Analyzing the Plasmodium Life Cycle and Malaria Impact
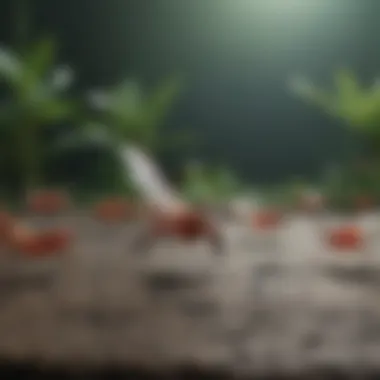
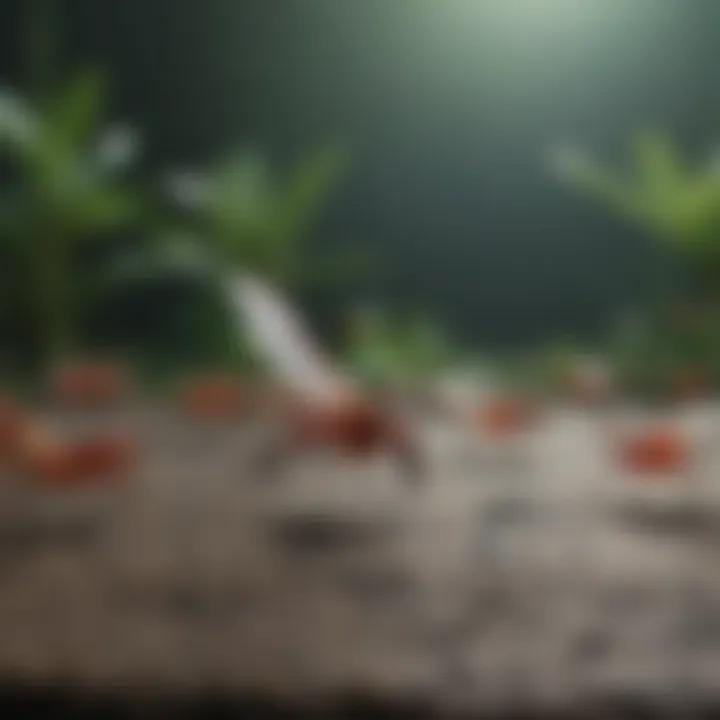
Intro
Malaria, a disease that has plagued humanity for centuries, stems from the complex life cycle of the protozoan known as Plasmodium. This organism, transmitted primarily through the bite of infected Anopheles mosquitoes, navigates a sophisticated journey from host to host, adapting and evolving at each stage. Understanding this life cycle is not merely an academic exercise; it holds significant implications for disease control and public health efforts around the world. By delving into the various phases Plasmodium undergoes in both human and mosquito hosts, we can uncover strategies to combat malaria effectively.
Research Background
Overview of the Scientific Problem Addressed
Malaria remains one of the most significant public health challenges, particularly in tropical and subtropical regions. Each year, millions suffer from the disease, mainly in regions where health infrastructure is already stretched. The key issue lies in understanding how Plasmodium completes its life cycle using two different hosts, the consequences of its development stages, and observing how these influence its transmissibility.
Historical Context and Previous Studies
Historically, the intricate interactions between Plasmodium and its vectors have been subjects of extensive research. The early 20th century witnessed groundbreaking discoveries like the identification of the mosquito as the vector for malaria. Over time, studies have revealed multiple species of Plasmodium, each with unique life strategies. Plasmodium falciparum, for instance, is the most deadly, and its life cycle intricacies have driven numerous studies aimed at unveiling its secrets.
This historical context sets the stage for a deeper exploration of the current findings regarding Plasmodium.
Foreword to Plasmodium
Understanding the life cycle of Plasmodium is a cornerstone of malaria research and public health strategies. This protozoan pathogen serves as the sole agent behind this life-threatening disease, which continues to afflict millions globally. As we peel back the layers of its biological intricacies, we uncover not just the mechanics of its reproduction and transmission but also the implications for disease control efforts. Knowledge of Plasmodium's lifecycle enriches our comprehension of the disease it induces, ultimately steering prevention and treatment advances.
Overview of Malaria
Malaria is not merely a health condition; it is a complex socio-economic dilemma that unfolds in many parts of the world. Each year, it accounts for thousands of deaths, particularly among children under five and pregnant women. The symptoms may start off creeping, like a slow-burning candle, beginning with fever, chills, and fatigue. Left unchecked, it can spiral into severe complications such as organ failure and death.
One might throw out the phrase that "an ounce of prevention is worth a pound of cure," which resonates profoundly in this context. Efforts to combat malaria traditionally focus on vector control, such as using insecticide-treated bed nets and indoor spraying, and early diagnosis and treatment. But to truly tackle the beast that is malaria, one must grasp how Plasmodium operates within its hosts.
