Neon Electroporation: Applications and Insights for Cell Lines
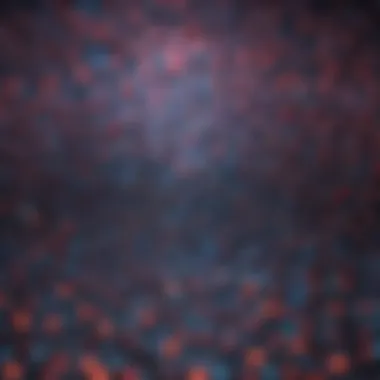
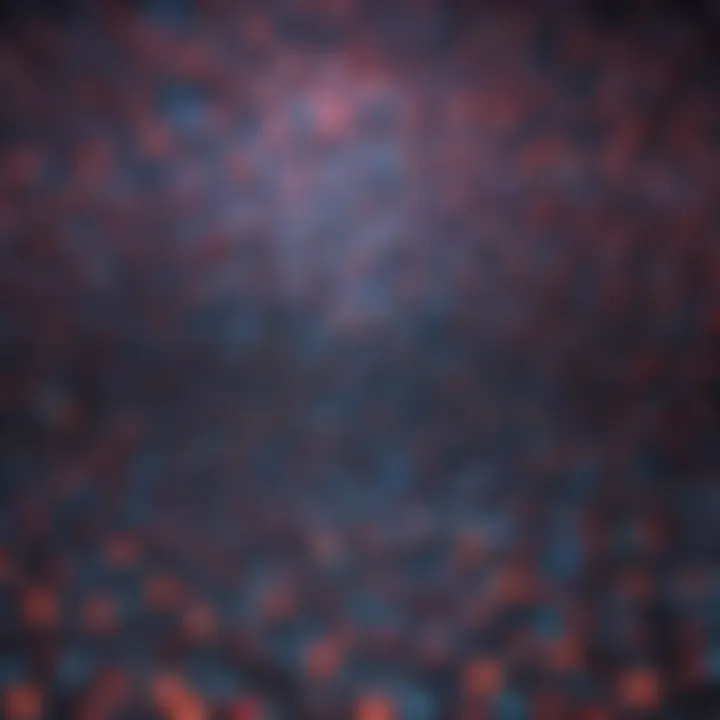
Intro
Neon electroporation represents a specialized methodology in the field of cellular engineering. This technique employs a unique combination of neon light and electroporation principles to enhance the permeability of cell membranes. As scientists advance their understanding of cellular functionalities, methods like neon electroporation take center stage due to their potential applications in areas such as gene therapy, vaccine development, and synthetic biology.
This article presents an exhaustive review of neon electroporation, which is essential for both research and industrial applications. The process not only alters cell membranes but also allows for the infusion of genetic material into various cell lines. This can lead to significant advancements in therapies for diseases, such as cancer or genetic disorders, widening the horizon for therapeutic interventions.
Research Background
Overview of the Scientific Problem Addressed
Cell membrane permeability is a crucial factor in the successful delivery of drugs and genetic materials to cells. Traditional methods for increasing permeability, such as chemical treatments or mechanical approaches, often lack precision and efficiency. Neon electroporation aims to address these shortcomings by providing a non-invasive means to enhance cellular uptake of materials through controlled electrical pulses accompanied by light stimulation.
Historical Context and Previous Studies
Historically, the use of electroporation dates back to the 1980s when scientists demonstrated that applying an electric field could disrupt cell membranes temporarily, allowing for increased molecule entry. Over the years, numerous studies have explored various forms of electroporation using different light sources and electrical settings. Research into the integration of neon light into this process is relatively recent but shows promising results.
In these earlier studies, researchers documented various types of cell lines that were successfully electroporated, revealing significant enhancements in the uptake of plasmids and other substances. The transition from conventional electroporation to neon-enhanced methods demonstrates the evolution of this field, promising better control over the electroporation process and potentially leading to improved outcomes in diverse applications.
Findings and Discussion
Key Results of the Research
Substantial findings have emerged from studies investigating the implications of neon electroporation. Key results indicated that specific cell lines, such as HEK293, can exhibit up to a 50% increase in gene transfer efficiency when illuminated with neon light during the electroporation process. This is vital for researchers looking to improve the delivery of therapeutic genes into cells.
Interpretation of the Findings
These findings suggest that neon electroporation could serve as a powerful tool in molecular and cell biology. The heightened efficiency not only aids researchers in achieving desired outcomes but could potentially lead to broader use in clinical settings. For instance, enhancing gene therapy techniques may provide new routes for treating genetic disorders that currently have limited treatment options.
