Neutron Battery: Principles, Applications, and Future
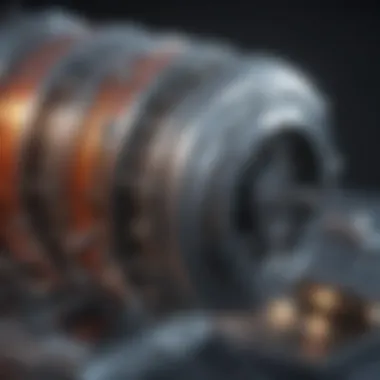
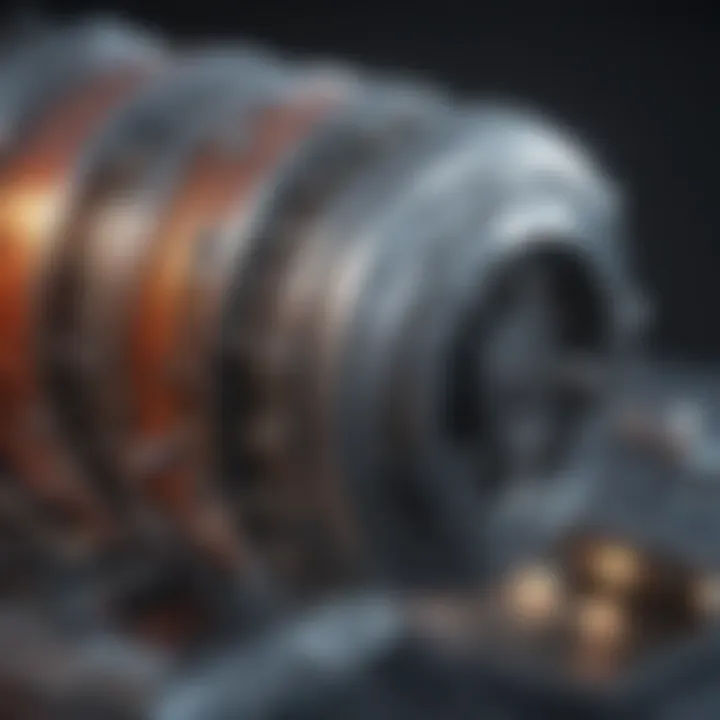
Intro
The emergence of advanced energy storage technologies has played a pivotal role in addressing today’s pressing issues related to energy consumption and sustainability. One of the promising approaches gaining attention is the neutron battery. This innovative system harnesses the unique properties of neutrons as a means to revolutionize the way we store and use energy.
Neutron batteries are grounded on principles that differ significantly from conventional battery technologies. Instead of relying on electron flow, these batteries utilize neutrons to facilitate energy storage and discharge. This fundamental shift offers intriguing possibilities, not just for enhancing energy efficiency but also for addressing various applications across different industries.
As we dive into this exploration of neutron batteries, we’ll illuminate the scientific challenges that prompted their development and chart their historical context. By doing so, readers will understand the relevance and potential impact of these systems in the energy landscape.
"The world is on the brink of an energy revolution. Neutron batteries might just light the way."
Let’s unravel this concept, one layer at a time, starting with a deep look into the research background in the realm of neutron battery technology.
Prologue to Neutron Batteries
The pursuit of efficient energy storage is at the forefront of modern technological challenges, and the interest in neutron batteries offers a unique perspective in this field. With increasing global energy demands and the push for sustainable solutions, understanding neutron batteries becomes pivotal. They present an intriguing alternative to conventional energy storage methods, potentially transforming how we think about power sources.
Understanding Energy Storage
Energy storage refers to the capture of energy produced at one time for use at a later time. In many cases, this involves converting energy into a format for storage that later can be converted back into usable forms. Traditional methods rely heavily on chemical reactions, seen in popular batteries like lithium-ion or lead-acid cells. However, neutron batteries operate on different principles.
Neutron batteries typically utilize the energy from neutrons, produced through nuclear reactions, to generate electric power. This process could result in batteries with significantly longer lifetimes and efficiency rates compared to conventional batteries. The fundamental concept here is to harness the intrinsic properties of elementary particles in service of energy, a feat not achievable by standard electrochemical means.
This nuanced understanding goes beyond just storing energy; it places neutron batteries at the intersection of nuclear physics and renewable energy technology. As mentionabl, the efficiency and energy density of neutron batteries could prove to be groundbreaking in applications where longevity and reliability are crucial, such as in aerospace and medical devices.
