Understanding the Role of Polypeptides in Protein Synthesis
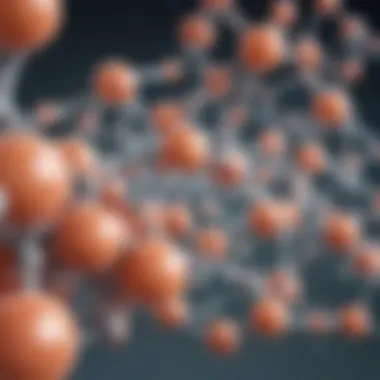
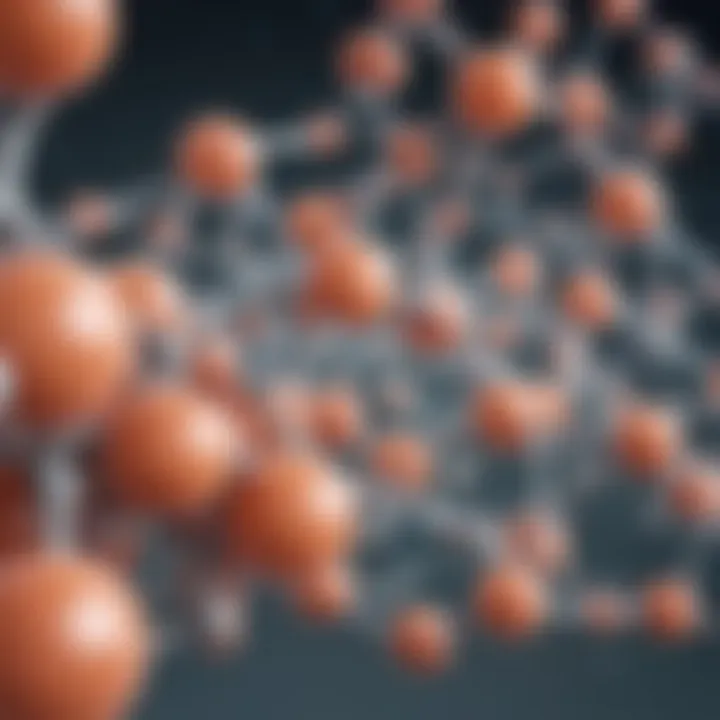
Intro
The study of polypeptides holds significant importance in understanding protein synthesis. As biopolymers, polypeptides are essential components in the production of proteins. They play a pivotal role within the cell, connecting the genetic code to functional proteins. The synthesis of proteins from polypeptides begins with the transcription of DNA to RNA, followed by the translation of that RNA into a polypeptide chain. This chain ultimately folds into a specific three-dimensional structure to create a functional protein.
Understanding the mechanics behind polypeptide formation can elucidate how errors in this process might lead to diseases. For example, mutations in DNA that disrupt the reference sequence can result in dysfunctional proteins, which could cause various health issues. Thus, analyzing polypeptides gives insights not only into basic biology but also into potential medical applications. The following sections will detail the processes involved in protein synthesis, focusing specifically on the actions involving polypeptides.
Research Background
Overview of the Scientific Problem Addressed
The intricate process of protein synthesis involves numerous molecular interactions that are critical for cellular function. Polypeptides are the direct products of this synthesis and serve as the building blocks for proteins. Understanding how polypeptides form and their subsequent functions is crucial for investigating biological systems. Researchers have long sought to clarify these processes, as any disruption can lead to severe pathological conditions.
Historical Context and Previous Studies
The understanding of polypeptides and their role in protein synthesis has evolved significantly over the years. In the early 20th century, scientists began unraveling the structure of proteins. The discovery of the double helix structure of DNA by Watson and Crick in 1953 opened new avenues for genetic research. Shortly after, the process of transcription was identified, revealing how RNA synthesized from DNA serves as a template for polypeptide formation.