Prelims to Electroporation
Electroporation is a sophisticated technique used in molecular biology to enhance the permeability of cell membranes. This process involves applying an electric field to induce temporary pores in the cell membranes, allowing for the uptake of various molecules, such as DNA, RNA, or drugs. In the context of this article, the focus on neon electroporation emphasizes how different light sources, specifically neon, can optimize this process for various cell lines.
Understanding electroporation is essential for many reasons. First, the technique allows for efficient transformation of cells, making it invaluable in research and therapeutic applications. It plays a significant role in gene expression studies, genetic engineering, and even vaccine development. The versatility of this approach is particularly beneficial for researchers aiming to alter or enhance cell function or behavior in controlled scientific experiments.
In addition to its applications in fundamental research, electroporation offers practical advantages in the fields of medicine and biotechnology. Utilizing this process can lead to effective gene delivery systems, which are critical in gene therapy. Moreover, harnessing the specificity of electroporation can minimize collateral damage to surrounding tissues, making it a powerful tool for targeted treatments.
Lastly, the advancement in electroporation technologies, especially through the combination with neon light, represents a frontier in cellular manipulation. This article aims to dissect each aspect of neon electroporation, showcasing its methodologies, outcomes, and potential future directions, and thereby providing a comprehensive overview for students, researchers, educators, and professionals in the field.
Definition of Electroporation
Electroporation refers to a process where an external electric field creates temporary pores in the cell membrane. This phenomenon allows for the passage of large molecules that typically cannot cross the lipid bilayer of the cell membrane. In practical terms, electroporation enhances various applications such as gene transfer, drug delivery, and the introduction of other substances into cells.
The process can be manipulated by varying the strength and duration of the electric field. These characteristics determine the efficiency and the extent of membrane permeabilization, offering a versatile tool for biological research.
Historical Context and Development
The concept of electroporation dates back to the mid-20th century, gaining traction as a method for cell transformation. Early studies demonstrated the capability of electric fields to induce permeability in bacterial cells. Over the years, significant developments improved the technique's efficiency and application range.
In the 1980s, as molecular biology advanced, researchers began exploring electroporating not just bacteria but also mammalian cells. This evolution led to further studies that uncovered various parameters influencing electroporation efficiency, such as pulse duration, field strength, and the physical state of the cell membranes.
Today, electroporation is an established method in both academic and clinical laboratories. Its integration with modern technologies, including neon light sources, enhances its applicability and precision in cell line studies, making it a notable focus for ongoing research.
Principles of Neon Electroporation
Understanding the principles of neon electroporation is crucial as it lays the foundation for the effective and efficient application of this technology in cellular research. Neon electroporation stands out through its mechanism, where electrical impulses and light interplay, offering advanced methods for gene delivery and other cellular manipulations. The ability to control cellular permeability is a significant benefit of this approach. Researchers have noted that optimizing electroporation parameters can lead to improved transfection outcomes while maintaining cell viability.
Mechanisms of Electroporation
Electric Field Effects
Electric field effects play a vital role in the electroporation process. This mechanism involves applying an electric field to the cell membrane, causing temporary disruptions. The key characteristic of this phenomenon is its ability to form pores in the lipid bilayer, which facilitates the entry of various molecules into the cell. It becomes a beneficial choice in applications like DNA transfection and drug delivery because it enhances cellular uptake rates.
A unique feature of electric field effects is that the intensity and duration of the field can be adjusted to minimize cellular stress. However, improper calibration might lead to unintended damage, highlighting the importance of optimizing these parameters for each specific application.
Membrane Permeability Changes
Membrane permeability changes are another fundamental aspect of the electroporation mechanism. This process effectively alters the membrane properties as the electric field is applied. Itβs recognized for its role in allowing significant molecular exchange between the extracellular and intracellular environments. The key characteristic of this mechanism is its dynamic nature, which can be influenced by both the electric field applied and the specific cell type used.
Membrane permeability changes can be advantageous due to their potential to increase uptake efficiency. However, an excessive increase in permeability could lead to cell lysis. Therefore, careful consideration must be given to balance the achievable permeability enhancement against cell survival rates.
Role of Neon in Electroporation
The role of neon in electroporation introduces an innovative layer of photonic activation. Neon light targets specific cellular outcomes, enhancing the overall transfection process. This approach benefits researchers aiming for precision in genetic engineering and therapeutic applications.
