Plasmid Extraction: Methods and Challenges in Biology
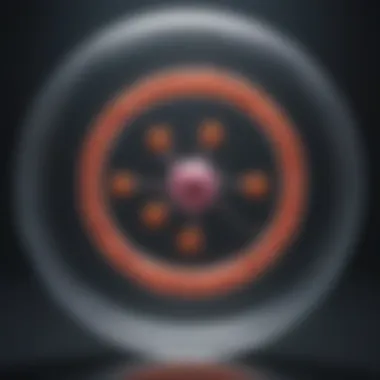
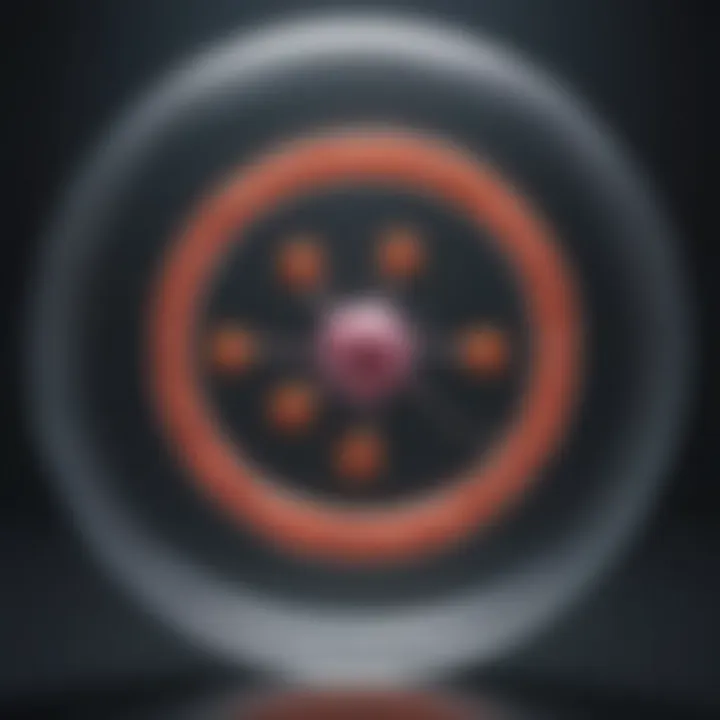
Intro
In the realm of molecular biology, the extraction of plasmid DNA stands as a pivotal task that plays a crucial role in diverse applications—from genetic engineering to cloning projects. Plasmids, which are circular DNA molecules distinct from chromosomal DNA, can carry genes beneficial for bacterial survival and growth. Understanding the nuances of plasmid extraction techniques is not just about the science; it's also about the subtleties in procedures that could influence outcomes significantly.
Research Background
Overview of the Scientific Problem Addressed
Plasmid extraction is not merely a routine laboratory technique. It addresses a significant scientific problem—the need for high-quality DNA that researchers can use reliably. Without efficient extraction, downstream applications like sequencing, gene expression studies, and transformation could yield erratic results. The quest is always for methods that optimize yield and purity, ensuring that the extracted DNA is in a usable form for experimental applications.
Historical Context and Previous Studies
Historically, plasmid extraction methods have evolved significantly. Early approaches included simple alkaline lysis, which, while effective, often resulted in contamination with genomic DNA and RNA. As the field advanced, modified protocols were introduced. For instance, the development of silica-based methods offered improved purity. Numerous studies have evaluated and compared these methods, and current research continues to focus on refining existing techniques for better efficiency and specificity.
Findings and Discussion
Key Results of the Research
Research has consistently shown that the choice of extraction method impacts both the quantity and quality of plasmid DNA obtained. For instance, studies comparing alkaline lysis and commercial kits often highlight that while kits can streamline the process, they might not always yield higher quality DNA. This nuance can be critical for experiments needing utmost precision.
Interpretation of the Findings
The repetition of such findings emphasizes that no single method fits all. Each technique caters to specific requirements and contexts, be it the scale of extraction or the intended application of the plasmid. The goal is often to strike a balance between ease of use, cost, and quality. For instance, while a more complex method could yield superior purity, it may not be practical for routine applications.