The Plasmodium Genus
The Plasmodium genus consists of several species, each contributing to the tapestry of malaria’s impact. Notable among these are Plasmodium falciparum, Plasmodium vivax, Plasmodium ovale, and Plasmodium malariae. These species differ not only in their geographic distributions but also in their pathology and immune response evasion tactics.
For instance, Plasmodium falciparum is predominately associated with severe cases due to its rapid multiplication and ability to affect all blood stages. In contrast, Plasmodium vivax is recognized for its dormant liver stages which can reactivate, leading to relapses of malaria symptoms long after the initial treatment.
It’s through studying the distinguishing features of these species that researchers can forge pathways toward targeted vaccines and treatments. Each species presents its own set of challenges and lessons that highlight the adaptability of Plasmodium. To navigate ahead, an intimate knowledge of these variants is indispensable both for researchers and public health professionals alike.
Understanding the Life Cycle
The life cycle of Plasmodium is a journey that underscores the complexity of its existence and its relationship with its hosts. Understanding this cycle is essential, not just from a biological standpoint, but also in terms of how we approach malaria control and prevention. The life cycle can be likened to an intricate dance, each step meticulously choreographed to ensure the survival and proliferation of the parasite.
This section will highlight several elements critical to grasping the significance of the life cycle:
- Biological Complexity: The stages that Plasmodium undergoes both in the mosquito and human hosts reveal a fascinating array of adaptations and changes. A deep understanding allows researchers and public health officials to identify potential points of intervention in the life cycle that could mitigate malaria transmission.
- Transmission Dynamics: By grasping how Plasmodium navigates between its hosts, we can better understand its transmission pathways. Every stage of the life cycle plays a role in how effectively malaria can spread, emphasizing the need for public health strategies that target these specific moments.
- Infection Mechanisms: The life cycle also gives insight into how Plasmodium infects its hosts, resulting in significant implications for treatment and prevention. Recognizing these mechanisms can inform vaccine development and treatment protocols.
In essence, the life cycle is not merely a sequence of events; it is a crucial framework that underpins all efforts against malaria.
Defining Life Cycle Stages
The life cycle of Plasmodium is broken down into distinct stages, each playing a pivotal role in its development and transmission. These include:
- Sporozoite Stage: This is where it all begins. After a mosquito bite, the sporozoites travel to the liver of the human host. This stage primarily focuses on survival and readiness to infect liver cells.
- Liver Stage Development: Once inside the liver, the sporozoites mature into liver stages, multiplying and preparing for the next phase of the cycle.
- Erythrocytic Stage: The matured forms then enter the bloodstream, invading red blood cells. Here, they continue to replicate, leading to the symptoms of malaria as the host's immune response kicks in.
- Gameotocyte Formation: Some merozoites will develop into gametocytes, the sexual form of the parasite, which are crucial for transmission back to mosquitoes when they bite an infected human.
- Mosquito Stage: This involves the sexual reproduction of Plasmodium in the mosquito's gut, leading to the formation of oocysts and eventually releasing new sporozoites, ready to initiate the cycle anew.
Through these stages, comprehension of each phase allows researchers to pinpoint where intervention strategies, including pharmaceuticals and vaccines, can be implemented.
Importance of the Life Cycle in Transmission
The life cycle of Plasmodium is instrumental in understanding transmission dynamics. Each stage has unique implications for how the parasite is spread, influencing everything from epidemiology to public health initiatives.
- Vector Transmission: The reliance on the Anopheles mosquito as a vector highlights the life cycle's complexity. If we grasp how mosquitoes become infected and subsequently infect humans, we can tailor more effective vector control strategies.
- Human Infection Pathways: Understanding the specific life cycle stages that Plasmodium undergoes in humans helps in crafting targeted interventions, reducing human susceptibility.
- Survival Adaptations: The adaptability of the parasite throughout its life cycle raises questions regarding resilience and the likelihood of developing strategies that can combat these adaptations.
In summary, the life cycle of Plasmodium does not merely constitute a series of biological events; it is a critical element that dictates how malaria is transmitted and how we can strategize for its prevention and control.