Photonic Activation
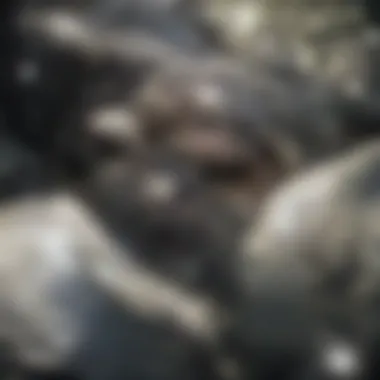
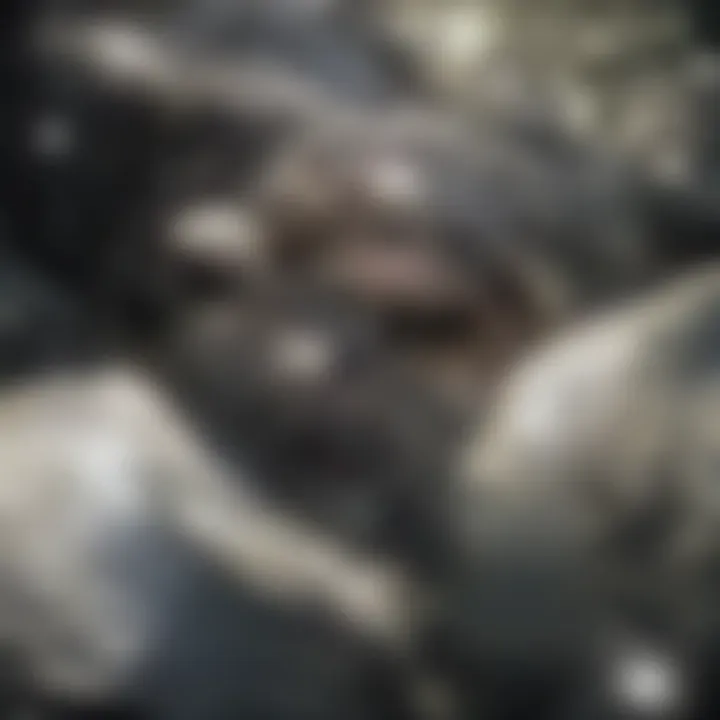
Photonic activation with neon light facilitates additional energy to the procedure, enabling more profound effects on membrane dynamics. This characteristic allows for improved control over the process, making it a popular choice for those looking to refine electroporation techniques. The unique feature of this method is the simultaneous application of light, which aids in cellular activation and potentially enhances the therapeutic effect.
The advantages of photonic activation include increased transfection efficiency and reduced mutagenesis, which is a concern in other methods of electroporation. This highlights the potential of neon light as a powerful tool in modern biological research.
Comparison with Other Light Sources
When comparing neon with other light sources, it becomes evident that neon provides unique benefits due to its specific wavelength characteristics. Unlike traditional light sources, neon light offers a stable and consistent output, crucial for reproducibility in research.
The key strength of using neon over other sources lies in its capacity for selective excitation of certain cellular structures. This can lead to more targeted therapies and efficient gene delivery. However, this advantage may come with the downside of limited availability in some laboratory setups, which could restrict its widespread adoption compared to more common light sources.
Neon electroporation combines techniques that enhance cell permeability while minimizing damage, providing a pathway for advanced cellular manipulation.
By comprehensively understanding the principles and mechanisms at play in neon electroporation, researchers can harness this technology to its fullest extent, pushing the boundaries of cellular biology research and applications.
Cell Lines Utilized in Neon Electroporation
Cell lines play a central role in the research and application of neon electroporation. These cultures of cells provide a controlled environment for scientists to investigate the effects of electroporation on various biological processes. By using specific cell lines, researchers can assess the efficiency of transfection and the overall viability of cells post-poration. Choosing the correct cell line is not merely a matter of convenience; it is essential for achieving significant results in experimental studies.
Types of Cell Lines
Mammalian Cell Lines
Mammalian cell lines are one of the most commonly used types in neon electroporation. These cells closely mimic human biological processes, making them vital for studies in gene therapy and drug development. One key characteristic of mammalian cell lines is their sensitivity to electroporation conditions. This sensitivity can lead to higher transfection rates when optimized appropriately. The unique feature of mammalian cell lines lies in their complexity, which allows for diverse cellular responses and interactions. Despite their advantages, mammalian cell lines can be more expensive to maintain compared to simpler systems.
Bacterial Cell Lines
Bacterial cell lines present a different but equally important choice for neon electroporation. The simplicity and rapid growth of these cells make them advantageous for studies focused on genetic engineering and synthetic biology. One key aspect of bacterial cell lines is their ability to take up foreign DNA efficiently, often yielding higher transfection efficiencies. Additionally, bacterial systems are generally easier and less costly to handle. However, their prokaryotic nature means that results cannot always be directly translated to eukaryotic systems, which can be a limitation in certain studies.
