Mastering Primer Design for PCR Efficiency
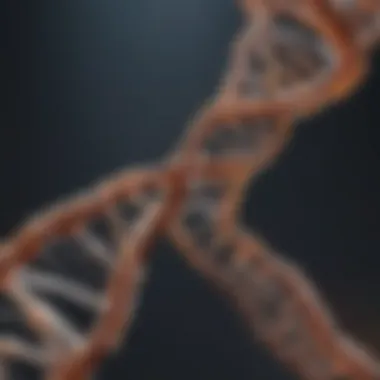
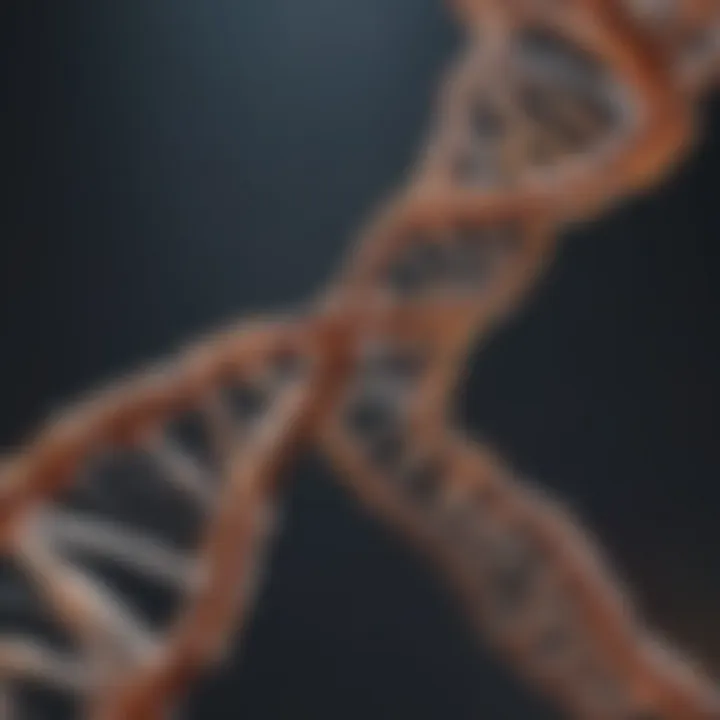
Intro
In the realm of molecular biology, the polymerase chain reaction (PCR) stands as a cornerstone technique, revolutionizing how researchers amplify specific DNA sequences. A well-designed primer is at the heart of successful PCR, as it determines not only the specificity of the amplification but also the efficiency of the entire process. This article serves as a practical guide for designing effective forward and reverse primers, aiming to equip students, researchers, educators, and professionals with the essential tools and insights needed for successful PCR applications.
Developing primers might seem straightforward, but it involves a nuanced understanding of biophysical principles, historical context, and iterative optimization. From the basic structure of primers to innovative strategies used in contemporary research, this guide offers a range of perspectives that will deepen the reader's understanding of the topic.
By exploring various design parameters and troubleshooting strategies, we aim to demystify the complexities involved and contribute to enhanced outcomes in biological research, ultimately pushing the boundaries of what is possible in gene amplification and analysis.
Research Background
Overview of the Scientific Problem Addressed
The need for precise DNA amplification arises in a multitude of scientific contexts—from diagnosing diseases to cloning genes. The success of PCR hinges on the quality and specificity of primers. When primers are not well-designed, the result can be non-specific amplifications or failed reactions, leading to wasted resources and frustrating setbacks. Thus, understanding how to create effective forward and reverse primers is crucial for successful experimental outcomes.
Historical Context and Previous Studies
The journey of PCR primer design traces back to the monumental work of Kary Mullis in the 1980s. His invention of the PCR technique paved the way for amplifying minute quantities of DNA, but it was not until the early 1990s that primer design strategies began to be formalized through systematic studies. Previous research has identified key factors that influence primer annealing, specificity, and overall performance.
Common guidelines emerged, such as avoiding secondary structure formation, ensuring a melting temperature (Tm) within a close range for paired primers, and considering potential dimerization. As the field has progressed, incorporating computational tools for primer design has become increasingly popular, allowing researchers to predict the behavior of primers in silico before actual laboratory testing.