Various experimental approaches, such as X-ray crystallography and mutations studies, have propelled the comprehension of polypeptide structure and function. Studies have shown that the linear sequence of amino acids in a polypeptide influences its final shape and activity within the cell. This emphasizes the vital connection between genetics and biochemical pathways, where polypeptides are at the center of protein synthesis.
Findings and Discussion
Key Results of the Research
Recent findings illustrate the essential mechanisms through which polypeptides contribute to protein synthesis. These include the role of ribosomes, which facilitate the translation of mRNA into polypeptides. Furthermore, the amino acid sequence dictates the way a polypeptide folds and interacts with other molecules, influencing its overall functionality.
Interpretation of the Findings
Understanding the way polypeptides form and function establishes a foundation for comprehending various biological processes. Post-translational modifications, which often occur after polypeptides have formed, can affect their activity and interactions significantly. These modifications include phosphorylation, glycosylation, and ubiquitination, each playing a distinct role in cellular signaling and regulation.
Polypeptides are more than just simple chains of amino acids; they embody the translation of genetic information into biological action. Recognizing their roles expands the horizon for biotechnological applications and advanced therapeutic strategies in molecular biology.
Polypeptides serve as a bridge between DNA and functional proteins, highlighting the central role they play in life sciences.
Foreword to Polypeptides
Understanding polypeptides is crucial to grasping the broader topic of protein synthesis. Polypeptides serve as the foundational structures from which proteins are built. This section will provide a clear outline of what polypeptides are and why they matter in the context of biological processes.
Polypeptides are strings of amino acids, linked together by peptide bonds, and they play vital roles in various cellular functions. Their sequences determine protein structure and function, influencing how proteins will behave in a biological environment. Here, we introduce essential concepts that will set the stage for a complete exploration of polypeptide functionality.
Definition and Basic Structure
Polypeptides are composed of amino acids, which are the basic units of proteins. Each amino acid has a general structure consisting of:
- A central carbon atom
- An amino group (-N)
- A carboxyl group (-COOH)
- A hydrogen atom
- A distinctive side chain (R group) that varies among different amino acids.
The unique sequence and composition of these amino acids create a polypeptide chain. Typically, polypeptides contain more than 50 amino acids. Once synthesized, they can fold into specific configurations, which are essential for their function as proteins.
In essence, the structure of a polypeptide dictates its properties and cellular functions. This foundational understanding is critical for further examining how polypeptides contribute to protein synthesis and various biological mechanisms.
Polypeptides vs. Proteins
There is often confusion between polypeptides and proteins. The distinction lies primarily in size and complexity.
- Polypeptides:
- Proteins:
- Chains of amino acids.
- Usually composed of fewer than 50 to 100 amino acids.
- Can serve as precursors to proteins, but may also function independently for specific tasks in the cell.
- Larger and more complex than polypeptides.
- Comprised of one or more polypeptide chains folded into a precise three-dimensional structure.
- Functionality and role in biological processes depend heavily on this structure and arrangement.
It is important to note that every protein starts as a polypeptide. The transition from polypeptide to fully functional protein involves folding, and often, additional modifications may occur after synthesis. Understanding these differences provides necessary clarity on how polypeptides fit into the larger narrative of protein synthesis and function.