Plant Cell Lines
Plant cell lines are gaining attention in the field of neon electroporation, particularly for applications in agricultural biotechnology. These cells offer unique insights into plant genetics and can be engineered for traits like pest resistance. One key characteristic of plant cell lines is their ability to regenerate whole plants from single cells. This regenerative capacity is a beneficial aspect for studies involving genetics and trait enhancement. However, plant cell lines may respond less predictably to electroporation compared to mammalian and bacterial lines, which can pose challenges during experiments.
Criteria for Cell Line Selection
Selecting the right cell line involves careful consideration of several factors.
Transfection Efficiency
Transfection efficiency is a crucial metric in evaluating the success of electroporation. Higher rates of transfection indicate better uptake of genetic material, which is essential for applications like gene therapy. The specific aspect of transfection efficiency in neon electroporation is defining optimal conditions that facilitate maximum genetic uptake. This efficiency makes transfection rates a primary concern in experimental design. However, achieving high transfection efficiency can sometimes lead to a trade-off with cell viability, necessitating careful optimization.
Cell Viability Post-Poration
Cell viability post-poration is another key criterion that cannot be overlooked. It assesses the health of cells after exposure to electric fields and neon light. Maintaining a high level of cell viability ensures that experimental results are relevant and reliable. The specific aspect of cell viability in neon electroporation is emphasizing the balance between sufficient electroporation for genetic uptake and preserving cellular integrity. Assessing cell viability not only informs the success of the electroporation process but also impacts future applications and experiments. If cell viability is compromised, the relevance of findings might be questioned, which can limit the potential impact of research.
Methodology of Neon Electroporation
Understanding the methodology of neon electroporation serves as a foundation to grasp its potential applications in modern cellular research. The methodology encompasses both the experimental setup and the protocol steps required for effective implementation of the process. Each component is essential for achieving the desired outcomes of electroporation, which primarily includes the introduction of substances into cells while maintaining their integrity. This section details the specific elements involved, focusing on the benefits, considerations, and crucial aspects of the methodology.
Experimental Setup
Electroporation Devices
The choice of electroporation devices is crucial in any research endeavor using neon electroporation. These devices use electric fields to create transient pores in the cell membrane, allowing for the delivery of various molecules such as DNA, RNA, or proteins. A key characteristic of these devices is their ability to control the electric field with precision. This controllability is beneficial in optimizing transfection efficiency while minimizing cellular damage. Many devices also feature adjustable parameters, enabling researchers to tailor the setup for different cell types and applications.
One unique feature of certain electroporation devices is their capability of integrating additional components, such as cooling systems. This advantage helps maintain optimal temperature during procedures, reducing heat stress on cells and thus improving overall cell viability post-electroporation, making them a preferred choice in this field.
Light Source Specifications
Light source specifications are another vital aspect of the experimental setup in neon electroporation. The use of neon as a light source is particularly relevant, as it enhances the permeability of cell membranes. Key characteristics of suitable light sources include intensity and wavelength. The right intensity is essential to activate the mechanism of electroporation effectively without causing harm to the cells.
A unique feature of neon light systems is their stable emission across various environments. This stability ensures consistent performance during experiments, thus granting reproducibility. However, one must consider that poor choices in light sources can lead to fluctuations in results, highlighting the necessity for careful selection.
Protocol Steps
Preparation of Cell Culture
Proper preparation of cell cultures is vital for successful neon electroporation. High-quality cell cultures ensure that the cells are healthy and in the right growth phase, which significantly impacts transfection efficiency. A key characteristic of this step is the optimization of culture conditions, such as nutrient media and growth factors. The right environment enhances the cells' ability to take up foreign molecules during electroporation.
In preparing cell cultures, specific considerations have to be taken. Contamination risks are a constant threat. Therefore, aseptic techniques are essential. If not adhered to, the results can be compromised, displaying poor transfection efficiency or viability.
Application of Electric Pulse
The application of the electric pulse is another crucial step in the methodology of neon electroporation. This pulse generates the electric field necessary to induce electroporation. Key characteristics of this process include the duration and intensity of the pulse. Both parameters directly affect the efficiency of substance delivery into the cells.
One vital aspect of this step is timing. An accurate timing sequence can significantly influence the results. If the pulse is too strong or too lengthy, it might lead to irreversible damage to the cells. Conversely, a pulse that is too weak may not achieve sufficient pore formation, leading to a low transfection rate.