Findings and Discussion
Key Results of the Research
Through the exploration of various studies, several critical insights have been obtained regarding primer design:
- Melting Temperature (Tm): A prime factor in primer performance; mismatched primers can significantly lower the amplification efficiency.
- GC Content: Primers with balanced GC content often yield better specificity and stability, as GC pairs form three hydrogen bonds compared to two from AT pairs.
- Length: Typical primers range from 18 to 25 nucleotides, providing a balance between specificity and synthesis efficiency.
Interpretation of the Findings
These foundational elements highlight that a meticulous approach is essential for primer design. While general guidelines exist, true expertise involves tweaking these variables based on the specific context of the PCR experiment being conducted. As technology evolves, continuous innovations in this field are shaping how we understand and apply primer design, signaling that while the principles may remain, the methods employed are always advancing.
It becomes critical to stay informed and adapt to these emerging techniques, which can enhance PCR efficiency and specificity—vital aspects for any researcher striving for precise results in DNA amplification.
Primer design is not one size fits all; it's an art that requires a balance of science and creativity, ensuring effective engagement with the target sequence.
Intro to Primer Design
Primer design is a cornerstone of molecular biology, especially when we talk about polymerase chain reaction (PCR). This technique allows for the amplification of specific DNA sequences. But before diving into the nitty-gritty of the process, it’s crucial to grasp why primer design holds such importance in this field. Effective primer design can mean the difference between a successful experiment and a frustrating dead-end.
Primers, which are short strands of nucleic acids, serve as the starting points for DNA synthesis. Think of them as the guideposts that direct the DNA polymerase to the right spot in the DNA strand you wish to duplicate. Without well-crafted primers, amplification may go off track, resulting in poor yields or even non-specific products.
To design optimal primers, one must consider several specific elements and benefits:
- Specificity: Primers should match the target sequence tightly. Any mismatch can lead to non-specific binding and amplification of unintended sequences.
- Efficiency: Well-designed primers help to ensure that the amplification process runs smoothly and quickly.
- Stability: Primers need to possess the right melting temperatures to balance the hybridization and denaturation steps, minimizing the chances of inadvertent mismatching during PCR cycles.
- Compatibility: If multiple primer pairs are used in a single run, their qualities must not only be optimized for their individual targets but also for their interactions with each other.
In summary, primer design is not just a technical task; it is an art that blends insights from chemistry and biology, requiring attention to detail and a deep understanding of the fundamental concepts of nucleic acids. By mastering this skill, researchers and educators equip themselves for success in various scientific applications, from genetic research to diagnostic testing.
Understanding Nucleic Acids
Nucleic acids are the building blocks of genetic information within all living organisms. The primary types, deoxyribonucleic acid (DNA) and ribonucleic acid (RNA), serve distinct functions but share common characteristics. Understanding these macromolecules is essential to effective primer design.
DNA, the molecule responsible for heredity, has a double helix structure made up of nucleotide units. Each nucleotide consists of a sugar, a phosphate group, and one of four bases: adenine (A), thymine (T), cytosine (C), or guanine (G). The sequence of these bases encodes genetic instructions. On the other hand, RNA is typically single-stranded and contains uracil (U) instead of thymine, which allows it to play a key role in protein synthesis.
- Structure: The unique structures of DNA and RNA are crucial; they underpin how primers anneal during amplification.
- Physical properties: The base-pairing rules – A with T (and U in RNA) and C with G – dictate the binding affinity of primers, which directly impacts the success rate of PCR.
By demystifying the role of nucleic acids, one starts to appreciate the subtle nuances of primer design, which ultimately feeds into achieving high specificity in PCR reactions.
The Role of Primers in PCR
Primers play a pivotal role in PCR, acting as essential components for amplifying the targeted DNA sequences. Without primers, the PCR process would essentially be rudderless: unable to initiate the replication of the targeted region.
When a PCR cycle begins, the DNA double helix is heated to denature it, separating the strands. At this point, primers bind to the template DNA, providing a starting point for DNA polymerase. The DNA polymerase extends the strands from the primers, creating new copies of the target sequence. This process, which involves repeated temperature cycling, exponentially increases the number of copies of the DNA in question.