"Selecting the right plasmid extraction method is akin to choosing the proper tool for a job. The effectiveness of your results lies in understanding your objectives."
This quote encapsulates the essence of plasmid extraction methodologies. Researchers must evaluate the strengths and limitations of each method to determine which will serve their needs best.
In summary, plasmid extraction remains a cornerstone of molecular biology, interwoven into countless applications that drive innovation in research and biotechnology. Understanding its techniques, challenges, and practical applications enables researchers to make informed choices, ultimately leading to fruitful scientific endeavors.
Overview of Plasmids
Understanding plasmids is like giving oneself the blueprint to the cellular universe. These small, circular DNA molecules, found predominantly in bacteria, pack a powerful punch. Their unique structure allows them to replicate independently of chromosomal DNA, making them an essential tool in molecular biology. Plasmids serve not just as vehicles for genes but also play pivotal roles in gene expression, stability, and engineering applications.
When we talk about plasmids, we aren't just discussing any old fragment of DNA; we’re diving into a complex world teeming with possibilities. Their importance lies not only in their biological functions but also in their practical applications in biotechnology and medicine. For instance, plasmids can carry antibiotic resistance genes, which can contribute to the phenomenon of multi-drug resistance in bacteria. Recognizing these implications is crucial, especially for those immersed in genetic research or the development of novel therapies.
Definition and Structure
A plasmid is a small, double-stranded DNA molecule distinct from a cell's chromosomal DNA. Typically ranging from 1,000 to 100,000 base pairs in size, plasmids can vary significantly in structure. They are usually circular but can also exist as linear forms. The structure consists of a few essential components:
- Origin of replication: This is where the plasmid's replication starts.
- Selectable markers: These are genes that allow for the survival of cells containing the plasmid, often providing resistance to antibiotics.
- Multiple cloning sites (MCS): A short sequence containing several restriction sites to facilitate gene insertion.
The interplay of these components allows plasmids to perform effectively inside host cells, amplifying genes of interest while enabling researchers to select for successfully transformed cells.
Types of Plasmids
Plasmids aren't just one-size-fits-all; they come in various types, each with specialized functions that cater to different needs. Here’s a brief overview of some notable types:
- Conjugative plasmids: These plasmids enable transfer between bacteria through a process called conjugation. They are crucial for the spread of antibiotic resistance.
- Non-conjugative plasmids: Unlike their conjugative counterparts, these plasmids cannot move between cells unless assisted by other mechanisms.
- R plasmids (Resistance plasmids): Carry genes that provide resistance against antibiotics, playing a significant role in the challenge of treating bacterial infections.
- Col plasmids: Specifically produce colicins, which can kill other bacteria, thus giving their host a competitive advantage.
- Virulence plasmids: Contain genes that enhance the pathogenicity of the bacteria.
Understanding these types can aid researchers in selecting the right plasmid tailored for their project needs, whether for functional studies, gene therapy, or the development of new vaccines.
"The relevance of plasmids stretches far and wide in the realms of biotechnology and medicine, earning them their reputation as molecular workhorses."
By fleshing out the topic of plasmids through this lens, readers equipped with this knowledge can better appreciate the significance of plasmid extraction and the implications it holds in cutting-edge scientific research.
Importance of Plasmid Extraction
Plasmid extraction is not just a routine task in molecular biology; it stands as a foundational pillar that supports a broad spectrum of scientific exploration. The significance of this process radiates across various domains like biotechnology and academic research, underpinning the development of new therapies and the understanding of genetic mechanisms.