Neon Light Exposure Duration

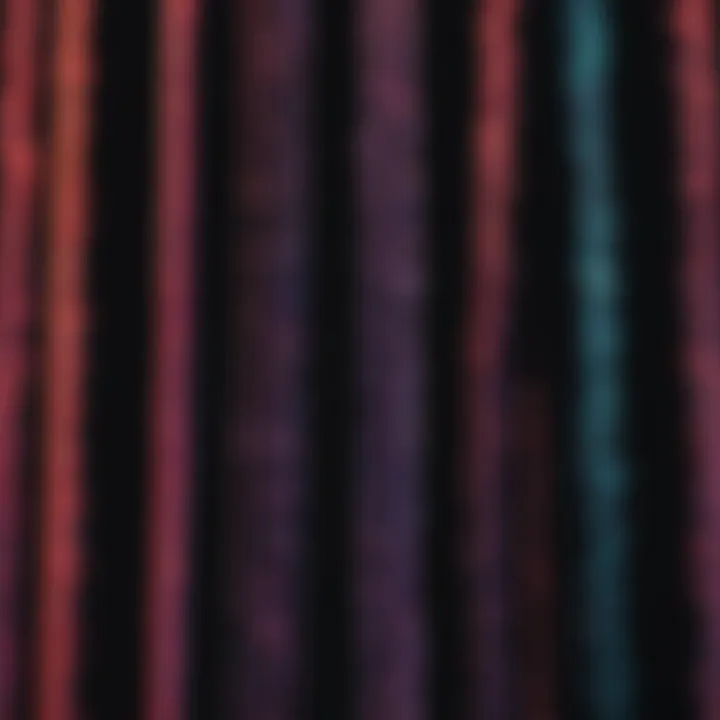
Neon light exposure duration is critical to ensuring the effectiveness of the methodology. Following electric pulse application, the right duration of exposure to neon light impacts the overall success of cell permeability enhancement.
A key characteristic is that this exposure needs to be optimized based on cell type. Different cells might respond variably to the same duration. Therefore, a unique feature of this aspect is the necessity for empirical testing. The advantage is that precise adjustments result in tailored methodologies for specific applications, but, unfortunately, it could also lead to variations in outcomes if initial conditions are not tested.
In summary, a well-structured methodology for neon electroporation is fundamental to its successful application in various cell lines. Careful attention to the experimental setup and protocol steps ensures that researchers can optimize the process for diverse applications, ranging from gene therapy to synthetic biology.
Outcomes of Neon Electroporation
The outcomes of neon electroporation are critical for understanding its efficacy and applications in various scientific fields. By evaluating the results obtained from this process, researchers can discern the potential of this technique for enhancing cellular permeability. The assessment of outcomes focuses on metrics such as transfection rates and the overall health of the cells post-electroporation. This information allows for optimization of methodologies and aids in drawing correlations between the electroporation process and its practical applications.
Assessment Metrics
Transfection Rates
Transfection rates are a key indicator of the success of neon electroporation. This metric quantifies the percentage of cells that have successfully incorporated exogenous genetic material. High transfection rates are essential for effective gene delivery, making this aspect of significant importance in research and clinical applications.
A major characteristic of transfection rates is their direct relationship with experimental conditions. Variations in electric field strength, pulse duration, and light exposure can all influence this rate, making careful calibration vital. A high transfection rate indicates a favorable environment for cellular uptake, therefore enhancing the method's attractiveness for gene therapies and other applications.
However, achieving high transfection rates can sometimes lead to challenges. For example, if the process is not optimized, it might result in high toxicity or reduced cell viability. Despite these challenges, the ability to effectively transfect cells is a strong reason why this metric is emphasized in assessments.
Cellular Health Indicators
Cellular health indicators are crucial for evaluating the impact of neon electroporation on cell lines. These indicators typically include parameters such as cell viability, growth rate, and metabolic activity following the procedure. Monitoring these aspects helps ensure that the cells remain functional and healthy, thereby confirming the technique's safety and effectiveness.
A notable feature of cellular health indicators is their ability to provide a comprehensive view of how cells respond to the electroporation process. This is particularly important since high transfection rates may come at the cost of cellular integrity. Maintaining a balance between effective transfection and the preservation of cellular health is a fundamental consideration for researchers.
One potential disadvantage of focusing solely on transfection rates is that it may obscure critical data on cell viability and overall functionality. Thus, incorporating cellular health indicators into assessments provides a more rounded understanding of the outcomes of neon electroporation.
Case Studies
Transfection of Reporter Genes
The transfection of reporter genes serves as a significant application of neon electroporation. This process involves introducing genes that encode for fluorescent proteins, allowing researchers to visualize and quantify gene expression effectively. The ability to track these reporter genes enhances the understanding of various biological processes and the effects of genetic modifications.
A key characteristic of this application is its relevance in both basic and applied research. For instance, reporter gene assays are widely used in drug development to monitor the effects of pharmacological compounds on gene expression. This makes the transfection of reporter genes an attractive option within this field, as it allows for real-time monitoring of cellular responses.
While beneficial, there are some challenges associated with reporter gene transfection. The reliability of results can depend heavily on the stability of the incorporated gene and the expression systems used. These factors should be considered when planning experiments.