Significance of Neutron Batteries
The allure of neutron batteries lies in their potential advantages over existing energy systems. For one, they may provide a more stable energy source due to the inherent characteristics of neutrons, which can lead to reduced degradation over cycles.
Moreover, their energy density has the potential to be exponentially higher than that of chemical batteries. This means a neutron battery could store significantly more energy in a compact form, making it ideally suited for portable devices, electric vehicles, and even grid stabilization efforts.
"Neutron batteries stand at the brink of innovative energy solutions, merging nuclear physics with everyday energy needs."
In weathering the environmental concerns tied to traditional battery production, neutron batteries could pose less toxicity and a lower overall ecological footprint. The limited resource requirement for neutron generation can make them more sustainable when compared to the mining and disposal issues faced by lithium or cobalt.
All these aspects point towards a substantial impact on future energy landscapes. As we dig deeper into the mechanisms and implications of neutron batteries, it becomes clear that this topic is not merely another chapter in energy technology but perhaps a turning point.
The Fundamentals of Neutron Production
Understanding neutron production is like peeling an onion; each layer reveals crucial elements that shape the efficiency and capabilities of neutron batteries. At its core, the production of neutrons is vital for establishing a reliable energy source that can cater to various technological demands. In this section, we will explore different sources of neutrons, look into how these methods play their part in the broader narrative of neutron batteries, and evaluate the mechanisms that allow for neutron collection. This foundation will set the stage for understanding how neutron batteries can operate efficiently and sustainably.
Neutron Sources Overview
Neutron sources are integral to the workings of neutron batteries. There are three main forms of neutron production: fission processes, fusion reactions, and radioactive decay. Each of these methods has its own characteristics and implications.
Fission Processes
Fission processes are a fundamental reaction in nuclear physics, involving the splitting of heavy atomic nuclei into smaller particles. This splitting generates a substantial amount of energy, coupled with the release of neutrons. One of the key characteristics of fission is its ability to produce a large number of neutrons in a very short span of time.
- Why Fission is Beneficial: Fission is widely considered beneficial for neutron batteries primarily due to its high neutron yield compared to other sources. With a careful balance, fission can sustain power without the need for external energy inputs.
- Unique Features and Challenges: However, fission does come with notable challenges, including the management of radioactive byproducts and the perpetual need for cooling systems to mitigate heat production.
Fusion Reactions
Fusion reactions, on the other hand, involve the merging of light atomic nuclei to form a heavier nucleus, simultaneously releasing energy and neutrons. Fusion holds an almost mythical status due to its potential for a cleaner energy path. The most showcased fusion reaction involves isotopes of hydrogen, commonly deuterium and tritium.
- Key Characteristics:
Fusion is often highlighted for its clean energy aspect, as the byproducts are usually less hazardous than those of fission. - Advantages and Limitations:
While fusion could provide nearly limitless energy, the complex conditions required for initiating fusion reactions limit its practicality at present, although research continues to yield promising developments.
Radioactive Decay
Radioactive decay represents a more passive means of neutron production, where unstable isotopes emit neutrons as they transition to stable forms. Unlike fission or fusion, this process occurs spontaneously and continuously over defined half-lives.
- Characteristics and Appeal:
A key appeal of radioactive decay is its straightforwardness; it doesn't require an intricate setup to induce reactions, making it accessible for various applications. - Disadvantages:
Yet, it suffers from limitations such as the relatively low neutron output and the long timescales involved in decay processes.
Collection Mechanisms for Neutrons
Once neutrons are produced, collecting them effectively becomes equally important. Two primary mechanisms govern this aspect: neutron capture theory and detection techniques.
Neutron Capture Theory
Neutron capture theory refers to the process through which free neutrons are absorbed by materials, forming stable or unstable nuclides. This mechanism is paramount in controlling the amount of energy and resources harnessed from neutron production.