Stages of the Life Cycle in the Mosquito Host
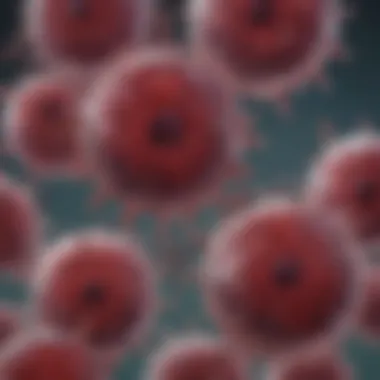
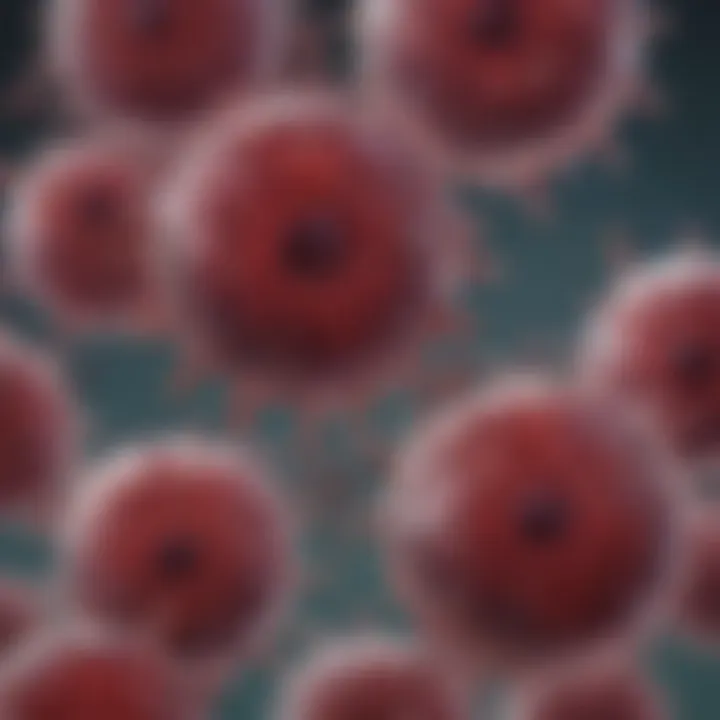
The life cycle of Plasmodium is a complex dance that takes place in both the mosquito and human hosts, but the stages occurring in the mosquito are critically important. Understanding the role of the mosquito—primarily the Anopheles species—offers insights into how malaria is transmitted and what can be done to interrupt that process. The dynamics of this life cycle are fundamental in crafting effective control strategies against malaria.
Foreword of the Anopheles Mosquito
Anopheles mosquitoes serve as the primary vectors for the Plasmodium parasites. These insects are not just any ordinary mosquitoes; they exhibit specific behaviors and habitats that facilitate the lifecycle of the parasite. Female Anopheles mosquitoes are the only ones that bite humans to obtain blood, necessary for their egg development. The choice of a host, the timing of feeding, and even the environmental conditions can influence their role in malaria's transmission.
The interaction starts with the female mosquito landing on the skin of a host—often at night, thanks to their crepuscular feeding habits. They have specialized mouthparts that enable them to pierce the skin and draw blood. This seemingly simple act sets off a chain reaction crucial to the survival and reproduction of Plasmodium.
Sporozoite Stage
When a female Anopheles bites an infected human, she ingests the gametocytes circulating in the bloodstream. Within the mosquito's midgut, these gametocytes undergo fertilization, leading to the development of zygotes and then to ookinetes. It is this stage of the lifecycle that transforms these cells into sporozoites.
The sporozoites migrate through the mosquito’s body and accumulate in the salivary glands. This is no small feat – it is a necessary step for transmission, allowing the parasite to move from one host to another. When an uninfected Anopheles bites a human afterward, these sporozoites are injected into the bloodstream, initiating the cycle once more.
Oocyst Formation
Oocyst formation marks the next crucial stage in the mosquito. After fertilization, the ookinetes penetrate the mosquito's midgut epithelium and begin to form oocysts, which are sac-like structures that house the developing parasites. This stage can take about ten days, depending on the temperature and overall conditions. In fact, temperature is an important factor in determining the speed at which oocysts mature.
Inside these oocysts, sporozoites begin to multiply asexually, going through multiple divisions. By the time they ready to break free, thousands of sporozoites can emerge from a single oocyst. This proliferation not only enhances the potential infectivity of the mosquito but also showcases Plasmodium's ability to adapt and thrive in its vector host.
Release of Sporozites
Finally, after completing the development within the oocysts, it's showtime. The mature oocysts burst, releasing the sporozoites into the mosquito's hemolymph—a bloodstream equivalent for insects. The timing of this release is crucial; optimal conditions ensure that sporozoites travel to the mosquito's salivary glands, where they await their chance to infect another human host.
The process of mosquito feeding becomes a matter of life or death for both the mosquito and the parasite, highlighting the intimate connection in their life cycles.
Thus, armed with knowledge of these stages, researchers and health professionals can devise better strategies to break this infectious cycle and combat malaria effectively.