Drug Delivery Applications
Neon electroporation is also being explored for drug delivery applications. This technique shows promise in facilitating the entry of therapeutic compounds into target cells. By leveraging the enhanced permeability induced by electroporation, researchers can improve the efficiency of drug delivery systems.
One major characteristic of drug delivery applications is their potential to enhance treatment efficacy. Through targeted delivery, localized therapy becomes more feasible, reducing systemic side effects and improving patient outcomes. This makes drug delivery applications a significant area of interest for research and development.
However, challenges can arise in determining the optimal conditions for drug delivery. Factors such as drug stability, timing of electroporation, and subsequent cellular responses need careful consideration. Addressing these concerns remains essential for harnessing the full potential of neon electroporation in therapeutic scenarios.
Applications of Neon Electroporation
Neon electroporation, a method employing electric fields and neon light, presents remarkable prospects across various applications in cellular biology. Its importance lies not only in its novel approach but also in enhancing existing methodologies. Specifically, it allows for targeted cellular manipulations that traditional methods may not achieve with equal efficacy. This section delves into three primary applications: gene therapy, vaccine development, and synthetic biology.
Gene Therapy
Gene therapy represents a prominent application of neon electroporation. This approach harnesses the unique methods of action to effectively introduce genetic material into cells, thus modifying their functions.
Mechanisms of Action
The mechanisms of action involved in gene therapy via neon electroporation are pivotal. Electric pulses create temporary pores in the cellular membrane, enabling the uptake of nucleic acids. This feature is essential, as it significantly increases transfection efficiency. One key characteristic of this mechanism is its precision in delivering specific genes without damage to the surrounding cellular structures.
Neon light aids in further enhancing the permeabilization, thereby increasing the rate of successful gene introduction. While advantageous, the reliance on precise electric field parameters remains a challenge, requiring meticulous calibration to minimize cellular stress.
Clinical Applications
Clinical applications of neon electroporation in gene therapy focus on conditions such as genetic disorders and cancers. Its pivotal role serves in producing targeted treatment options, showcasing its potential to address previously untreatable diseases. A notable feature of clinical applications is the adaptability of the method, which allows customization based on patient needs and specific disease states. However, considerations for patient safety and effective dosages of both electric fields and genetic material are crucial. This cautious approach can impact the pace of clinical adoption, but the resulting therapies could revolutionize patient care.
Vaccine Development
The expanding field of vaccine development greatly benefits from neon electroporation's capabilities. Traditional methods often face limitations in efficacy and speed, which neon electroporation seeks to overcome.
Potential Strategies
Potential strategies for applying neon electroporation in vaccine development include using it to enhance antigen delivery systems. This approach significantly boosts the immune response, improving vaccine effectiveness. Moreover, it presents a rapid means of formulation, allowing for timely vaccine rollout during outbreaks. One notable characteristic of these strategies is the ability to tailor the electroporation parameters for specific antigens, thus optimizing responses. Nevertheless, the long-term stability of the resultant vaccines in varied conditions remains a point of concern.
Advantages Over Traditional Methods
The advantages that neon electroporation presents over traditional vaccine development methods are noteworthy. Enhanced immune responses and reduced timeframes for vaccine production are paramount advantages. Moreover, this method allows for multiplexing of antigens, meaning a single electroporation can deliver multiple components of a vaccine simultaneously. This efficiency can considerably reduce the time and resources needed for vaccine development. Despite these advantages, regulatory frameworks may require significant evaluation and validation to ensure safety and efficacy, potentially delaying widespread application.