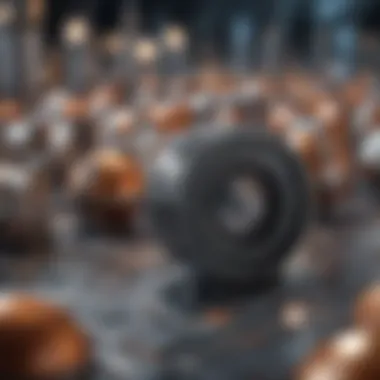
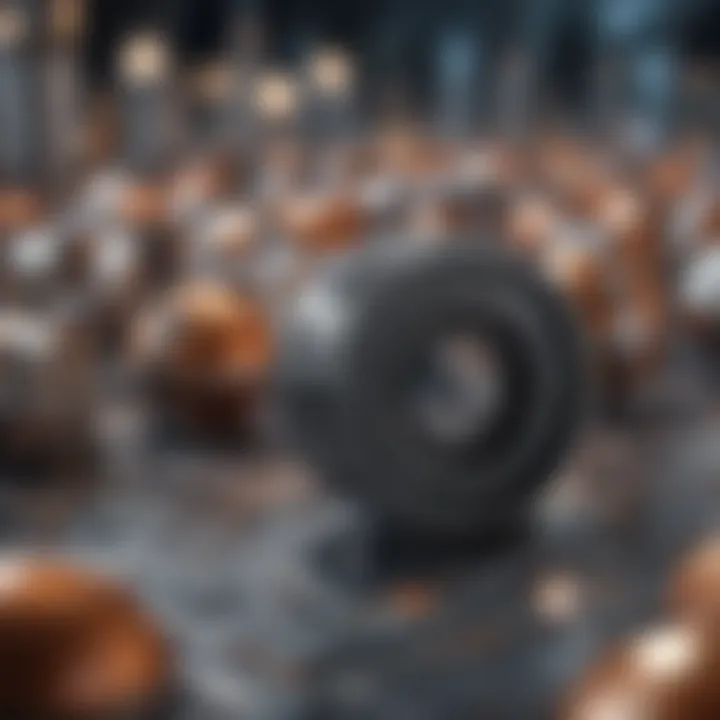
- Why it Matters:
The theory demonstrates how neutrons, when captured, can significantly influence the energy output and efficiency of neutron batteries. - Unique Features: An advantage of neutron capture is its ability to selectively target specific isotopes, enhancing the versatility in applications. However, its efficiency heavily relies on the neutron flux and the properties of the material used for capturing.
Detection Techniques
Detection techniques for neutrons encompass methods that identify and monitor neutron emissions, crucial for assessing the operational state of neutron batteries. Various sophisticated detectors utilize materials that react with neutrons, producing measurable signals.
- Key Characteristics:
A major characteristic of these techniques is their capacity for real-time monitoring, ensuring safety and efficiency in neutron battery operation. - Advantages and Limitations:
While effective, they may face limitations based on the types of detectors used and the environments in which they operate.
A successful neutron battery hinges not just on the fission, fusion, or decay of neutrons but also on how effectively we can harness and manage them once produced.
Mechanism of Neutron Batteries
Understanding the mechanism of neutron batteries is paramount to appreciating their groundbreaking potential in the field of energy storage. The relevance of this section lies in its ability to dissect how these batteries operate, the intricacies involved in energy conversion, charge storage, and the overall efficiency considerations that make them a noteworthy alternative in energy technology.
Operating Principles
Energy Conversion
Energy conversion in neutron batteries entails the transformation of neutron energy into usable electrical power. This process hinges on the ability of device materials to efficiently capture and convert the kinetic energy of neutrons. A key characteristic of this conversion is its high efficiency rate compared to traditional battery technologies, which may waste significant energy during transformation processes. In the case of neutron batteries, the unique feature is the capability to directly utilize neutron emissions for energy through nuclear reactions, which is a game-changer for energy applications.
This mechanism is beneficial as it opens the door to harnessing a unique energy source that is not reliant on conventional chemical processes. However, the potential drawbacks include the need for specialized materials and shielding due to the radioactive nature of some neutron sources, which presents both an engineering challenge and safety concern. The constant innovation in materials science could address these disadvantages over time.
Charge Storage
Charge storage is another critical aspect of neutron batteries, playing a pivotal role in determining their usability in real-world applications. The key characteristic here is the method by which the system retains energy after conversion. Neutron batteries utilize advanced materials that can effectively hold and release energy, giving this system a significant edge over traditional lithium-ion designs.
One unique feature of charge storage in these batteries is the possibility of employing different types of mediators to enhance charge retention. Such mediators can lead to improved performance and stability, particularly during extended use. However, while these advantages are promising, they do come with their own set of challenges, such as increased costs and complexity in manufacturing. Nevertheless, ongoing research aims to refine these methods to improve practical applications in consumer electronics and large-scale energy systems.
Efficiency Considerations
Thermodynamic Efficiency
Thermodynamic efficiency is a pivotal concept when discussing neutron batteries. This factor denotes how much of the energy input can be converted into usable output. A standout point is that neutron batteries display improved thermodynamic efficiency over many conventional energy storage systems, largely because they leverage nuclear reactions rather than chemical ones. It suggests that more energy can be extracted per unit of fuel, making it a favorable choice for applications requiring high energy density, like military or space explorations.