The Biogenesis of Polypeptides
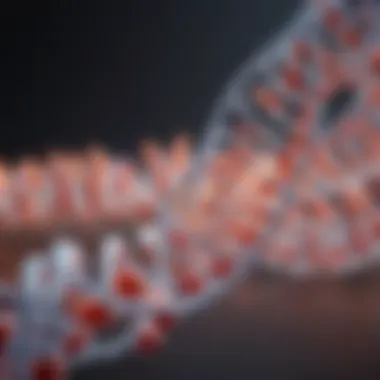
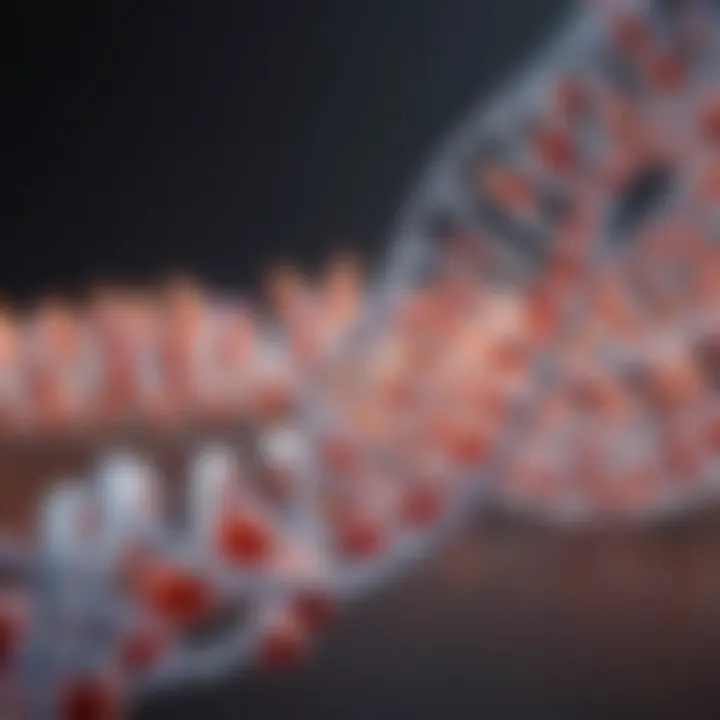
The biogenesis of polypeptides is a fundamental concept that underpins the processes of protein synthesis in all living organisms. Understanding how polypeptides are formed is essential for comprehending their functions in cellular biology. The formation of polypeptides involves a series of carefully orchestrated steps that begin with the assembly of amino acids. This process is crucial because polypeptides serve as the building blocks for proteins, which play diverse roles in biological systems. By exploring the details of this process, we lay the groundwork for understanding the significance of polypeptides in health, disease, and biotechnology.
Amino Acids as Building Blocks
Amino acids are often referred to as the basic units of polypeptides. There are 20 standard amino acids, each with a specific side chain that gives it unique properties. These amino acids link together to form polypeptides through a series of reactions. The sequence in which amino acids are arranged is determined by the genetic code found in DNA. Each sequence corresponds to a specific protein and its unique function.
Amino acids have an amine group, a carboxyl group, and a defining side chain. The diversity of the side chains allows for a wide variety of interactions, which ultimately influence the structure and function of the resulting polypeptides. This functional diversity is critical. Some common functions of proteins formed from amino acids include:
- Catalyzing biochemical reactions (enzymes)
- Providing structural support in cells and tissues
- Transporting molecules across cell membranes
- Regulating biological processes
Given their essential role, changes in the availability of amino acids can have profound effects on polypeptide synthesis, and thereby influence cellular functions. Understanding how amino acids act as building blocks enables researchers to explore new avenues in medicine and biotechnology.
Peptide Bond Formation
Peptide bond formation is the process that links amino acids together to create polypeptides. This occurs when the carboxyl group of one amino acid reacts with the amine group of another, releasing a molecule of water. This reaction is known as a condensation reaction or dehydration synthesis. The resulting bond is quite stable and essential for the integrity of polypeptides.
Peptide bonds are characterized by partial double-bond characteristics, which limit rotation around the bond and contribute to the stability of the polypeptide structure. Once formed, peptide bonds result in a linear chain of amino acids, which can then fold into specific three-dimensional shapes essential for proper functioning.
The process of peptide bond formation can be summarized as follows:
- Activation: The amino acid is activated, typically by tRNA in the ribosome.
- Binding: The activated amino acid binds to the ribosome.
- Condensation: A peptide bond forms as a water molecule is removed.
This formation is vital, as the sequence and arrangement of amino acids dictate the final protein's structure and function. Failure in peptide bond formation can lead to dysfunctional proteins, which in turn can cause various diseases. Hence, understanding peptide bond formation helps in revealing the mechanisms through which various polypeptide-related abnormalities may arise.