Stages of the Life Cycle in the Human Host
Understanding the stages of the malaria parasite's development within the human host is crucial. It unveils how Plasmodium orchestrates its life cycle through specific adaptations that enhance its survival and proliferation. Grasping these stages sheds light on the mechanisms underlying the disease's transmission and offers insight into potential intervention points for treatments and vaccines.
Infection via Mosquito Bite
The story begins with the bite of the female Anopheles mosquito. As it takes a blood meal, the mosquito injects Sporozoites, the infective form of Plasmodium, into the bloodstream. This marks the initiating step of infection. Each mosquito can carry thousands of these microscopic parasites, effectively turning them into needle-like delivery systems for Plasmodium. When these Sporozoites enter the bloodstream, they make a beeline for the liver, where their next transformation begins.
It’s no coincidence that this phase is particularly opportunistic. The incident of a mosquito bite is often considered a routine occurrence; however, it carries the potential to initiate a significant health crisis. The rapid and almost stealthy way in which Sporozoites invade liver cells highlights the delicate and perilous interaction between host and pathogen.
Liver Stage Development
Once inside the liver, the Sporozoites undergo a fascinating transformation during what is referred to as the liver stage. In a span of about 7 to 10 days, these Sporozoites grow and multiply within liver cells, transforming into another form known as Merzoites. By the end of this developmental phase, a single Sporozoite can give rise to thousands of Merzoites. This exponential increase in parasite numbers primes the stage for the subsequent erythrocytic stage.
The liver’s unique microenvironment allows Plasmodium to become a master at evading the immune system. Liver cells (hepatocytes) are designed to store and metabolize various substances, and by exploiting this environment, Plasmodium can quietly grow without triggering an immediate immune response. This is what makes liver stage development a critical component of the infection cycle.
Erythrocytic Stage
Once Merzoites are released back into the bloodstream, they swiftly target red blood cells, marking the start of the erythrocytic stage. Inside these red cells, Merzoites transform into Trophozoites. These Trophozoites consume hemoglobin for nutrition and further develop into Schizonts, where the parasites replicate. Eventually, Schizonts burst, releasing more Merzoites and causing the infected red blood cells to lyse, leading to symptoms characteristic of malaria - fever, chills, and anemia.
The frequency at which infections recur is due to this dynamic behavior. The cycle can repeat every 48 or 72 hours, depending on the species of Plasmodium. Each burst of Merzoites results not just in more parasites but also releases toxins into the bloodstream, exacerbating the symptoms and potentially leading to severe complications for the host.
Merozoite Release and the Cycle of Infection
The final step in the life cycle within the human host is the continual release of Merzoites into the bloodstream. This is a carefully synchronized process that maximizes infection potential. As previously mentioned, the lysis of red blood cells leads to the release of hundreds of new Merzoites, maintaining the cycle.
Each time Merzoites enter the bloodstream, they have the opportunity to infect new red blood cells, thereby perpetuating the life cycle of Plasmodium even further.
Understanding this repetitive cycle is vital in controlling malaria. It's crucial for researchers and health authorities to pinpoint where intervention can occur to disrupt these stages effectively, be it through antimalarial drugs or vaccines aimed at preventing the initiation of infection altogether.
In summary, the stages of Plasmodium's life cycle within the human host are not just interesting biological events but are part of an acute survival strategy that maximizes the pathogen’s opportunity to multiply and spread, impacting healthcare on a global scale.