Synthetic Biology
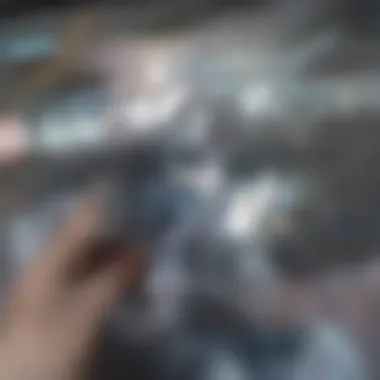
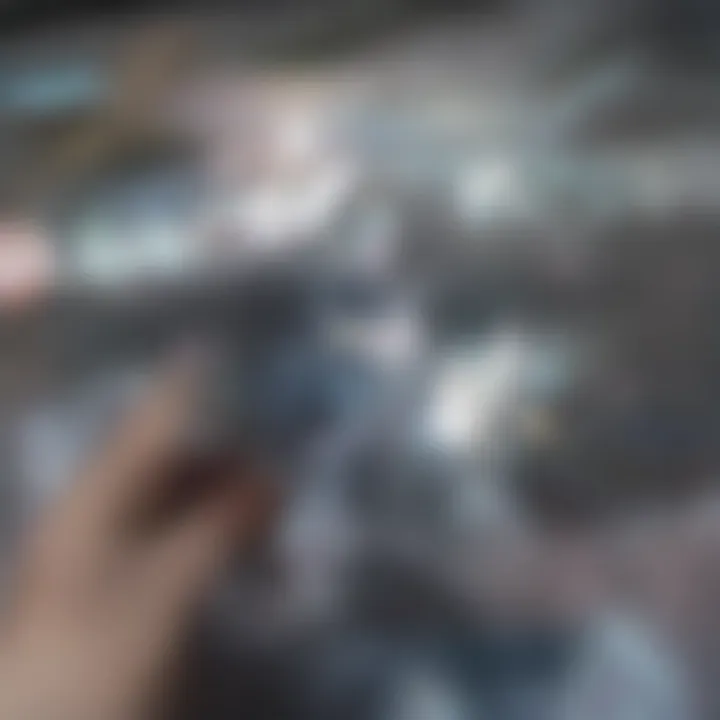
The innovative field of synthetic biology is inherently suited for the application of neon electroporation, fostering new methods of genetic manipulation and bioengineering.
Construction of Genetic Circuits
The construction of genetic circuits takes considerable advantage of neon electroporation. This technique facilitates the introduction of multiple genetic elements into living organisms, allowing complex circuit designs. A key characteristic of this process is its ability to improve the functionality of these circuits in dynamic environmental conditions. Moreover, the versatility of neon allows for higher precision in placing genetic elements where desired within host cells. However, successful integration into the coding sequences can still present challenges.
Applications in Bioengineering
Applications in bioengineering benefit from the utility of neon electroporation for creating bio-based products, such as biofuels and pharmaceuticals. This method enables multifunctional modifications in microbial and algal systems. Such contributions make it a highly beneficial choice in the ever-evolving fields of biotechnology. However, there may be concerns regarding scalability and economic feasibility, which require attention for broader industrial adoption.
Challenges in Neon Electroporation
Understanding the challenges in neon electroporation is crucial for researchers and practitioners. This technique, while promising, faces multiple hurdles that can affect its effectiveness and application. Addressing these challenges can lead to more successful outcomes in experiments involving cell lines. Several aspects, including technical limitations and biological considerations, require careful thought and planning.
Technical Limitations
Equipment Constraints
Equipment constraints are significant when considering neon electroporation. Implementing electroporation requires specialized devices that are not always easily accessible. The reliance on precise equipment limits the ability of some laboratories to conduct high-quality experiments. It is vital to use devices specifically designed for electroporation to ensure consistent results. This equipment often comes with various features, such as adjustable pulse parameters, which contribute to the overall success of the process.
Ultimately, while the cost of equipment can be a barrier, advanced tools offer enhanced control over the electroporation process. Researchers must weigh the pros and cons of investing in quality devices versus the potential risks of using substandard equipment. The challenge lies in balancing budget constraints with the need for operational efficiency.
Optimization of Parameters
Optimization of parameters stands as another crucial element in neon electroporation. The process requires fine-tuning parameters like electric field strength and pulse duration to achieve desired outcomes. Each cell line may respond differently to these variables, demanding a customized approach for each experiment.
This ongoing requirement to calibrate conditions highlights the complexity of the technique. Finding the right combination of settings maximizes transfection rates while minimizing cellular damage. Successful optimization requires a significant investment of time and resources. As such, continuous research is necessary to develop guidelines for better parameter selection.
Biological Considerations
Cell Type Variability
Cell type variability presents a challenge during neon electroporation. Different cell lines exhibit varied properties regarding permeability and resilience to electroporation. Such differences can significantly impact transfection efficiency. A cell line that responds well to one experiment might fail in another due to intrinsic biological characteristics.
This variability necessitates thorough preliminary testing for each cell line of interest to determine optimal conditions before proceeding with larger experiments. Adequate understanding of the unique features of each cell line can allow researchers to tailor the protocol effectively, thus enhancing the overall success of neon electroporation efforts.
Potential Cellular Damage
Potential cellular damage is an important consideration in the context of neon electroporation. The introduction of an electric field can cause unintended harm to cells, leading to cell death or impaired function. Monitoring toxicity levels during electroporation is therefore critical.
Research has shown that certain parameters can mitigate cellular damage. For instance, shorter pulse durations generally create less stress on the cell membrane. However, this must be balanced against effective transfection rates. Scientists face the challenge of ensuring that they minimize potential cellular damage while maximizing transfection efficiency.
"The ability to balance the benefits of effective transfection and the risks of cell damage defines the future success of neon electroporation in various applications."