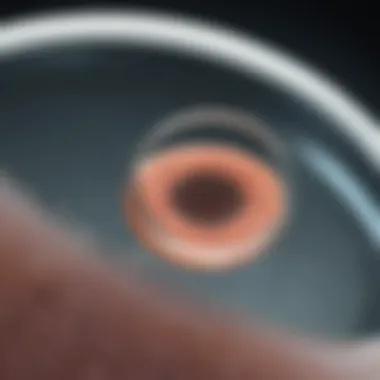
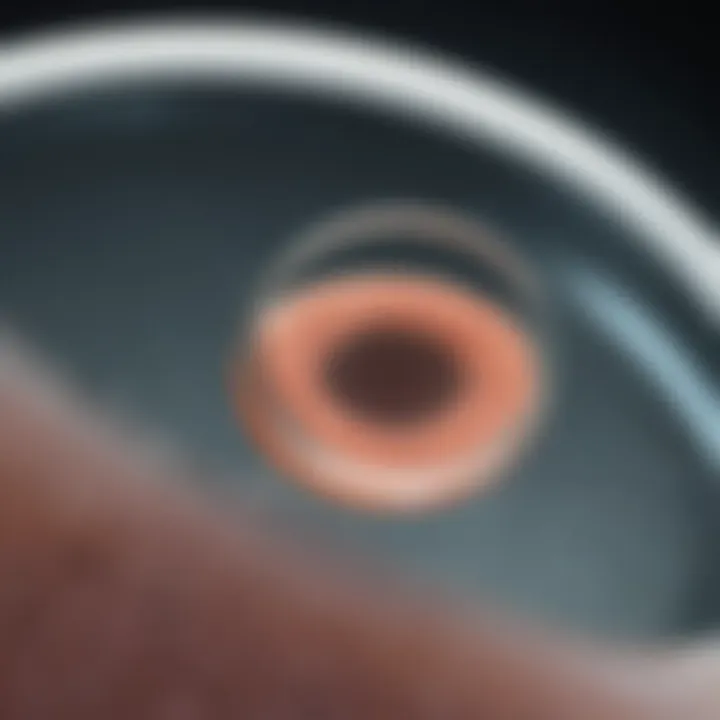
One aspect that highlights its importance is the biotechnological applications, where plasmids play a crucial role in genetic engineering. A well-extracted plasmid can carry genes of interest into host organisms, enabling the production of proteins or enzymes critical for advancements in medicine, agriculture, and industry.
Biotechnological Applications
Plasmids serve as vehicles for gene transfer in genetic modifications. This has revolutionized fields like medicine and agriculture. For instance, plasmid DNA is employed in vaccine development, particularly in the case of DNA vaccines, which offer a novel approach to immunization. The extraction process ensures that the plasmid is free of contaminants, which might hinder its effectiveness.
In agriculture, plasmids contribute to the development of genetically modified organisms (GMOs) that can withstand pests or harsh environmental conditions. The successful expression of desirable traits often hinges on the quality of the extracted plasmids. For example, Bt corn utilizes a plasmid-based gene to express a protein toxic to specific insects, thereby reducing the need for chemical pesticides.
Furthermore, plasmids are key in producing biopharmaceuticals, such as insulin and monoclonal antibodies, which have changed the landscape of diabetes management and cancer treatments. A clean extraction contributes to higher yields and purification of therapeutic proteins, essential for effective patient care.
Research Applications
On the research front, plasmid extraction opens doors to numerous scientific inquiries. Scientists rely on these plasmids for cloning, where they insert genes into plasmids that are then introduced into bacterial cells for replication. This allows researchers to study gene function and expression in controlled settings, aiding discoveries that could lead to groundbreaking treatments.
For example, the study of antibiotic resistance often employs plasmid extraction to isolate resistance genes from bacterial populations. These studies help understand how resistance spreads and what interventions might curb its rise.
Moreover, in the realm of synthetic biology, plasmids are engineered to construct complex biological systems that can produce new compounds or perform specific tasks. The extraction process must ensure plasmids maintain their integrity to function as designed, making the quality of plasmid extraction a critical factor in experimental success.
In summary, the importance of plasmid extraction cannot be overstated. It influences various sectors, enabling advancements in biotechnology and providing the tools necessary for extensive research. High-quality plasmids extracted using effective methods facilitate innovations that can ultimately reshape how we approach diverse challenges in health, agriculture, and the scientific community.
Methods for Plasmid Extraction
In the realm of molecular biology, methods for plasmid extraction play a pivotal role. The extraction of plasmids not only lays the groundwork for genetic analysis but also fuels advancements in various biotechnological applications. This section delves into the specifics of notable methodologies, laying bare their principles, step-by-step protocols, and the necessity of each technique in the broader context.