Morphological Changes Throughout the Life Cycle
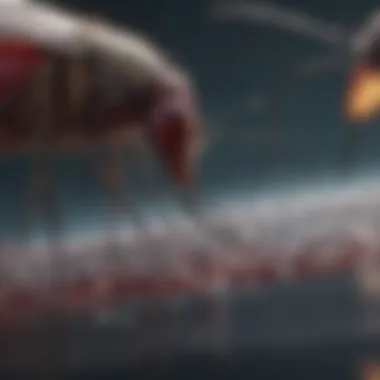
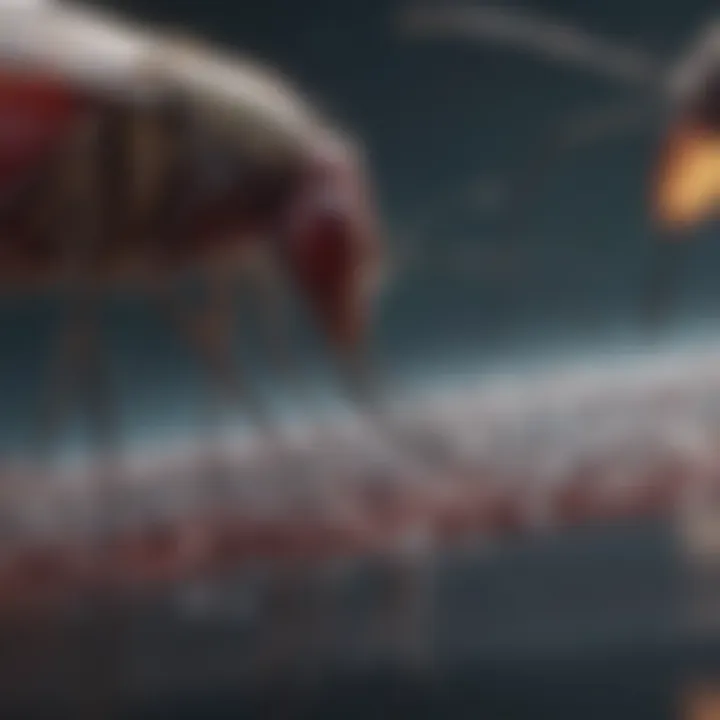
Understanding the morphological changes that occur throughout the life cycle of Plasmodium is crucial for comprehending how this protozoan parasite survives, adapts, and effectively transmits between its hosts. These changes are not merely a coincidence; they are evolutionary adaptations that enhance the parasite's ability to navigate its complex life cycle. Each morphological stage plays a significant role in the parasite's interaction with both the mosquito and human hosts, which is essential for its proliferation and ultimately its survival.
The life cycle of Plasmodium comprises distinct stages, each marked by notable morphological features. These alterations have profound implications regarding transmission dynamics, immune evasion strategies, and potential targets for drug intervention. By closely examining these stages, one can appreciate how Plasmodium has fine-tuned its biology to maximize its chances of transmission.
Sporozoites to Merozoites
The transition from sporozoites to merozoites is one of the most critical transformations in the life cycle of Plasmodium. Sporozoites emerge from the mosquito's salivary glands and enter the human bloodstream during a bite. Their shape is elongated and designed for motility, enabling them to efficiently migrate through the bloodstream to the liver.
Once in the liver, sporozoites undergo significant morphological changes, developing into liver stages known as hypnozoites or schizonts, depending on the species of Plasmodium. During this phase, they enlarge and multiply through several asexual divisions, producing thousands of merozoites. This process is akin to a factory churning out products for distribution.
Here are some key points concerning this transformation:
- Morphological Adaptation: The shift allows for an increase in the volume of the parasite without significant energy expenditure, which is vital for survival in a nutrient-scarce environment.
- Evasion of Host Immune Response: The liver stages can evade the immune system, as they remain largely intracellular during this time. They exhibit varying surface proteins that help them dodge detection by host immune cells.
"The ability of Plasmodium to morphologically adapt at different life cycle stages is one of its greatest evolutionary advantages."
Merozoites then burst forth from the liver cells, re-entering the bloodstream to invade erythrocytes, where yet another series of morphological adaptations will occur.
Trophozoites and Schizont Formation
Once merozoites invade red blood cells, they undergo further morphological changes, transitioning into trophozoites. This stage is distinguished by a change in shape; trophozoites appear amoeboid and actively consume hemoglobin from the erythrocytes, which is critical for their growth and multiplication. Within the infected red blood cells, the parasites continue to develop until they reach the schizont stage, where they prepare to produce a new generation of merozoites.
- Trophozoite Characteristics: The amoeboid shape is not just for looks; it facilitates movement within the crowded environment of red blood cells and aids in nutrient absorption.
- Schizont Formation: As the trophozoite's size increases, it divides asexually within the red blood cell, forming a multinucleated schizont. This stage is crucial as it represents the final step before the release of new merozoites.
This intricate cycle of morphological changes underscores the important relationship between parasite form and function. Each transformation plays a strategic role in the life cycle, allowing Plasmodium to colonize hosts effectively, evade immune defenses, and ensure its persistence in a broad range of environments. Understanding these changes can aid in the development of targeted interventions to disrupt the life cycle of this notorious pathogen.
Interactions Between Host and Pathogen
The interactions between the host and Plasmodium are pivotal in understanding the pathology of malaria. The relationship is a classic tug-of-war, where both parties exert influence over the other. The host's immune response acts as a frontline defense, while Plasmodium, in turn, has evolved sophisticated mechanisms to evade this defense. Recognizing these dynamics is essential for developing effective interventions and control strategies.
Immune Response in Humans
When a human is bitten by an infected mosquito, Plasmodium invades the bloodstream and initiates an intricate dance with the immune system. The first line of defense consists of innate immunity, where various cells, including macrophages and neutrophils, rush to the site of entry. They attempt to engulf and destroy the invading parasites. However, Plasmodium has its tricks up its sleeve. It can manipulate host cells to avoid detection and can rapidly replicate within the liver and red blood cells before the immune system mounts a significant response.