Future Directions in Neon Electroporation Research
The study of neon electroporation is at a pivotal point. Substantial advancements in this technology have emerged alongside a growing recognition of its potential across multiple disciplines. As researchers delve deeper into the nuances of cellular behavior under electroporation conditions, several promising directions for future investigation become apparent. This branch of research is crucial not only for improving existing methodologies but also for exploring new horizons in cellular manipulation.
Innovations in Electroporation Techniques
Recent years have seen a wave of innovations in electroporation techniques. Researchers are striving to refine existing methodologies, improving precision in applying electric fields. One notable technique includes the integration of microfluidics with electroporation. This method allows for a more controlled environment, enabling better handling of cells. By doing so, it optimizes transfection efficiencies while lowering the risk of cellular stress.
Additionally, advancements in electrode design have emerged. These new configurations can address challenges related to heterogeneous cell populations. By using multiplexed electrode systems, researchers can target various cell types simultaneously, enhancing the versatility of the technique. Moreover, programmable electroporation devices are on the rise. These devices utilize software to fine-tune pulse parameters, allowing researchers to adjust frequency, intensity, and duration based on specific cell line requirements.
The use of real-time monitoring tools during electroporation procedures is another innovation. Implementing imaging techniques during the process provides immediate data on cellular responses, helping to refine protocols on the fly. These innovations signify a shift towards more adaptive and responsive electroporation practices.
Expanding Applications Beyond Current Utilizations
The applications of neon electroporation extend well beyond traditional realms. Currently, it serves vital roles in gene therapy, vaccine development, and synthetic biology. However, researchers are probing outside these established boundaries. For instance, neon electroporation is being evaluated in regenerative medicine. The potential to deliver precise gene edits or therapeutic agents directly into tissues is profound. Such applications could revolutionize how we approach tissue repair and regeneration.
Furthermore, the industrial sector is beginning to recognize the advantages of neon electroporation for bioproduction processes. The technique could be applied to enhance metabolic pathways in microbial cell factories, thereby increasing yield efficiency. Additionally, explorations into agricultural biotechnology are underway, with neon electroporation being considered for enhancing plant traits.
Ongoing research is also directed at improving understanding of electroporation effects on larger multicellular structures. Another exciting avenue involves hybrid techniques combining electroporation with CRISPR gene editing technologies. By integrating these tools, researchers can achieve more efficient and targeted genetic modifications.
The trajectory of neon electroporation research is promising. With continuous innovations and expanding applications, the field is poised for significant breakthroughs, potentially transforming approaches across biology and medicine.
Closure
Neon electroporation has emerged as a significant technique in cellular research and biotechnological applications. Its importance lies not just in the principles it utilizes, but also in its versatility across various disciplinary boundaries. The successful application of neon electroporation contributes to advancements in gene therapy, vaccine development, and synthetic biology. Moreover, it poses intriguing possibilities for future breakthroughs in medicine and biotechnology.
Summary of Key Points
In reviewing the prior sections, several key points become apparent:
- Mechanisms of Electroporation: Understanding the mechanisms is essential for optimizing the technique. Electric fields induce changes in cellular membranes, significantly increasing permeability.
- Role of Neon Light: The special properties of neon light facilitate the process of electroporation, enhancing the efficacy of cellular infiltration compared to other light sources.
- Diverse Cell Lines: A variety of cell lines can be utilized, including mammalian, bacterial, and plant cell lines, each offering unique advantages based on the experimental goals.
- Protocols and Methodologies: Standardized protocols ensure reproducibility and reliability in experimental outcomes, making it easier to assess results across different studies.
- Challenges Addressed: As with any technology, neon electroporation faces challenges like equipment limitations and biological variability. Addressing these concerns is crucial for widespread acceptance and application.
- Impacts on Future Research: The findings indicate potential avenues for increased research, emphasizing the need for continuous innovation in both technique and application.
Implications for Future Research
Future research in neon electroporation holds promising potential across various sectors. Specifically:
- Innovative Techniques: Exploring new electroporation techniques might further enhance cellular uptake. This may include advances in equipment design that tailor to specific cell types or uses.
- Cross-Sector Applications: Beyond current uses, the technique may find applications in personalizing medicine, augmenting gene therapy strategies, or designing novel vaccines.
- Investigating Cellular Responses: More comprehensive studies on how different cell types respond to neon electroporation could lead to optimized protocols and enhance overall efficacy.
- Student and Educator Involvement: Engaging students and educators in this evolving field can foster a new generation of researchers. Their insights may drive innovative approaches and broaden the understanding of electrophysiological processes.
Research focusing on these avenues can lead to impactful findings that will shape future studies, ultimately refining neon electroporation's role in molecular biology and biomedicine.