allows for the storage and transport of energy, making it a valuable asset in efforts to combat climate change. Hydrogen can be generated from diverse energy sources and subsequently used across various applications. Its versatility makes it essential for numerous fields, including transport, heating, and electricity generation.
Fuel Cells
Fuel cells represent a primary mechanism through which hydrogen can be harnessed as an energy carrier. A fuel cell converts the chemical energy from hydrogen into electricity through a reaction with oxygen. The byproducts of this reaction are water and heat, which are far less harmful to the environment compared to emissions from traditional combustion engines.
Fuel cells offer several benefits:
- Efficiency: Fuel cells are generally more efficient than conventional internal combustion engines. They can convert up to 60% of the energy in hydrogen into usable electricity, compared to around 20% for gasoline engines.
- Low Emissions: Only water vapor is produced when hydrogen is used, which significantly reduces air pollution.
- Scalability: Fuel cell systems can be scaled to suit various power requirements, from small portable devices to large stationary applications.
The automotive sector has notable potential for fuel cell applications, as seen with manufacturers like Toyota and Honda, both investing heavily in fuel cell technology. This adaptation could lead to cleaner transportation solutions that contribute positively to environmental sustainability.
Hydrogen Combustion
Hydrogen combustion is another prominent application of hydrogen as an energy carrier. Similar to traditional fuels, hydrogen can be combusted to produce energy. However, unlike fossil fuels, burning hydrogen produces only water vapor and no carbon dioxide. This characteristic positions hydrogen combustion as a sustainable alternative in certain sectors.
Some important aspects include:
- Direct Usage: Hydrogen can be used directly in modified internal combustion engines. This can facilitate a smoother transition from traditional fuels without the need for entirely new technologies.
- Rapid Energy Release: The combustion of hydrogen releases energy quickly, making it suitable for applications that require rapid power output, like certain industrial operations.
- Infrastructure Compatibility: Existing natural gas infrastructure could be adapted to transport hydrogen, reducing initial costs and time required for broader adoption.
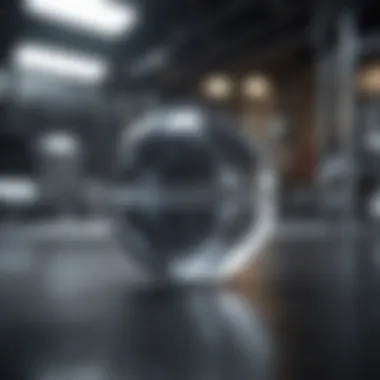
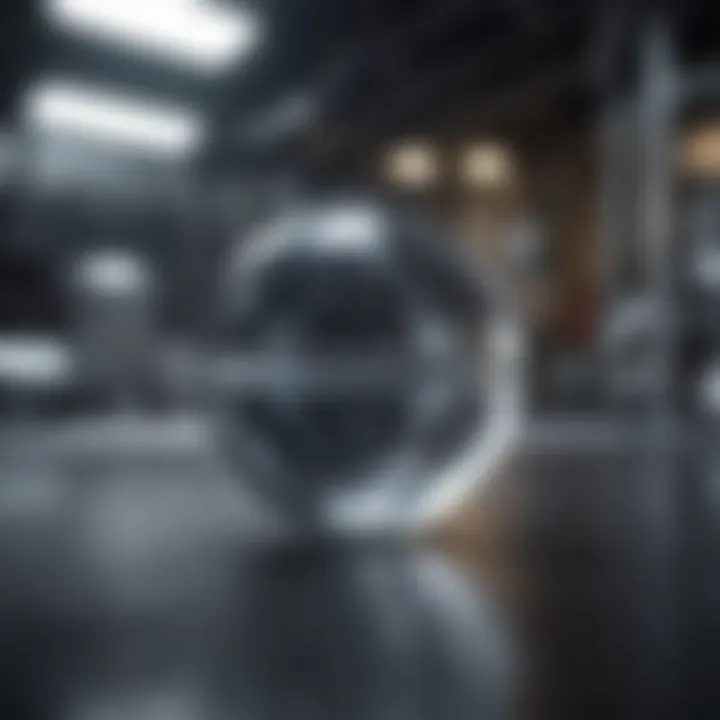
In summary, both fuel cells and hydrogen combustion illustrate the diverse applications of hydrogen as an energy carrier. The push for research and development in these areas remains crucial for enhancing hydrogen's role in a sustainable energy future.
Hydrogen offers a unique opportunity to address energy needs while significantly reducing environmental impacts. Embracing its utilization is essential in today's world.