Protein Synthesis Overview
Protein synthesis plays a pivotal role in cellular function and regulation. This process is crucial for producing polypeptides, which eventually shape into functional proteins, serving various roles in metabolism, signaling, and structure. Understanding protein synthesis provides insights into how genetic information translates into real-world biological activity.
Mechanisms of Gene Expression
Gene expression is the fundamental process through which genetic instructions are converted into products like proteins. It involves a series of steps, including transcription and translation. The mechanisms underlying gene expression are vital, as they highlight how cells respond to internal and external signals. In essence, gene expression determines which proteins are synthesized, directly influencing cellular behavior.
Transcription Process
The transcription process is the first step in gene expression where genetic information from DNA is transcribed into messenger RNA (mRNA). This step is crucial since it sets the stage for subsequent protein synthesis. A well-coordinated transcription process ensures that only the necessary proteins are expressed at any given time.
Role of RNA Polymerase
RNA polymerase is the enzyme responsible for synthesizing RNA from a DNA template. It binds to a specific site on the DNA, initiating transcription. One key characteristic of RNA polymerase is its ability to read the DNA strand and incorporate complementary ribonucleotides to form a single-stranded mRNA transcript. This enzyme is favored in this context due to its efficiency and specificity in recognizing promoter regions.
One unique feature of RNA polymerase is its proofreading capability, which helps maintain the accuracy of mRNA production. However, despite its advantages, if RNA polymerase encounters damaged DNA, this may lead to faulty mRNA, affecting protein synthesis down the line.
Formation of mRNA
Formation of mRNA involves the precise copying of the DNA sequence, resulting in a strand that is complementary to the original DNA. The key characteristic of mRNA formation is the processing that occurs after transcription. This includes capping, polyadenylation, and splicing. These modifications are essential as they protect the mRNA and facilitate its recognition by ribosomes during translation.
One unique advantage of forming mature mRNA lies in its stability and transport efficiency within the cell. However, improper mRNA processing can lead to issues in translation, which can have downstream effects on protein production.
Translation Process
Translation is the subsequent step following transcription, where the mRNA strand is read by ribosomes to synthesize polypeptides. This process is essential for effective protein synthesis and is divided into three stages: initiation, elongation, and termination.
Initiation
Initiation marks the beginning of translation, where the ribosome assembles around the start codon of the mRNA. The recognition of the start codon is a significant aspect since it establishes the reading frame for the ribosome. This phase is crucial as it determines the accuracy of subsequent polypeptide synthesis.
One distinguishing quality of the initiation phase is the role of initiation factors, which assist in ribosome assembly. An advantage of focused initiation mechanisms is the increased efficiency in producing proteins needed at specific times, although complex initiation processes can introduce errors if not properly regulated.
Elongation
During elongation, amino acids are added sequentially to the growing polypeptide chain based on the mRNA sequence. This stage requires elongation factors that facilitate the binding of new aminoacyl-tRNAs to the ribosome. The key feature of elongation lies in its stepwise addition of amino acids, driven by the codon-anticodon interactions.
The unique aspect of elongation is the rapid incorporation of amino acids when conditions are optimal, allowing for efficient protein synthesis. Nonetheless, elongation can be delayed if proper tRNAs are not available, negatively impacting overall protein output.