However, contingencies arise due to technological constraints tied to heat management. Thermal energy from the reactions must be adequately dissipated to avoid system failures. Despite this, the incremental advancements within the field aim to harness this efficiency wholly.
Cycle Lifetime
Cycle lifetime refers to the number of charge and discharge cycles a battery can perform before its capacity diminishes significantly. For neutron batteries, the longevity aspect is an attractive feature, as they promise a longer cycle life due to the stability of nuclear reactions compared to their chemical counterparts. This characteristic can drastically reduce the frequency of replacements, which not only cuts down costs but also lessens the environmental impact associated with battery disposal.
Nevertheless, challenges exist. The materials used in the batteries may degrade over time due to radiation exposure, complicating the overall lifecycle of the product. Finding a balance between cycle life and performance remains a matter of intense research and development.
"The efficiency and lifecycle of neutron batteries might reshape our understanding and expectations of energy storage systems, particularly in high-demand scenarios."
Comparative Analysis with Conventional Batteries
Understanding how neutron batteries stand up against more traditional energy storage solutions is crucial. As we delve into this comparison, we will highlight technical differences, environmental impacts, and their implications for future energy solutions. The nuances observed in these discussions not only shine a light on the potential of neutron batteries but also foster an appreciation for their unique benefits and the challenges they present.
Technical Differences
Energy Density Comparisons
Energy density refers to how much energy a battery can store in a given volume or mass. In the realm of neutron batteries, their energy density is often cited as being substantially higher than that of conventional lithium-ion batteries. This characteristic is crucial as energy density directly correlates with how long a device can run before needing a recharge. A neutron battery's ability to store large amounts of energy without the bulk often required in more conventional systems makes it a desirable option in settings where space is limited.
For example, small satellites or remote sensing devices could benefit significantly from this aspect. High energy density means these devices could function longer without frequent recharges, which can be a real game-changer during missions. However, it is important to keep in mind that while the theoretical energy densities are impressive, practical applications must overcome hurdles related to energy conversion efficiencies and real-world constraints.
Longevity and Reliability
Longevity and reliability are fundamental factors that users consider when evaluating battery options. Neutron batteries are reputed for their lengthy operational life, often outpacing conventional options. In technical terms, a longer lifespan translates into fewer replacements, which is not only cost-effective but also a win for sustainability due to less waste.
One of the standout features of neutron batteries is their capacity to maintain performance over many cycles of charge and discharge. Whereas lithium-ion batteries tend to lose capacity after numerous cycles, neutron batteries show promise in retaining their effectiveness over time. This reliability is particularly appealing for applications in remote or critical fields, where battery replacement might not be feasible for extended periods. However, this potential longevity comes with a caveat regarding the initial higher costs involved in development and production.
Environmental Impact
Material Sourcing
Material sourcing is another pivotal concern in the discussion about battery technology. Neutron batteries can utilize materials that are, in essence, less environmentally disruptive compared to those required for conventional batteries. Traditional lithium-ion batteries rely on lithium and cobalt, both of which come from mining operations that can cause significant ecological damage.
Neutron batteries may, in contrast, leverage materials that are more abundant and less harmful to extract. For instance, using certain isotopes that can be sourced more sustainably can diminish the environmental footprint. Still, this is a developing area, and researchers need to navigate the nuances of sourcing these materials in a responsible manner. Thus, while the potential benefits stand out, the realities of material sourcing must also be critically assessed as technologies evolve.