Environmental Impact of Hydrogen
The discussion surrounding the environmental impact of hydrogen is critical within the larger context of modern science. As the world shifts towards sustainability, understanding the role of hydrogen becomes increasingly important. Hydrogen can mitigate some of the most pressing environmental issues we face today, primarily those related to climate change and air pollution. Its utilization in various sectors can lead to significant reductions in greenhouse gas emissions, thus contributing positively to our ecological footprint. However, it is essential to address the associated challenges and potential shortcomings that arise from hydrogen production and usage.
Carbon Footprint Analysis
Conducting a carbon footprint analysis of hydrogen involves evaluating the emissions generated at different stages of its lifecycle. This includes production, storage, transport, and actual consumption. The type of hydrogen being produced, whether it is green, blue, or grey, significantly influences its overall carbon emissions.
- Grey Hydrogen: This is produced from natural gas or methane through steam methane reforming. It generates substantial carbon dioxide emissions.
- Blue Hydrogen: This also comes from natural gas but employs carbon capture and storage technologies that reduce emissions. While it is cleaner than grey hydrogen, it is not emission-free.
- Green Hydrogen: This is produced via electrolysis, using renewable energy sources such as wind and solar power. Its lifecycle emissions are minimal, making it a more sustainable option in terms of environmental impact.
The comprehensive analysis indicates that shifting to green hydrogen could lower overall carbon emissions significantly when compared to fossil fuels. By investing in green hydrogen technologies, industries can reduce their environmental footprint and reliance on conventional energy sources.
Comparison with Fossil Fuels
When comparing hydrogen to fossil fuels, hydrogen exhibits several noteworthy advantages in terms of sustainability and environmental impact. Fossil fuels, such as coal, oil, and natural gas, are major contributors to air pollutants and greenhouse gas emissions, which are detrimental to human health and the environment.
- Emissions: Burning fossil fuels releases carbon dioxide, nitrogen oxides, and particulate matter, worsening air quality. In contrast, hydrogen fuel cells produce only water vapor as a byproduct when harnessed correctly, contributing to cleaner air.
- Resource Availability: Fossil fuels are finite resources. As they become scarcer, prices are likely to rise. Hydrogen, on the other hand, is the most abundant element in the universe, offering a near-limitless supply when produced sustainably.
- Energy Efficiency: Hydrogen can offer higher energy densities compared to conventional fossil fuels, leading to improved efficiency in energy systems.
However, it must be noted that hydrogen production is not entirely without environmental challenges, especially in terms of renewable energy use for electrolysis. Ideally, advancements in hydrogen production technologies will further minimize the environmental disruption associated with its use.
In summary, while hydrogen holds the promise of a cleaner future, the methods of its production and consumption must be continuously examined to maximize environmental benefits and minimize adverse effects.
Applications of Hydrogen
The applications of hydrogen extend across various industries, showcasing its versatility and relevance in modern science. This section examines its use in key sectors, emphasizing the unique benefits and considerations associated with each application. The understanding of these applications is crucial as they underpin hydrogen's role in advancing technology and promoting sustainable practices.
Aerospace Industry
In the aerospace sector, hydrogen is a vital component for both propulsion and energy storage. The primary application is in fuel cells, which provide power for spacecraft and unmanned aerial vehicles (UAVs). These fuel cells convert hydrogen and oxygen into electricity, offering a cleaner option compared to traditional fuels. The importance of hydrogen in this context stems from:
- Lightweight Properties: Hydrogen has a high energy-to-weight ratio, making it suitable for aerospace applications where reducing mass is essential.
- Environmental Benefits: Using hydrogen as a fuel emits only water vapor, significantly diminishing the carbon footprint of aviation.
- Long-duration Missions: Hydrogen fuel cells can supply sustained power, which is critical for long-duration space explorations or high-altitude flights.
Despite the benefits, challenges persist, including the need for robust storage solutions and safety protocols during launch and operation.
Automotive Sector
Hydrogen's role in the automotive sector is gaining traction, particularly in the development of hydrogen fuel cell vehicles (FCVs). These vehicles utilize hydrogen rather than gasoline, which has substantial advantages:
- Zero Emissions: FCVs emit no harmful pollutants, making them an attractive alternative to traditional internal combustion engines.
- Faster Refueling: Refueling a hydrogen vehicle can be comparable to filling up a gasoline car, which is a significant improvement over the charging times of battery electric vehicles.
- Long Range: Hydrogen vehicles often offer greater range than their electric counterparts, addressing a key limitation for consumers.
However, the automotive sector faces hurdles such as the limited availability of hydrogen refueling stations and the cost of hydrogen production, which impacts consumer adoption.