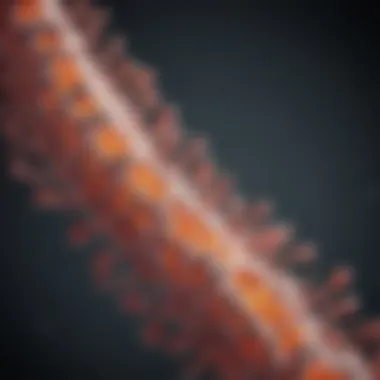
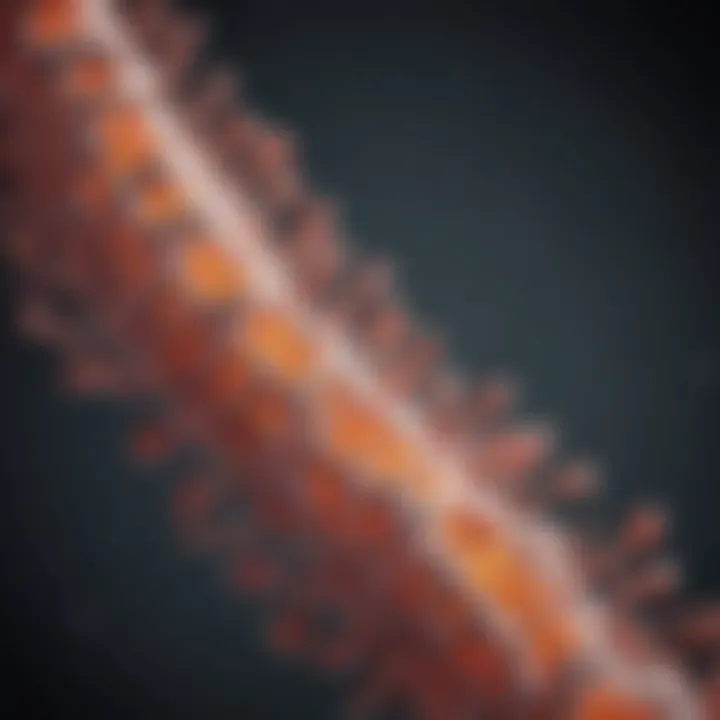
Termination
Termination occurs once the ribosome reaches a stop codon on the mRNA. This phase is characterized by the release factors that promote the disassembly of the ribosomal complex and release the newly synthesized polypeptide. The significance of termination cannot be understated as it ensures that proteins are completed and released properly.
One key feature of termination is its reliance on stop codons, ensuring that protein synthesis concludes at the correct point. This precision allows for the production of functional proteins that can perform their respective roles. Misregulation during termination can lead to truncated proteins, which might be non-functional or detrimental to cell function.
Functions of Polypeptides in Cells
Polypeptides serve critical functions within cells. Their roles extend beyond mere construction units for proteins. This section will explore the importance of polypeptides in cellular activities, focusing mainly on two key roles: their enzymatic functions and their contribution as structural components.
Enzymatic Roles
Polypeptides act primarily as enzymes within biological systems. Enzymes are specialized proteins that facilitate biochemical reactions. They lower the activation energy, thereby accelerating the reaction rate. Without polypeptides functioning as enzymes, many metabolic processes would occur too slowly to sustain life.
Some notable aspects of enzymatic roles include:
- Catalysis: Enzymes play a crucial role by converting substrates into products efficiently. The active site of an enzyme is the region where this conversion occurs. The structure of polypeptides allows for specific folding patterns which create unique active sites tailored to particular substrates.
- Regulation: Polypeptides can also regulate enzymatic activity. Modifications after translation, such as phosphorylation or methylation, can enhance or inhibit their actions. This flexibility is essential for adapting cellular responses to various signals.
In summary, the enzymatic roles of polypeptides underscore their importance in maintaining cellular function and promoting metabolic pathways. Their specific structures dictate their functionality, highlighting the intricate relationship between polypeptide formation and enzymatic efficiency.
Structural Components
Beyond their roles as enzymes, polypeptides are integral to cellular structure. They contribute to the formation of various cellular components, including membranes, cytoskeletons, and other organelles. The structural roles of polypeptides can be understood through these points:
- Protein Frameworks: Polypeptides assemble into complex protein structures that provide support and shape to cells. Collagen, for instance, is a polypeptide-rich protein that helps maintain the integrity of tissues, such as skin and cartilage.