Alkaline Lysis Method
Principle
The alkaline lysis method stands out with its core principle rooted in differential solubility. This technique utilizes an alkaline solution to disrupt the cellular structures while simultaneously denaturing chromosomal DNA and proteins. The key characteristic that makes this method prevalent is its ability to selectively isolate plasmid DNA. By extracting plasmids through a simple yet effective chemical reaction, the alkaline lysis method is both efficient and cost-effective, making it a frequently chosen procedure in laboratories. The unique feature of this method is its rapid execution, often yielding plasmid DNA in just a few hours, though careful handling is critical to avoid contamination.
Step-by-step Procedure
The step-by-step procedure of this method is straightforward, dismantling the complexities often associated with DNA extraction. Begin with bacterial cultures, then proceed with cell lysis using an alkaline buffer. This lysis allows for the release of plasmid DNA. Following the lysis, neutralization is crucial, as it prevents the re-annealing of chromosomal DNA. The extraction’s simplicity is its hallmark - anyone familiar with basic laboratory techniques can undertake it without hesitance. However, while effective, the nuances of temperature and timing can greatly influence outcomes, warranting meticulous attention during each phase.
Limitations
However, it's essential to recognize the limitations of the alkaline lysis method. Though popular, it may suffer from low yield, particularly when dealing with larger plasmids or in cases of suboptimal bacterial growth. Another limitation lies in the potential for contaminants, which can adversely affect downstream applications. Furthermore, not all plasmids respond favorably to this procedure, particularly plasmids that exhibit high complexity or varying conformations.
Silica Membrane-Based Methods
Principle
Silica membrane-based methods leverage the binding properties of silica in the presence of high salt concentrations. The principle revolves around the selective adsorption of nucleic acids to silica, thus allowing for a purified extraction process. This characteristic serves as an attractive alternative to traditional methods due to its high efficiency in isolating plasmid DNA with minimal contaminants. Its unique feature is versatility; it can be applied to various cell types and plasmid constructs, making it adaptable in diverse laboratory settings.
Step-by-step Procedure
The procedure follows a clear path, an appealing feature for many practitioners. It starts with lysing the cells, followed by the addition of a binding buffer containing salt and sometimes additives that enhance DNA stability. The lysate is then applied to a silica membrane and subjected to washing buffers that eliminate impurities. The plasmid DNA can then be eluted with a low-salt buffer or water. The precision of this method is commendable, ensuring purity and quality, though one must be cautious about the binding conditions to maximize efficiency.
Advantages and Disadvantages
This methodology boasts several advantages, including high yields and the capability to work with low-volume samples. Nevertheless, its disadvantages can include the expense associated with proprietary extraction kits and the tedious nature of the method at times. The high cost may deter some laboratories from fully embracing these extraction kits, despite their effectiveness and reliability in yielding high-quality DNA.
Magnetic Bead-Based Methods
Principle
Magnetic bead-based methods pivot around the interaction of DNA with magnetic beads, allowing for a convenient extraction process. The principle is straightforward; once cells are lysed, DNA binds to the beads under specific conditions, which enables washing away of contaminants. The key characteristic of this method is the speed and efficiency with which plasmid DNA can be isolated, making it appealing for high-throughput needs.
Step-by-step Procedure
The stepwise approach here involves cell lysis, followed by the addition of magnetic beads. The mixture is then manipulated using magnets that attract the beads, allowing for the removal of unbound materials. This streamlined methodology is beneficial when time is of the essence. However, handling magnetic beads can require some finesse, especially in larger batch experiments, which sometimes leads to bead loss if not managed carefully.