Chemical Manufacturing
In chemical manufacturing, hydrogen serves as a feedstock in the production of various chemicals. It is essential for processes such as:
- Ammonia Production: Hydrogen plays a critical role in synthesizing ammonia through the Haber process, which is fundamental for fertilizers and agriculture.
- Petrochemical Refining: Hydrogen is used to remove sulfur and other impurities, thereby improving the quality of fuels.
- Hydrogenation Processes: Many organic compounds undergo hydrogenation, which modifies their chemical structure to enhance properties like stability and reactivity.
The demand for hydrogen in this sector underscores the importance of sustainable production methods, particularly as regulations on emissions tighten globally.
Hydrogen is becoming a cornerstone of the green transition across industries, enabling cleaner processes and products.
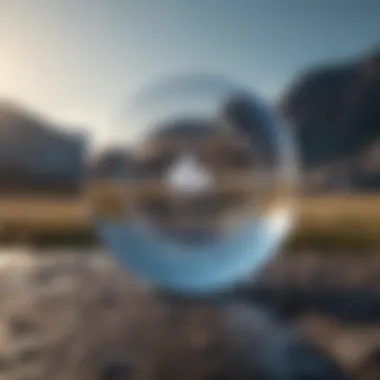
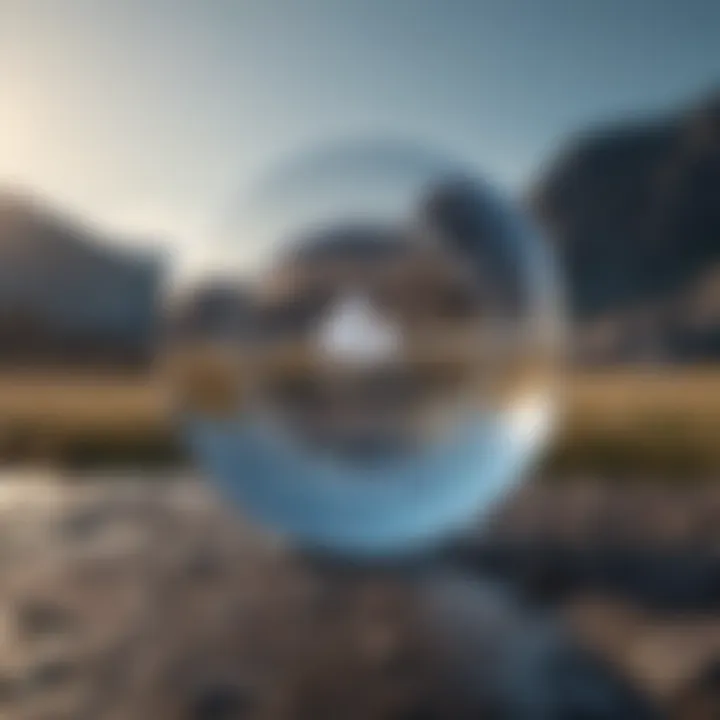
Safety Considerations
Hydrogen presents unique safety challenges that are critical to address in its various applications. Understanding these challenges is essential, especially given hydrogen's potential role as a clean energy source. This section delves into the importance of safe handling and storage practices as well as the transport regulations for hydrogen. These considerations ensure that hydrogen is utilized effectively while minimizing risks associated with its flammability and reactivity.
Handling and Storage
Effective handling and storage of hydrogen are vital to preventing accidents. Hydrogen is colorless, odorless, and highly flammable, making it difficult to detect leaks. Due to its low density and small molecular size, hydrogen can easily escape and accumulate in confined spaces, posing explosion risks. Therefore, industries working with hydrogen must implement stringent safety protocols.
- Storage Solutions: Hydrogen can be stored in various ways, including compressed gas cylinders, liquid hydrogen tanks, and solid-state hydrogen storage systems. Each method has its own advantages and challenges. For instance, liquid hydrogen requires cryogenic temperatures for storage, while gas cylinders need robust pressure-resistant materials.
- Ventilation: Adequate ventilation is necessary in areas where hydrogen is stored or used. This helps disperse any leaked hydrogen and minimizes explosion risks. Continuous monitoring systems are also recommended for detecting hydrogen concentrations in the air.
- Personal Protective Equipment (PPE): Workers must wear appropriate PPE, such as flame-resistant clothing and safety goggles, to protect themselves from potential hazards.
Transport Regulations
Transporting hydrogen involves a set of regulations designed to ensure safety in transit. These regulations address the requirements for packaging, labeling, and transportation infrastructure. Following proper guidelines is crucial in preventing accidents during hydrogen transport.
- Packaging Standards: Hydrogen must be transported in compliant containers that can withstand high pressures and are resistant to leaks. Specific standards dictate the design and construction of these pressure vessels.