- Membrane Formation: Certain polypeptides become part of the membranes that encase cells and organelles. Integral membrane proteins facilitate communication and transport across the membrane, directly impacting cellular interactions with the environment.
- Cell Movement: The cytoskeleton, composed of various polypeptides like actin and tubulin, is essential for cellular movement and shape changes. These structural components allow for processes such as cell division and motility.
"Polypeptides, through their diverse roles, highlight the complexity and elegance of life at the molecular level."
By examining these functions, we gain insight into how polypeptides influence biological processes, reinforcing their position as foundational elements in life sciences.
Post-translational Modifications
Post-translational modifications (PTMs) play a significant role in the functionality of polypeptides and the proteins they form. Once polypeptides are synthesized through translation, they undergo a series of chemical changes that can drastically influence their activity, stability, and interactions with other molecules. These modifications are crucial for determining the final structural configuration and functional capacity of proteins. Understanding PTMs provides insight into how proteins can perform a wide range of biological functions within the cell.
Common Modifications
Phosphorylation
Phosphorylation is one of the most widely studied post-translational modifications. This process involves the addition of a phosphate group, typically to serine, threonine, or tyrosine residues on a polypeptide. The key characteristic of phosphorylation is its reversible nature, allowing proteins to quickly change their activity in response to various cellular signals. This makes phosphorylation a beneficial choice in regulating numerous processes, such as signal transduction, cell cycle progression, and metabolic pathways.
One unique feature of phosphorylation is its role in creating binding sites for other proteins. The introduction of a phosphate group can alter the three-dimensional structure of the protein, leading to new interactions that are vital in cellular signaling pathways. However, while beneficial, improper phosphorylation can lead to various diseases, including cancers and neurodegenerative disorders, underscoring the importance of regulatory mechanisms.
Glycosylation
Glycosylation involves the addition of carbohydrate groups to a polypeptide chain. This modification is critical for protein stability, localization, and recognition. One of the defining characteristics of glycosylation is its complexity. It can occur in various forms, such as N-linked or O-linked glycosylation, depending on the attachment point of the carbohydrate.
This modification is particularly beneficial as it impacts protein folding and enhances solubility. Most glycoproteins play vital roles in cell-cell interaction and signaling. However, the intricate nature of glycosylation can pose a challenge, as aberrant glycosylation patterns are often implicated in diseases like congenital disorders and cancer. Thus, understanding glycosylation processes is key in therapeutic developments and disease management.
Impact on Protein Function
The impact of post-translational modifications on protein function cannot be overstated. PTMs are imperative in dictating the activity, lifespan, and interacting partners of proteins. They enable proteins to respond to cellular environments dynamically, catering to the complex needs of cellular networks.
For instance, phosphorylation can activate or deactivate enzymatic activity based on cellular stimuli, whereas glycosylation often dictates the proteinβs destination within the cell. Collectively, these modifications not only fine-tune protein functionality but also integrate into larger signaling networks that govern cellular behavior.
As we continue to unravel these processes, the potential for PTMs in medical applications becomes increasingly clear. Better understanding these modifications may lead to novel therapeutic strategies for diseases where protein function is impaired.
Post-translational modifications are essential for the versatility and adaptability of proteins in biological systems.
Polypeptides and Genetic Regulation
Polypeptides play a crucial role in the regulation of gene expression. This regulation is essential for maintaining cellular homeostasis and ensuring that proteins are produced as needed. Polypeptides can act as regulatory proteins that influence various cellular processes, including those involved in transcription and translation. Understanding how polypeptides regulate gene expression helps in deciphering the complex networking between genes and proteins.