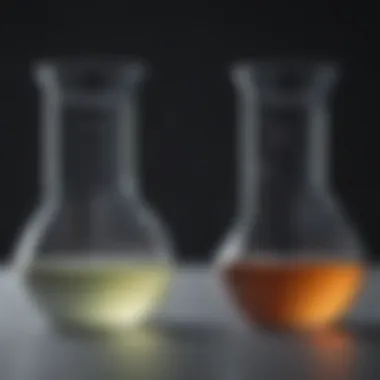
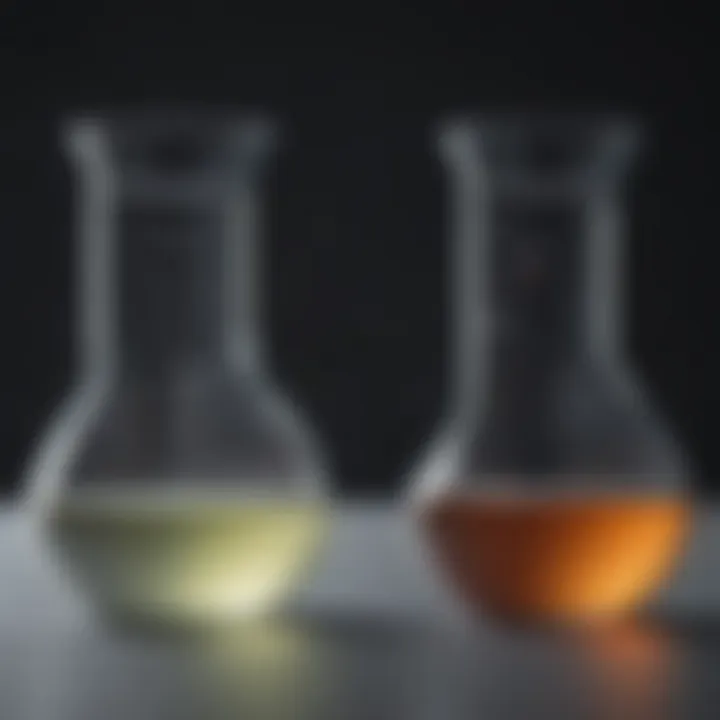
Comparison with Other Methods
When compared to other extraction techniques, magnetic bead-based methods provide a unique shift towards automation and ease of use. This can drastically reduce processing times and manpower, but the trade-off may come in the form of increased costs versus more traditional methods like alkaline lysis or silica membrane extraction. The method's unique aspect lies in its approach, which offers a fresh perspective for labs that value efficiency and quality, making it a worthy consideration among the extraction toolbox.
CTAB Extraction Method
Principle
The CTAB extraction method utilizes cetyltrimethylammonium bromide to precipitate nucleic acids, a unique feature among other common extraction methods. The principle is rooted in the ability of CTAB to selectively precipitate plant and pathogen DNA, creating a versatile tool in the extraction repertoire. This makes it particularly beneficial for samples that contain polysaccharides or secondary metabolites, which can interfere with DNA purity.
Step-by-step Procedure
In the CTAB method's procedure, starting with cell lysis using a CTAB buffer followed by a series of washes with chloroform and isoamyl alcohol can yield clean plasmid DNA. The key component is the CTAB, which selectively binds to proteins and polysaccharides, simplifying the purification process. Although effective, this method can be time-consuming with multiple washing steps, which could deter those pressed for time.
Use Cases
This method shines when dealing with complex samples, especially plant materials. The unique feature of CTAB extraction is its capability to handle challenging matrices, making it an invaluable technique in molecular biology. Many researchers favor this method when they detect difficulties using more straightforward techniques, understanding its effectiveness in diverse applications. However, one must be aware that the subsequent steps often entail more effort and can extend overall extraction time.
Quality Assessment of Extracted Plasmids
Assessing the quality of extracted plasmids is a cornerstone in ensuring successful downstream applications in molecular biology. Poorly extracted plasmids can lead to inefficient cloning, incorrect sequencing results, or failed transfections. This makes it paramount to adopt robust quality assessment methods to guarantee the integrity and suitability of plasmid DNA for various uses.
Agarose Gel Electrophoresis
Agarose gel electrophoresis is a cornerstone technique that allows researchers to visualize and assess the quality of plasmid DNA. This method not only separates nucleic acids by size but also provides insights into the purity and integrity of the DNA samples.
When performing agarose gel electrophoresis, plasmid samples are loaded into wells within an agarose gel matrix. An electric current is applied, causing the negatively charged DNA to migrate towards the positive electrode.
Key Benefits of Agarose Gel Electrophoresis:
- Size determination: Bands corresponding to different plasmid sizes help verify if the extraction process produced the desired plasmids.