Disposal Issues
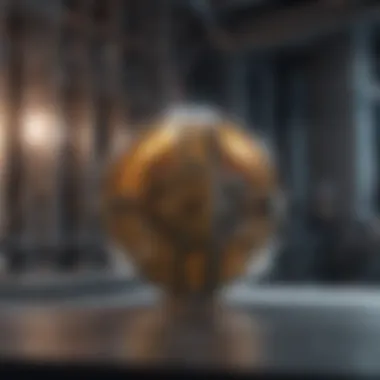
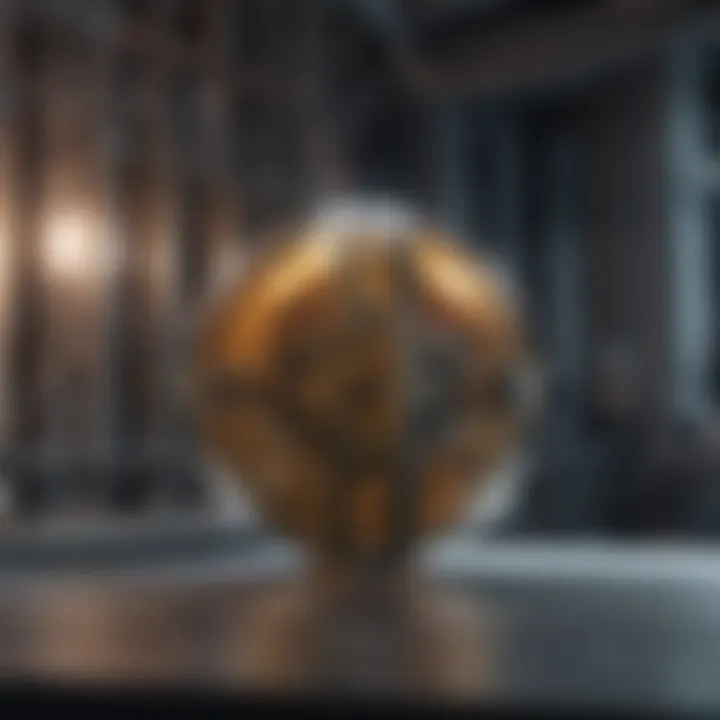
Disposal issues play a significant role in the lifecycle of any battery technology. Conventional lithium-ion batteries pose a considerable challenge at their end-of-life stage due to hazardous materials that necessitate careful handling and recycling processes. Contrarily, neutron batteries have the potential to present fewer disposal issues due to the types of materials they employ, which might be less toxic or easier to manage environmentally.
However, the technology is relatively nascent, and the complete implications regarding disposal procedures for neutron batteries are yet to be fully understood. Nevertheless, their design holds promise for more environmentally-friendly disposal options, which is an essential consideration as we move towards a sustainable future in energy storage.
Applications of Neutron Batteries
Neutron batteries hold promising potential across a variety of sectors due to their distinct energy storage capabilities. These batteries present unique advantages that can fundamentally alter the landscape of energy utilization. By harnessing the unique properties of neutron interactions, they can offer efficiencies and applications that traditional batteries simply cannot. Their reliance on neutron production provides robust energy delivery systems suitable for high-demand environments. The analysis of how they can be utilized across multiple domains is essential in understanding their role in future energy frameworks.
Military and Defense
Remote Operations
Remote operations are vital in military contexts where situations demand resilience and efficiency. The specific aspect of neutron batteries suited for this use is their ability to provide sustained energy without the weight and maintenance issues associated with conventional batteries. A key characteristic of these batteries is their capability to operate in various adverse conditions, making them a beneficial choice in the battlefield or for reconnaissance missions. A unique feature of neutron batteries is their compact design, which allows for easier transportation and deployment. This advantage translates into lower logistical challenges and reduces the need for frequent re-supply missions, a crucial factor in military operations.
Powering Autonomous Systems
Powering autonomous systems is another facet benefiting immensely from neutron battery technology. Neutron batteries can deliver steady energy to unmanned systems that operate far from conventional energy sources. The critical characteristic here is their ability to sustain longer missions without frequent recharging. This feature fosters operational flexibility for drones and other autonomous devices. However, the integration of neutron batteries also raises challenges. For instance, current technology may struggle with scalability, which is an aspect that still needs addressing for broader deployment in this context.
Commercial and Consumer Electronics
Portable Devices
In the realm of consumer electronics, portable devices are a primary candidate for neutron battery applications. These batteries promise to offer longer life spans and quicker recharge times compared to lithium-ion alternatives. A significant advantage of neutron batteries is their energy density, potentially enabling sleek designs without compromising performance. The unique feature of portability without sacrificing energy power makes them an appealing choice for manufacturers. Yet, challenges remain in adaptation and consumer acceptance, which could slow their entry into this competitive market.
Electric Vehicles
Electric vehicles benefit from advances in neutron battery technology by providing an alternative to traditional battery systems. The specific role of neutron batteries in this context is to offer faster charging options and greater driving ranges. A key characteristic here is their potential to maintain performance over repetitive charge cycles. This resilience could attract consumers looking for vehicles that offer convenience and efficiency. However, the initial costs associated with development and the integration of neutron batteries into existing infrastructure are challenges that need careful consideration before widespread adoption.
Industrial Applications
Grid Stabilization
Neutron batteries could play a critical role in grid stabilization by supplying a consistent energy flow that supports peak demands. The paramount advantage in this application is reliability, offering energy solutions that can rapidly respond to fluctuations in grid demand. The unique characteristic of neutron batteries delivering high power outputs without delays makes them an enticing solution for energy providers. Nevertheless, concerns regarding safety regulations and technological compatibility with existing grid infrastructures warrant further scrutiny.