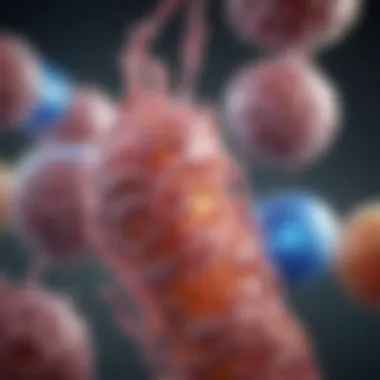
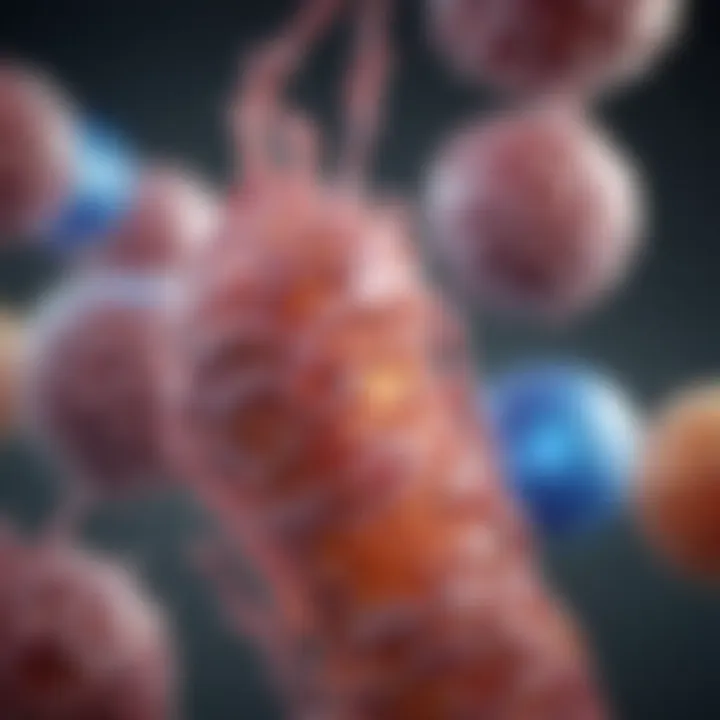
Role of Regulatory Proteins
Regulatory proteins, often specific polypeptides, interact with DNA and other proteins to control the transcription of genes. These interactions can either enhance or inhibit the transcription process. For instance, transcription factors are a type of regulatory protein that binds to specific DNA sequences. By doing this, they can facilitate or obstruct the recruitment of RNA polymerase, the enzyme responsible for synthesizing RNA from a DNA template.
Notably, regulatory proteins can also influence chromatin structure, thereby affecting gene accessibility. This is crucial because tightly packed chromatin can prevent transcription, while loosely packed chromatin allows easier access for the transcription machinery.
In addition, some regulatory proteins serve as co-factors that modify other proteins. They can add chemical groups to certain polypeptides or influence their conformation, which can change their activity and, consequently, gene expression. This highlights the dynamic nature of polypeptide interactions and their importance in regulating genetic pathways.
Feedback Mechanisms
Feedback mechanisms provide a vital control system in cellular processes. In genetic regulation, polypeptides can play a role in feedback loops, allowing cells to respond to internal and external signals effectively. When a protein product reaches a certain threshold, regulatory polypeptides may inhibit further transcription of the corresponding gene. This prevents overproduction and maintains balance in cellular environments.
For instance, negative feedback occurs when the end product of a pathway inhibits an earlier step, often through the action of a regulatory polypeptide. This mechanism is vital for metabolic pathways, where the accumulation of substances can signal that enough product is already present, thus stopping further synthesis.
The interplay between genetic regulation and polypeptide activity is essential for cellular adaptation and function.
On the other hand, positive feedback can amplify the effects of a particular gene by enhancing its expression under certain conditions. Regulatory polypeptides involved in this mechanism can help rapidly escalate the cell's response to environmental changes or stresses by promoting the synthesis of more proteins in a given pathway.
Disorders Related to Polypeptide Synthesis
Polypeptides play a crucial role in the synthesis of proteins, which are essential for nearly all biological functions. Disorders related to polypeptide synthesis can have significant consequences on health and cellular processes. Understanding these disorders is vital for developing strategies to address them. It involves a complex interplay between genetic, biochemical, and environmental factors.