A couple of key points about the role of primers:
- Specificity in amplification: By targeting unique sequences, primers ensure that only the desired DNA segments are duplicated, which is critical for applications ranging from forensic analysis to cloning.
- Efficiency factors: The right primers can significantly enhance the efficiency of PCR. Sub-optimal primers may lead to lower yields or non-specific products, prolonging experiments and wasting resources.
Types of Primers
Understanding the different types of primers is the backbone of effective PCR (Polymerase Chain Reaction) utilization. Each type serves distinct roles in the amplification process, and knowing when and how to use them can significantly enhance the accuracy and efficiency of your results. This section sheds light on two primary categories: forward and reverse primers, discussing their unique characteristics, applications, and considerations when designing them.
Forward Primers Explained
Forward primers, as the name suggests, are crucial for initiating the DNA synthesis in a specific direction. They bind to the complementary strand of DNA, providing a starting point for DNA polymerase during replication. This specificity is key; the selected region must closely match your target sequence.
When designing forward primers, researchers often consider several factors:
- Length and Composition: A typical forward primer ranges from 18 to 25 nucleotides. The goal here is to ensure enough specificity without being too long or short, which could either hinder binding or lead to non-specific amplifications.
- GC Content: An ideal GC content lies between 40-60%. High GC stretches can create stable binding regions, but excessive GC can also complicate melting temperatures.
- Avoiding Secondary Structures: Using tools to visualize potential hairpin formations or homodimers is essential. These structures can inhibit proper annealing and thus affect the yield.
Properly designed forward primers can significantly reduce non-specific binding and enhance the clarity of your results.
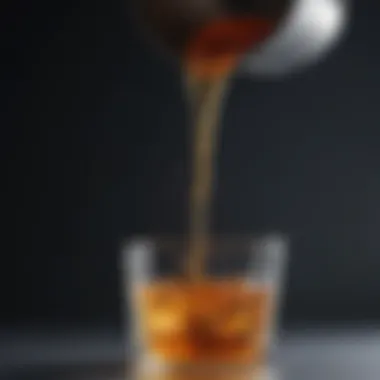
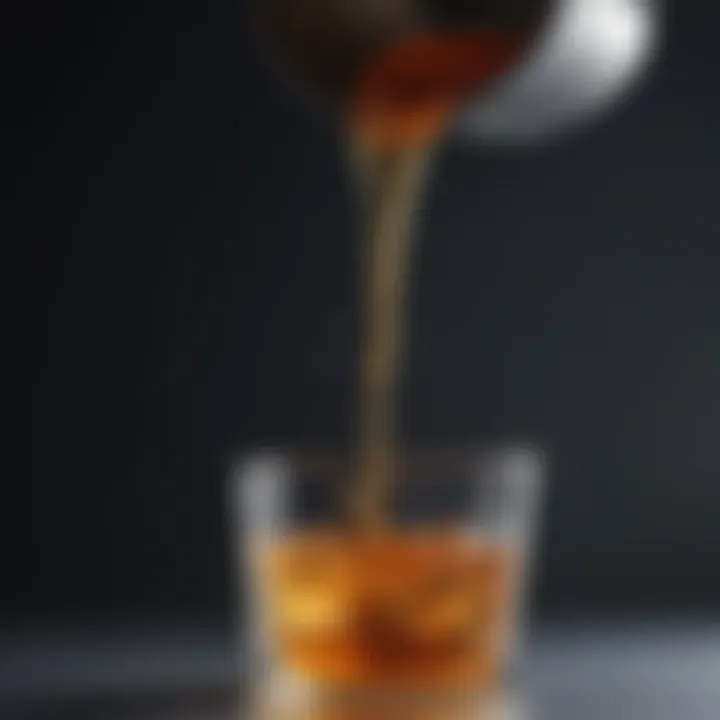
Reverse Primers Explained
On the other hand, reverse primers complement the forward primers by binding to the opposite strand of DNA. They also determine the ending point of the replication process. In a way, reverse primers balance the sequencing act, ensuring that the DNA strands are synthesized accurately and fully.