- Labeling and Documentation: Vehicles transporting hydrogen must display clear hazard labels that inform others of the flammable nature of the cargo. Proper documentation, including details about the quantity and properties of hydrogen, is necessary for regulatory compliance.
"Safety in handling and management of hydrogen is not just regulatory; it is essential for preventing disasters."
- Transport Modes: Hydrogen can be transported via pipelines, trucks, or rail. Each mode has its own regulatory framework overseeing safety measures during transport. For instance, hydrogen pipelines must comply with stringent safety standards to prevent leaks.
Future Prospects of Hydrogen Research
The role of hydrogen in modern science is continually evolving, making its future prospects both intriguing and essential. As energy demands rise and environmental concerns become more pressing, researchers seek innovative solutions through hydrogen. This section discusses advancements necessary for hydrogen technology and its potential for a sustainable future.
Innovations in Hydrogen Production
Recent years have seen significant innovations in hydrogen production methods. Traditional processes like steam methane reforming, while effective, contribute carbon emissions. Alternatives such as electrolysis are gaining traction. Electrolysis utilizes renewable energy to split water into hydrogen and oxygen. This method is crucial for producing green hydrogen, which holds the potential to be a clean energy source.
Another area of innovation is biological production. Microorganisms can produce hydrogen through fermentation processes. This method often utilizes organic waste, leading to a dual benefit of waste management and hydrogen generation.
Furthermore, research is being conducted to enhance hydrogen production efficiency. Advances in materials science may lead to better catalysts, reducing energy required for electrolysis. Additionally, thermochemical processes involving high temperatures can also be explored. These methods promise to minimize the carbon footprint further while increasing hydrogen yield.
"Innovations in hydrogen production can redefine energy landscapes by providing cleaner alternatives to traditional fossil fuels."
Potential for Hydrogen Economies
The transition towards hydrogen economies is another promising prospect. Nations are recognizing hydrogen’s potential to diversify energy sources and reduce dependency on fossil fuels. With proper infrastructure, hydrogen can be utilized not only for transport but also for heating and power generation.
Investments in hydrogen infrastructure are crucial. Countries like Germany and Japan are leading efforts in developing hydrogen grids and fueling stations. As the technology matures, scale economies could result in lower costs for hydrogen production and distribution, making it a viable alternative for consumers and industries alike.
However, challenges remain. Safety, cost, and storage issues must be addressed effectively. Overcoming these hurdles will determine the pace at which hydrogen economies can develop. Therefore, ongoing research and collaboration between governments, academia, and industries are imperative to realize the full potential of hydrogen.
In summary, the future of hydrogen research is full of potential. Innovations in production and the establishment of hydrogen economies could significantly contribute to global sustainability goals. It underscores hydrogen's multifaceted role in addressing modern energy challenges.
Finale
The exploration of hydrogen in modern science plays a crucial role in several domains, ranging from energy production to environmental sustainability. This article synthesizes diverse aspects of hydrogen, enabling a deeper appreciation of its multifaceted nature.
Summary of Findings
Throughout this discussion, several key points emerge regarding the significance of hydrogen.
- Chemical Properties: Hydrogen is the lightest and most abundant element in the universe. Its unique properties enable it to serve as a potential energy carrier.
- Production Methods: Various methods, including electrolysis and steam methane reforming, show potential for producing hydrogen sustainably.
- Environmental Impact: Hydrogen has a relatively low carbon footprint compared to fossil fuels. However, the source of the hydrogen plays a significant role in its overall environmental impact.
- Applications: Hydrogen finds applications in industries such as aerospace, automotive, and chemical manufacturing. This versatility highlights its importance in addressing current and future energy demands.
- Safety Considerations: The necessary precautions for handling and storage are essential. Awareness of transport regulations aids in the responsible and safe movement of hydrogen.
- Future Prospects: Ongoing research aims to innovate hydrogen production techniques, moving towards hydrogen economies which could reshape energy consumption.
Final Thoughts on Hydrogen
The future of hydrogen is promising, but it demands careful consideration. The path to a sustainable hydrogen economy is fraught with challenges but offers significant opportunities for innovation and advancement in science and technology.
The journey towards hydrogen's full adoption requires commitment and collaboration across industries. Sustainable practices must be at the forefront of this evolution.
By recognizing the findings discussed in this article, students, researchers, educators, and industry professionals can position themselves at the cutting edge of this transformative element and its applications.
With hydrogen's critical role becoming more apparent, the collective focus should be not only on its potential but also on the practicalities surrounding its use and the structures needed to support its integration into existing systems.