- Adaptive Immune Response: After the initial encounter, the adaptive immune response kicks in. This system generates antibodies specific to Plasmodium antigens, primarily focusing on the proteins expressed on the surface of infected cells.
- Role of T Cells: T cells, both CD4+ and CD8+, are crucial in controlling infections. They help in orchestrating a more robust and targeted immune response, especially during the erythrocytic stage of Plasmodium.
Despite these defenses, the immune response can be paradoxically detrimental. The intense immune reaction leads to symptoms such as fever and anemia, creating a vicious cycle that can undermine the host's health.
Quote: “The body’s attempt to fight Plasmodium often leads to the very symptoms that define malaria: fever, chills, and fatigue.”
Adaptation of Plasmodium to Host Defenses
Plasmodium’s ability to adapt is impressive, making it a formidable foe. Throughout its evolutionary history, the protozoan has acquired a series of evasion strategies to mitigate the impacts of the host immune response. Understanding these mechanisms is critical in the ongoing battle against malaria.
- Antigenic Variation: One notable strategy is antigenic variation, where Plasmodium changes its surface proteins to confuse the immune system. This makes it challenging for antibodies to identify and neutralize the parasite.
- Modulation of Host Responses: Additionally, Plasmodium can influence the host's immune responses. By secreting effector molecules, the parasite can alter cytokine profiles, further dampening the immune attack.
- Latency: The creation of hypnozoites during the liver stage allows Plasmodium to evade detection temporarily. This capability complicates treatment because the parasite can remain dormant for an extended duration, leading to relapses of malaria.
Both the immune responses in humans and the adaptive traits of Plasmodium present complexities that define the ongoing struggle between these organisms. Continued research into these interactions is essential for developing effective therapies and ultimately controlling malaria transmission.
Transmission Dynamics
Transmission dynamics is a cornerstone of understanding the lifecycle of Plasmodium, the protozoan responsible for malaria. Grasping how Plasmodium spreads from host to host not only unveils the biological processes at play but also provides insights into effective control strategies. By delving into the intricacies of transmission dynamics, we can vastly improve our approach to combating this ancient yet persistent disease.
Vector Competence of Mosquitoes
When discussing Plasmodium's transmission, one cannot overlook the role of mosquitoes, particularly the Anopheles species. These tiny creatures, while often dismissed as mere nuisances, are pivotal vectors, acting as both hosts and facilitators in the lifecycle of these parasites.
Understanding vector competence refers to the ability of these mosquitoes to carry and transmit Plasmodium. Not every mosquito is worthy of the title "vector." Factors such as genetic makeup, physiology, and even the environment contribute to this competence.
- Species Variation: Not all Anopheles mosquitoes are equal. Research shows that specific species like Anopheles gambiae are more capable of transmitting malaria than others like Anopheles stephensi.
- Infectivity Rates: Interestingly, the rate at which these mosquitoes can carry the parasites post-infection can vary widely. A distinct genetic predisposition influences how well their bodies handle the Plasmodium during its developmental phases.
- Feeding Behavior: Mosquitoes also differ in their feeding habits. The frequency and timing of blood meals drastically influence the likelihood of transmitting Plasmodium. Mosquitoes that prefer to feed at night may pose a greater risk, particularly when humans are more likely to be exposed.