- Purity assessment: If contaminants are present, they may show additional bands or smearing on the gel, indicating impure samples.
- Confirmation of supercoiled form: Supercoiled plasmids often have the highest transfection efficiency. This technique allows researchers to distinguish between supercoiled, open circular, and linear forms of DNA.
The results can be quantitatively analyzed as well, by comparing the brightness of the bands on the gel, often using software for image analysis. Furthermore, it is vital to include a DNA ladder or marker during the process for accurate size estimation.
"Visualizing plasmid integrity through agarose gel electrophoresis is akin to reading the roadmap of a journey yet to begin."
Spectrophotometric Analysis
Spectrophotometric analysis is another powerful tool to assess the quality of extracted plasmids. This technique typically involves measuring the absorbance of the DNA solution at specific wavelengths, primarily 260 nm and 280 nm. This method offers essential insights into both concentration and purity.
Key Considerations for Spectrophotometric Analysis:
- A260/A280 Ratio: This ratio is critical for determining protein contamination. Pure nucleic acids typically exhibit a ratio of around 1.8 for DNA. Ratios significantly lower than this suggest the presence of proteins or other contaminants.
- A260/A230 Ratio: This ratio helps in assessing contaminants such as phenol or other organic solvents. Values above 1.5 are generally acceptable.
- Concentration determination: Spectrophotometry provides a simple means to quantify the amount of plasmid DNA, essential for engaging in quantifiable experiments.
While spectrophotometric analysis provides quick feedback on the quality of extracted plasmids, it's essential to combine these data sets with results from techniques like agarose gel electrophoresis to form a comprehensive picture of the plasmid integrity.
Challenges in Plasmid Extraction
Extracting plasmids is not merely a procedural task; it’s a nuanced discipline fraught with potential pitfalls. The effectiveness of plasmid extraction greatly affects downstream applications such as cloning, sequencing, or synthetic biology projects. Thus, understanding the challenges involved is a prime necessity. These challenges can impact the purity and yield of extracted plasmid DNA, ultimately influencing research outcomes and biotechnological advancements. Below are key challenges that demand attention in this critical stage of molecular biology.
Contamination Issues
Contamination during the plasmid extraction process can spell disaster for any experiment. It’s like trying to bake a soufflé in a dirty oven—no matter how good your recipe is, the outcome will be less than desirable. Contaminants can originate from various sources, including the bacterial host, reagents, or even carelessness in handling samples.
Sources of Contamination:
- Bacterial Genomic DNA: Unwanted extraction of the host’s genomic DNA can complicate results.
- RNA Contamination: Leftover RNA can interfere with subsequent analyses.
- Protein Presence: Residual proteins can inhibit enzyme reactions crucial for manipulations that follow.
To mitigate these issues, strict adherence to sterile techniques, timely processing of samples, and the use of purification methods are essential. Regular blank runs can be useful as a precautionary measure to ensure that reagents remain uncontaminated before use.