Heavy Machinery
Heavy machinery is another area that could benefit from neutron battery applications, particularly in enhancing operational efficiency and reducing downtime. The specific aspect relevant here is their ability to provide high energy output in demanding conditions. A significant feature of neutron batteries is their durability, capable of withstanding stress without performance degradation. While this promising application could revolutionize industrial activity, it also raises questions about the cost implications and the necessary transition from established energy sources to neutron technology.
"Neutron batteries are poised to redefine multiple industries, from military applications to enhancing consumer convenience. Their implications for energy efficiency and sustainability are profound."
Understanding the full scope of neutron battery applications highlights not only their versatility but also the extensive research and development required to address associated challenges. This information shapes the conversation around the future of energy sources and highlights the transformative potential of neutron batteries both now and for years to come.
Current Research and Developments
Research into neutron batteries is gaining momentum, fueled by the potential these systems have to revolutionize energy storage solutions. With consistent advancements in technology, the exploration of neutron batteries offers the possibility of enhanced efficiency and sustainability. It is an exciting time where various institutions and researchers are diving into this field, probing the science behind neutron generation and manipulation. The relevance of this research cannot be overstated, as it could affect everything from military applications to daily consumer electronics.
Key Research Institutions
Across the globe, a number of top-tier research institutions are taking the reins in neutron battery investigation. Places like MIT and the Oak Ridge National Laboratory are putting effort into understanding how neutron-based systems can be optimized. These institutions often deploy state-of-the-art facilities and cutting-edge technology to explore new materials and configurations that could make neutron batteries not only feasible but also practical for everyday use. The collaboration among researchers, engineers, and industry partners enhances the scope and depth of these studies, showing how essential teamwork is in scientific exploration.
Innovative Technologies in Development
Technological advances are peppering the field of neutron batteries, particularly in innovative materials and microbattery configurations. Each component plays a crucial role in achieving the energy efficiency and longevity that these systems promise.
Advanced Materials
When it comes to advanced materials, they stand as a backbone for neutron battery production. The specific aspect driving innovation here involves the synthesis and application of materials that can withstand high-energy environments. For example, materials like boron nitride and lithium hydride are being researched for their unique properties that allow for enhanced neutron capture. These materials are not just foundational; they have a significant characteristic of thermal and chemical stability that positions them as a reliable choice in the manufacturing of neutron batteries.
The unique feature of advanced materials is their ability to provide structural integrity without compromising performance, yielding a balance of durability and efficiency. This characteristic makes them a popular pick among researchers who aim for a sustainable and efficient battery system. One potential drawback is that some advanced materials can be cost-prohibitive, which could slow down widespread adoption until economies of scale are achieved.
Microbattery Configurations
On the flip side, microbattery configurations bring to the forefront the evolving design of energy storage devices. The specific aspect here revolves around miniaturization and the ability to deliver high energy densities in small form factors. This becomes essential in applications where space is at a premium, such as in portable electronics. Microbattery configurations allow for the integration of neutron battery technology into compact devices without sacrificing performance.
A key characteristic of these microbatteries is that they often utilize thin-film technologies that enable efficient energy capture and release. Their design flexibility makes them extremely advantageous for novel consumer electronics and niche industrial applications. However, the challenge they face revolves around scalability—while it's one thing to create small batteries, producing them en masse can be a different kettle of fish. The further development of microbattery configurations is crucial for identifying viable solutions that can be produced consistently to meet market demand.
Innovation in the field of neutron batteries is not a solitary pursuit but a collective effort by researchers striving for breakthrough technologies that can redefine energy storage.
In summary, ongoing research and development in neutron battery technology, driven by institutions and innovations focused on advanced materials and microconfiguration designs, holds immense promise. By working through challenges and exploring newer ideas, scientists and engineers hope to establish neutron batteries as a mainstay in the future of energy solutions.