Genetic Mutations
Genetic mutations can lead to disorders that impact polypeptide synthesis. Mutations may alter the amino acid sequence of polypeptides, which in turn affects the structure and function of the resulting protein. Some mutations result in non-functional proteins, while others create proteins with altered functions, contributing to disease. Such issues exemplify the delicate nature of genetic information and its direct influence on health outcomes.
Impact on Health
Example Conditions
One prominent example of a condition related to polypeptide synthesis disorders is Cystic Fibrosis. This genetic disorder arises from mutations in the CFTR gene, which encodes for a protein involved in the regulation of salt and water transport across cell membranes. A key characteristic of Cystic Fibrosis is the production of thick, sticky mucus, leading to severe respiratory and digestive problems.
The unique feature of Cystic Fibrosis is not just the genetic mutation but also the manifestation of symptoms that arise from this polypeptide dysfunction. It highlights the impact that faulty polypeptide synthesis can have on overall health, emphasizing the need for targeted therapies.
Therapeutic Approaches
There are various therapeutic approaches aimed at addressing disorders related to polypeptide synthesis. One such method is the use of gene therapy, which seeks to correct the underlying genetic mutation responsible for conditions like Cystic Fibrosis. This approach can potentially restore normal protein function, thus alleviating symptoms.
Key characteristic of these therapeutic approaches is their focus on targeting the root cause of the disorder, rather than just managing symptoms. Moreover, they present promising avenues for treatment, but they also come with challenges. Genetic correction needs to be precise to avoid adverse effects, which makes research and clinical trials essential in this area.
The Future of Polypeptide Research
The future of polypeptide research remains a crucial element in understanding various biological processes. As biotechnology advances, the study of polypeptides opens doors to new insights into protein synthesis and function. Understanding polypeptides can unlock new ways to tackle diseases and improve therapeutic strategies.
Continued exploration in this domain also fosters developments in synthetic biology and bioinformatics. This interdisciplinary approach allows researchers to analyze polypeptide structures and their interactions more effectively. The importance of this research is evident in its potential to create tailored therapies that target specific proteins related to various conditions, offering more personalized treatment options.
Advancements in Biochemistry
Recent advancements in biochemistry offer unprecedented opportunities for polypeptide research. Techniques such as CRISPR and high-throughput sequencing have transformed how scientists study the intricate details of polypeptide formation. These technologies enable more precise manipulation of genetic material, allowing for clearer insights into the processes involved in protein synthesis.
With the advancement of computational methods, models predicting polypeptide behavior in different environments have become increasingly accurate. This precision will enable researchers to simulate polypeptide interactions and their physiological roles more effectively, contributing to a deeper understanding of cellular functions.
Applications in Medicine
Drug Development
In drug development, polypeptides play a vital role due to their ability to interact with various biological targets. This characteristic is critical for creating new therapeutics aimed at specific diseases. Polypeptide-based drugs are often designed to mimic naturally occurring proteins, improving their efficacy and reducing side effects.
One significant advantage of polypeptide drugs is their specificity. They can be engineered for high affinity and selectivity towards particular biomolecules, enhancing therapeutic effects. However, challenges do arise, such as stability and delivery mechanisms, which require ongoing research and innovation.
Gene Therapy
Gene therapy represents another promising application of polypeptides in medicine. By utilizing polypeptides that can deliver genetic material to targeted cells, researchers can effectively address genetic disorders at their source. This targeted approach enhances the potential for curing diseases that were once seen as untreatable.
The unique feature of gene therapy is its ability to provide long-lasting solutions by correcting or replacing defective genes. While the benefits are substantial, challenges remain concerning delivery efficiency and safety, necessitating rigorous testing and development.
The exploration of polypeptides continues to pave the way for innovative medical treatments that can redefine patient care.
Overall, the future of polypeptide research is filled with promise and potential applications that could reshape the landscape of medicine and biochemistry.