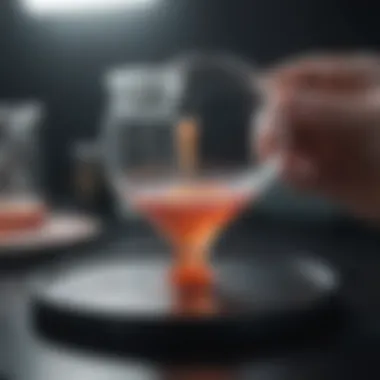
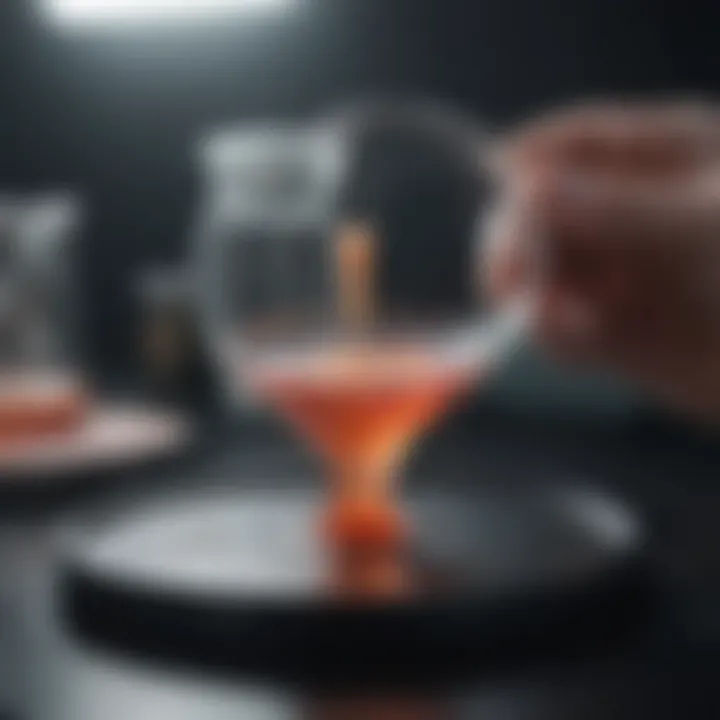
Yield Limitations
Yield limitations represent another significant hurdle. Sometimes, even after going through the entire extraction process meticulously, the yield of plasmid DNA falls short of expectations. Low yields can stymie research progress and lead to wasted time and resources. But the question arises: why do some extractions yield less plasmid than expected?
Factors Influencing Yield:
- Cell Density: Lower cell concentrations can result in lackluster yields of plasmid DNA.
- Extraction Method: Not all methods are created equal. Depending on the plasmid and bacterial strains, certain techniques may offer higher yields than others.
- Lysis Efficiency: Inadequate cell lysis leads to suboptimal recovery.
Strategies to enhance yield include optimizing the culture conditions prior to cell lysis, testing various extraction methodologies, and possibly pooling multiple extractions to concentrate the DNA.
Stability of Extracted DNA
The stability of extracted plasmid DNA also poses a significant concern, particularly when the intended use involves storage or further experimentation. Plasmid DNA should ideally remain intact and functional under various storage conditions. Factors influencing its stability include temperature fluctuations, exposure to light, and even the buffer solutions used for storage.
Recommendations for Stability:
- Short Term Storage: Typically at 4 °C for a few weeks, but longer durations are best avoided unless an appropriate buffer is used.
- Long Term Storage: For prolonged periods, temperatures at -20 °C or -80 °C are recommended to maintain integrity.
- Avoid Repeated Freeze-Thaw Cycles: Each thawing can subject plasmid DNA to degradation.
In summary, dealing with contamination, optimizing for higher yields, and ensuring the stability of extracted DNA are core challenges that must be critically addressed in the process of plasmid extraction. Understanding and addressing these obstacles will not only streamline research but will also enhance reproducibility and reliability of results, paving the way for more robust findings in molecular biology.
Best Practices for Effective Plasmid Extraction
Plasmid extraction is a process that, while straightforward in theory, can become daunting in practice without the right guidance. For those delving into molecular biology, understanding the best practices for effective plasmid extraction is crucial. It’s not just about the extraction itself, but ensuring that the resultant plasmid is of high purity and yield. With the growing reliance on plasmid DNA in research and biotechnology, the significance of these best practices cannot be overstated.
Sample Preparation
Before one even touches the reagents or begins the extraction protocols, the initial step of sample preparation holds immense importance. A well-prepared sample can set the tone for the quality of the extracted plasmid. This phase generally involves selecting the appropriate bacterial culture for plasmid isolation. The key here is to pick cultures that are in their exponential growth phase, as this maximizes the plasmid copy number.
- It's advisable to use fresh cultures, ideally under 24 hours old, because older cultures may enter a stationary phase, resulting in lower yields.
- Harvesting cells at the right time can significantly influence the final outcome. Too early or too late, and you may end up with compromised DNA.
Another critical consideration is the method of cell collection. High-speed centrifugation collects cells effectively, but equally it’s vital to ensure that the pellet is washed properly to remove any contamination. In some cases, using a wash buffer containing salts can reduce the likelihood of DNA degradation during further treatment. Prep goes a long way in ensuring success in plasmid extraction.