Environmental Factors Influencing Transmission
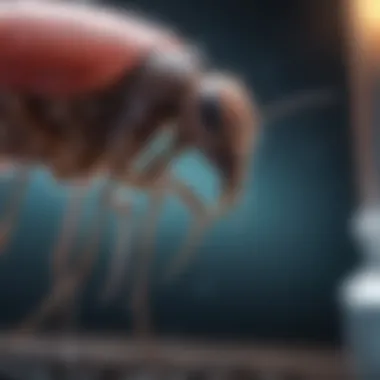
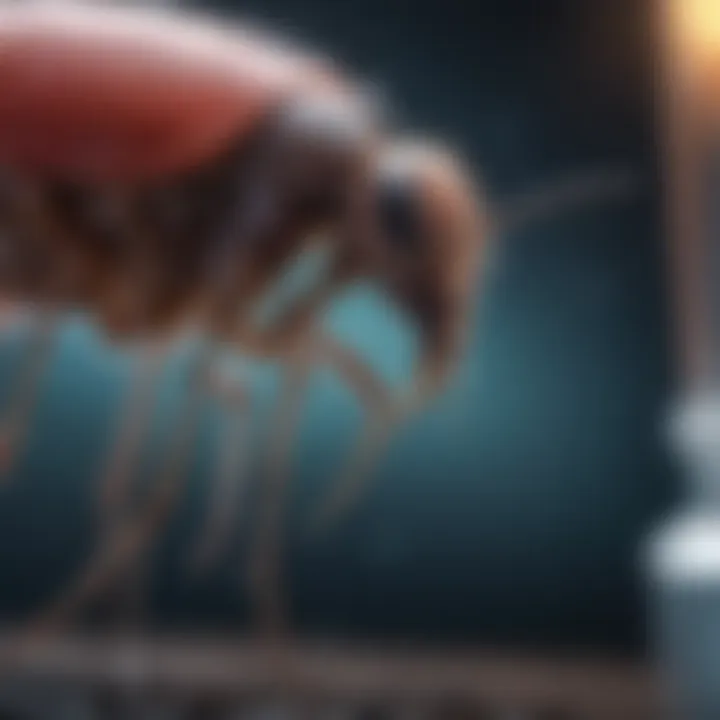
The backdrop against which malaria transmission occurs is complex and multifaceted. Environmental conditions can either facilitate or hinder the lifecycle stages of Plasmodium and its mosquito vectors.
- Climate: Temperature acts as a double-edged sword. Warm conditions may accelerate the development of Plasmodium within the mosquito and the rapid increase in mosquito populations. However, extremes—like heat waves or harsh cold—can reduce habitats where mosquitoes breed.
- Rainfall: Water is both an enabler and a threat. Areas with stagnant water pools provide breeding grounds for mosquitoes. Yet, excessive rainfall can flood these habitats, washing away larvae and pupae.
- Urbanization: As populations shift toward urban centers, the environment changes. Urban spaces may have pockets that either lend themselves to mosquito breeding or mitigate it altogether, influencing Plasmodium's spread.
Overall, the interplay between vector competence and environmental factors intricately weaves the narrative of malaria transmission dynamics. Without dissecting these layers and understanding the underlying mechanisms, addressing the malaria epidemic becomes a daunting task. Successful control methods hinge on this knowledge; appropriate interventions can then be crafted to disrupt transmission at various points in the cycle, aiding in the fight against malaria.
"Understanding how and why Plasmodium spreads is essential in making strides toward controlling malaria effectively."
This insight can potentially save countless lives and allow communities to regain their health with informed measures.
Controlling Malaria: Challenges and Strategies
Malaria remains one of the most pressing global health issues, and effectively controlling it poses considerable challenges. Despite existing methods, the Plasmodium parasite has shown remarkable resilience, making it imperative to continually evolve our approach to malaria management. This section sheds light on the importance of controlling this disease, emphasizing both the obstacles faced and the strategies employed to mitigate its impact.
Current Control Methods
Several control measures are already in place aimed at reducing malaria transmission and prevalence. Some of the most utilized methods include:
- Insecticide-Treated Nets (ITNs): These have become a frontline defense for households, particularly in high transmission areas. Beds draped with ITNs help to protect individuals from nocturnal mosquito bites, thus limiting exposure to the parasite.
- Indoor Residual Spraying (IRS): Spraying homes with long-lasting insecticides can significantly lower the mosquito population. This method, however, must be managed carefully to prevent resistance among mosquito populations.
- Antimalarial Medications: Treatment protocols such as artemisinin-based combination therapies (ACTs) are essential for managing infections once diagnosed. Timely treatment helps prevent severe disease and mitigates the risk of transmission to others.
- Health Education Campaigns: Empowering communities through awareness initiatives plays a crucial role in combating malaria. Educating individuals about symptoms, prevention, and the importance of early treatment can lead to reduced transmission rates.
"Prevention is better than cure," and this quote resonates profoundly in the fight against malaria. It's crucial not only to control infections but also to stop them before they occur.
Innovative Research Directions
Ongoing research is pivotal in finding new ways to combat malaria. Here are some exciting advancements in this field:
- Vaccine Development: The ongoing quest for an effective malaria vaccine has shifted in recent years, with several candidates moving into pivotal clinical trials. This area of research holds the promise of a powerful tool that can offer long-term protection against malaria.
- Gene-Editing Technologies: With tools like CRISPR, researchers are looking to manipulate mosquito genomes to reduce their ability to transmit Plasmodium. This innovative approach not only holds potential for controlling mosquito populations but also influences their capacity to spread the disease.
- New Insecticides and Mosquito Traps: Continuous development and testing of novel insecticides and traps could address the challenge of insecticide resistance faced by current control measures. Some of these innovations showcase biodegradable and environmentally friendly options that pose minimal threat to ecosystems.