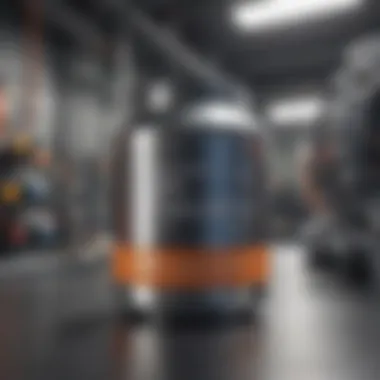
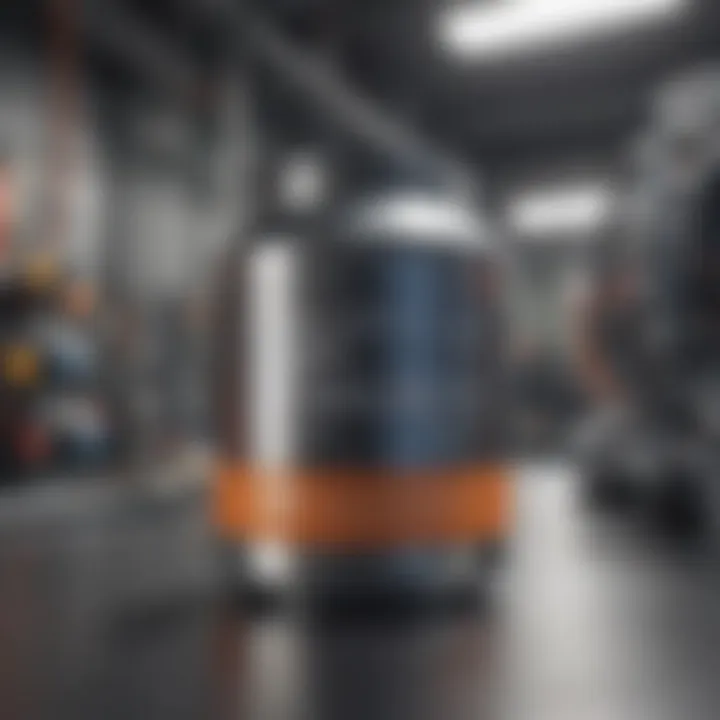
Challenges Facing Neutron Batteries
The rise of neutron batteries holds promise for transforming energy storage by leveraging advanced scientific principles. However, the technology faces significant hurdles that need to be addressed before it can be fully realized in commercial applications. From regulatory complexities to technological limitations, understanding these challenges is crucial for fostering innovation and developing viable solutions.
Regulatory and Safety Challenges
Neutron batteries, by their very nature, involve the handling and use of radioactive materials. This brings about a slew of regulatory hurdles meant to ensure public safety and environmental protection.
Governments around the world impose strict regulations on radioactive materials. These govern everything from the sourcing of materials to the final disposal of spent battery components. Navigating this intricate landscape demands comprehensive understanding and compliance from manufacturers. Moreover, the public perception of safety concerning radiation exposure can deter innovation. Keeping the public well-informed and assuring them of safety measures is paramount.
Ensuring that neutron batteries meet all safety standards is a high-stakes endeavor. Inadequate regulatory oversight could lead to accidents, which would pose serious risks. Achieving a compliance standard not only protects consumers but also enhances the credibility of neutron battery technology as a whole.
Technological Barriers
While the potential for neutron batteries is immense, several technological challenges must be overcome. Two primary facets of these barriers are cost efficiency and scalability.
Cost Efficiency
Addressing the complexities of cost efficiency in neutron batteries is pivotal. Developing these technologies requires substantial investment in sophisticated materials and research. Consequently, initial production costs can be markedly high compared to conventional batteries.
The key characteristic of cost efficiency lies in the ability to produce neutron batteries without incurring exorbitant expenses while maintaining quality. For the future of neutron batteries, finding a good balance between technological advancement and cost control is essential. If the costs can be brought down, widespread adoption becomes more realistic. It is notable that advanced materials often come with high price tags, posing a unique challenge.
Investing in cost efficiency can lead to lower prices for consumers, making neutron batteries a competitive alternative. Yet, the gamble for cheaper production could also result in sacrificing quality or safety in pursuit of reduced costs. Striking the right balance here is crucial.
Scalability Issues
Scalability remains another challenging aspect of neutron battery development. As the demand for energy storage solutions grows, being able to produce neutron batteries at a large scale is imperative. The process of scaling up from small laboratory setups to commercial production introduces many complications.
The uniqueness of scalability in this context relates to the need for both infrastructure and workforce capable of supporting large-scale manufacturing. It’s beneficial to note that scalability could allow companies to meet the growing demand for sustainable energy solutions. However, the production process must be efficient enough to allow this without heavy reliance on labor-intensive methods and substantial resource allocation.
While tackling scalability issues, the focus should remain on developing robust manufacturing practices that ensure quality alongside quantity. On the flip side, poorly executed scaling can lead to inconsistent product quality, which may undermine the credibility of neutron batteries.