Optimizing Extraction Conditions
Once the sample preparation is out of the way, the next focus should be on optimizing extraction conditions. Each method — be it alkaline lysis, silica membrane-based methods, or magnetic bead techniques — has particular parameters that affect efficiency. Here are some factors to consider:
- Reagent Quality: Always use high-purity reagents. Impure chemicals can introduce contaminants, making your extracted plasmid unusable.
- Temperature Controls: Many reactions can be sensitive to temperature. Be sure that all incubations are performed at the correct temperature and that reagents are pre-conditioned as needed.
- pH Levels: Certain steps in the extraction process, such as lysis and neutralization, require specific pH levels for optimal results. Regularly check the pH of your buffers, as deviations can lead to insufficient lysis or DNA denaturation.
- Timing: Don’t cut corners on incubation times. Each protocol has been optimized to ensure maximum plasmid yield. Skipping steps or shortening times can lead to subpar results.
Translating these optimized conditions into practice can ensure reproducibility in your work.
"Good practices in plasmid extraction not only increase yield but can also enhance the stability of the extracted DNA, reducing issues downstream in applications such as cloning or sequencing."
By keeping a close eye on each of these elements, researchers can avoid some common pitfalls. It is this attention to detail that leads to successful plasmid extraction, paving the way for effective application in research.
Future Perspectives in Plasmid Extraction
As we look to the horizon of molecular biology, advancements in plasmid extraction present unique opportunities and challenges that can reshape research methodologies. The ability to effectively extract plasmids is foundational, impacting everything from basic research to clinical applications. Keeping abreast of innovations in extraction techniques can enhance the yield and purity of plasmid DNA, a necessity for ensuring the success of downstream applications. Moreover, recognizing the potential integration with cutting-edge technologies can revolutionize this field, pushing the envelope of what’s possible.
Innovations in Extraction Techniques
New extraction techniques are emerging that promise to optimize not just the yield but the quality of plasmids as well. One noteworthy development is the utilization of ultrasonication to disrupt cell membranes more efficiently than traditional methods. This energy-based approach can reduce sample processing time while maintaining the integrity of the plasmid DNA. Studies have shown that this method can lead to a substantial increase in purity levels compared to conventional techniques.
Another exciting advancement involves the use of enzymatic digestion specifically tailored to target bacterial cell walls. By customizing the enzyme cocktails, researchers can enhance lysis effectiveness, thus facilitating easier access to plasmid DNA. This precision has potential implications for applications requiring high-quality DNA, such as in synthetic biology and gene therapy.
"The landscape of plasmid extraction is evolving. Each innovation not only enhances yield but also the robustness of the processes employed in research and industry."
Additionally, miniaturized and automated systems for plasmid extraction are gaining traction. These systems reduce hands-on time and the risk of contamination while also allowing for high throughput processing, which is essential for large-scale projects. Integrating robotics into the extraction workflows can significantly enhance productivity, especially in laboratories that deal with numerous samples simultaneously.
Integration with Emerging Technologies
Plasmid extraction techniques are increasingly intersecting with emerging technologies. For instance, the integration of blockchain technology in laboratory processes is a topic gaining momentum. This could enhance traceability of samples, improve data integrity, and ensure compliance with regulatory standards, particularly in industries like biopharmaceuticals.
Furthermore, machine learning and artificial intelligence are starting to find their place in optimizing extraction conditions. By analyzing previously obtained data, AI models can predict optimal lysis conditions—factor in variables such as cell type, plasmid size, and extraction method. This could mean the difference between a mediocre yield and an excellent one, which is vital in competitive research environments.
The marriage of microfluidics with plasmid extraction is another frontier. This innovative approach allows for manipulation of small volumes for extraction, yielding time-efficient processes that can be particularly valuable in clinical settings where sample availability might be limited.
In summary, the future of plasmid extraction is bright, characterized by rapid advancements that promise to enhance every aspect of the process—from yield and purity to operational efficiency. Staying informed about these developments will empower researchers and professionals alike, allowing them to leverage state-of-the-art techniques to push the boundaries of what is currently achievable in molecular biology.