- Proactive Research on Environmental Impact: Understanding how climate change and habitat alterations influence mosquito breeding patterns is vital. Research into these environmental factors can help anticipate shifts in malaria transmission
In summary, controlling malaria involves a multifaceted approach, marrying existing methods with innovative research to draft a comprehensive strategy. While challenges abound in this ongoing battle, the marriage of solutions stood strong against Plasmodium presents avenues for optimism in future malaria control efforts.
Future Perspectives
As we look ahead at the ongoing battle against malaria, the insights garnered from studying Plasmodium’s life cycle present a roadmap for future strategies. This segment serves to underscore critical elements regarding vaccine development and genomic studies, both of which are pivotal for enhancing our understanding and control strategies for malaria.
Vaccine Development
The quest for an effective malaria vaccine has spanned decades with various challenges that stem from the complex life stages of Plasmodium. Current vaccine candidates, like RTS,S, show promise but also highlight significant hurdles. This vaccine, aimed primarily at the first stage of the parasite’s development after it enters the human bloodstream, exemplifies the challenges inherent in targeting a rapidly altering pathogen.
One significant benefit of focusing on vaccine development is the potential for reducing disease incidence, thereby alleviating the strain on health systems in affected regions. The ability to intervene early, ideally before infection exacerbates, means that health care resources could be allocated more efficiently. Moreover, a successful vaccine could foster herd immunity, particularly in densely populated areas, which could further curtail transmission rates.
However, developing a vaccine isn't just about the biology of the parasites. Considerations also involve public acceptance, distribution logistics, and potential geopolitical factors that could impede effective rollout. Understanding these can make or break a program's success.
"A vaccine is not just a biological product; it's a socio-political phenomenon that can change the landscape of health care in malaria-endemic regions."
Genomic Studies and Their Implications
Genomic research plays an invaluable role in the future perspectives of malaria control methods. By decoding the genetic blueprints of various Plasmodium strains, researchers are better equipped to understand how these parasites adapt and evolve. This knowledge can lead to the identification of new drug targets, improving existing treatments and perhaps even guiding vaccine development.
One of the exciting aspects of genomic studies is their precision. Modern techniques permit scientists to explore the genomes of different strains with a level of detail unheard of in previous eras. This effort can reveal why certain strains are resistant to drugs, how they evade the immune response, and where adaptations are occurring that facilitate transmission.
Additionally, genomic surveillance aids in tracking the emergence of drug resistance and monitoring the effectiveness of control measures. This adaptive approach not only helps in tailoring interventions to specific outbreaks but also allows for bulk understanding of malaria epidemiology across diverse locales.
- Enhanced drug design tailored to specific genetic traits.
- Identification of genetic markers associated with virulence and transmission bottlenecks.
- Facilitation of international cooperation through shared genomic data, promoting a unified response to outbreaks.
Closure
The exploration of the physical and biological life cycle of Plasmodium is not just an academic pursuit but a vital endeavor for public health and disease control. Understanding how this protozoan develops, particularly its stages within both mosquito and human hosts, is paramount in devising effective strategies to combat malaria.
Summary of Key Points
- Complex Life Stages: The life cycle of Plasmodium involves intricate transitions from sporozoites in the mosquito to various forms in human hosts, including merozoites and trophozoites. Each stage is designed to exploit vulnerabilities in the hosts' physiological systems.
- Transmission Dynamics: Highlighting the dependency of Plasmodium on its mosquito vector emphasizes the integral connection between environmental factors and infection rates. From climate to ecological changes, various elements enhance or hinder transmission.
- Host-Pathogen Interaction: The interaction between Plasmodium and human immune responses elucidates the capabilities of this parasite to adapt and thrive, often evading typical immune defenses. Key adaptive mechanisms shed light on treatment resistance, which is crucial for future therapies.
- Innovative Control Methods: The article also discusses present control methods while presenting cutting-edge research directions. Innovations in vaccine development and genomic studies may hold the key to eradicating malaria, underlining the optimistic outlook ahead.
Call for Continued Research
Continued research remains essential for several reasons:
- Understanding Adaptations: Investigating how Plasmodium evolves in response to new host defenses can improve our strategies to counteract its growth.
- Addressing Resistance: As drug resistance becomes a pressing issue, there's a pivotal need to focus on alternatives that could provide new solutions.
- Global Health Implications: With malarial transmission remaining a global concern, insights drawn from ongoing research could lead to more effective public health policies and educational outreach programs.
To sum up, our grasp of the Plasmodium life cycle, its complexities, and its implications must keep evolving through rigorous studies and innovative approaches. The fight against malaria is anchored not just in current knowledge but in the relentless pursuit of better understandings and solutions.