"Ultimately, resolving both cost efficiency and scalability is integral not just for the feasibility of neutron batteries, but also for their longevity in a competitive energy landscape."
Addressing the multifaceted challenges of regulatory compliance and technological barriers is vital for the advancement of neutron batteries. Focusing on innovation while meeting safety requirements can pave the way for practical and larger-scale implementations of this emerging technology.
Future Prospects of Neutron Battery Technology
The future of neutron battery technology holds significant promise, particularly as it pertains to the evolving landscape of global energy needs. As energy demands increase, the exploration of alternative energy sources becomes paramount. Neutron batteries, with their unique principles and advantages, present a viable solution to some of the persistent challenges associated with conventional batteries. It is critical to examine how these systems may seamlessly integrate into our energy infrastructure while fostering sustainability and efficiency.
Integration with Renewable Energy Sources
As renewable energy continues to shine in the limelight, the integration of neutron batteries into this sphere could enhance the reliability of renewable systems. By harnessing the power of neutrons for energy storage, we can manage and stabilize energy fluctuations. The unpredictability of solar and wind energy often acts as a stumbling block, but neutron batteries could potentially smooth the ride, acting as a buffer that absorbs surplus energy and then discharges it during times of low generation.
For instance, consider solar power farms. On bright sunny days, these facilities generate excess energy that might go unused; with neutron batteries in place, this power could be stored efficiently. During cloudy periods or nighttime, the stored energy could be released into the grid, ensuring consistent supply. Thus, the synergy between renewable sources and neutron batteries could contribute to a more resilient energy ecosystem.
Potential Market Impact
The market impact of neutron batteries could be transformative. As industries and individuals seek more efficient and sustainable energy storage options, neutron batteries could capture a sizable segment of the energy storage market. Their potential to offer higher energy density and longevity compared to traditional options like lithium-ion batteries positions them favorably in the ever-competitive marketplace.
Moreover, as governments around the world emphasize clean energy and sustainable practices, the demand for innovative battery technologies is anticipated to soar. Neutron batteries could play a pivotal role in meeting regulatory requirements and sustainability commitments. For businesses, this presents an opportunity not only to comply with emerging energy regulations but also to enhance their public image by investing in cleaner technology.
Long-term Sustainability Outlook
Considering long-term sustainability, neutron batteries may contribute to a greener planet. Traditional batteries often involve resource-intensive mining processes, resulting in significant environmental degradation. In contrast, neutron batteries could potentially utilize nuclear reactions that minimize the environmental footprint, assuming safe and regulated implementations.
The long-term viability of neutron batteries must also assess their lifecycle management. If these systems can be designed with recyclability in mind, they could reshape how we think about energy storage. For example, developing methods to recycle neutron sources or components post-use could drastically reduce waste and resource consumption.
"The future of energy storage lies in our ability to innovate responsibly, and neutron batteries may exemplify such progress."
Closure
The conclusion of our exploration into neutron batteries serves as a crucial touchstone for both practitioners and theorists in the energy sector. It underscores not just the technical nuances and innovations associated with this technology, but also its broader implications for sustainability and efficiency in energy systems. Understanding neutron batteries becomes more than just an academic exercise; it is a window into the future of how we might store and utilize energy in an evolving landscape dominated by climate concerns.
Recap of Key Insights
In revisiting the key points covered in this article, it is essential to highlight several elements that define the relevance and promise of neutron battery technology:
- The unique principles of neutron production and charge storage that differentiate it from conventional battery systems.
- Its potential applications, particularly in sectors where reliability and energy density are paramount, such as military defense and electric vehicles.
- Challenges that accompany this emerging technology, including regulatory hurdles and technological barriers that must be surmounted for practical deployment.
- The prospects of integrating neutron batteries with renewable energy resources to enhance grid stability and energy efficiency.
This recap not only consolidates the information we've discussed, but also opens a pathway to consider how these insights will influence future research and development.
The Role of Neutron Batteries in Future Energy Systems
Looking forward, neutron batteries represent a significant potential shift in energy storage paradigms. They could provide solutions to several pressing issues faced by energy systems today:
- Energy Density: Neutron batteries may offer superior energy density compared to traditional batteries, making them an attractive option for applications requiring reliable, long-lasting power sources.
- Sustainability: By reducing reliance on lithium and cobalt, neutron batteries could mitigate some of the environmental concerns linked to current battery technologies.
- Integration with Renewables: As the world pivots toward renewable energy, neutron batteries might facilitate smoother transitions by storing energy generated from intermittent sources like solar and wind.