While designing reverse primers, similar considerations apply:
- Complementarity with Target Sequence: Ensure that the reverse primer binds accurately to the complementary target. Even slight mismatches can lead to ineffective PCR.
- Orientation Matters: Reverse primers should be designed to be complementary and antiparallel. This ensures they align correctly during synthesis.
- Stability: It is crucial to maintain stability between the forward and reverse primers, considering their combined melting temperatures to avoid amplification issues.
Utilizing a well-thought-out approach with both forward and reverse primers increases the chance of successful PCR reactions, which can be pivotal in research settings.
"In PCR, the choice of primers can make or break your experiment; they are the silent conductors of your genetic masterpiece."
In summary, distinguishing between forward and reverse primers provides a clearer lens through which to view their roles in PCR. Understanding their designs, functions, and interdependencies is step one towards successful amplification and experimentation.
Essential Characteristics of Effective Primers
When it comes to the art of PCR, there’s no denying that the effectiveness of a primer is central to the success of the entire endeavor. Effective primers bridge the gap between simple DNA sequences and the intricate processes of amplification. They don’t just ride along; they play a pivotal role. Understanding the essential characteristics of these primers means diving into the nuances of their design and functionality. In this section, we'll break down what makes primers effective and why those characteristics matter.
Length and Sequence
The length and sequence of a primer are like the blueprint of a building; they set the foundation for everything that follows. A typical forward or reverse primer ranges from 18 to 25 nucleotides. If it's too short, it risks binding nonspecifically, which leads to a muddle of unwanted products. Conversely, a primer that's excessively long may bind too tightly, limiting the desirability of amplification efficiency.
Key considerations to ponder:
- Specificity: You want a sequence that aligns perfectly with your target DNA. If a single base pair doesn't match, your primer could end up misfiring.
- GC Content: Maintaining a balanced GC content—ideally around 40-60%—helps ensure stable binding. Too much GC increases stability but may hinder melting, while too little can lead to fragility.
- Avoidance of Repeats: Sequences that are rich in repeats might bind to multiple sites, complicating the results.
Remember, the right blend of these factors can make all the difference between success and a fizzle.
Melting Temperature Considerations
The melting temperature (Tm) is another cornerstone concept in primer design. Think of Tm as the temperature where half of your DNA strands are in double-helix form and half are in the "single-stranded" state. The beauty of Tm lies in its ability to predict how well a primer will function under different temperatures—something critical during the denaturation phase of PCR.
How to gauge Tm effectively:
- Use the formula: Tm = 2(A + T) + 4(G + C), which provides a quick estimate.
- Ideally, primers should have similar Tm values—within 2-5°C of each other—to ensure that both forward and reverse primers act in unison. This harmony leads to reliable amplification.
- Avoiding low Tm values is key; primers with Tm below 50°C may struggle to bind tightly to the target DNA during PCR’s cycles.
In essence, thinking about Tm proactively helps avert a plethora of common problems.
Specificity and Binding Affinity
The terms specificity and binding affinity might seem interchangeable, but they serve distinct purposes. Specificity can be understood as a primer’s ability to bind only to the intended target sequence and not adjacent regions or random sequences. Meanwhile, binding affinity concerns the strength of that relationship once a connection is made.
Things to keep in mind:
- In silico analysis: Utilizing software tools for predicting binding sites is beneficial. These can help identify potential non-specific binding events before you hit the lab.
- Annealing temperature: Setting your PCR’s annealing temperature wisely will optimize the likelihood of specific binding while discouraging nonspecific interactions.
- Primer-dimer formation: Watch out for potential dimerization, as primers can also bind to each other instead of their DNA target. Maintaining distinct sequences helps mitigate this risk.
Ultimately, effective primer design isn't just a matter of following guidelines. It's about mastering the subtleties that lead to precision and reliability in experiments.
Emphasizing these specific characteristics isn't just an academic exercise—it's foundational for any successful PCR application. Understanding the delicate balance of length, Tm, specificity, and binding affinity will serve any researcher well, whether they're embarking on genetic testing, diagnostics, or any myriad of applications that PCR enables.
Designing Forward Primers
When it comes to the world of molecular biology, the importance of designing forward primers cannot be overstated. Forward primers serve as the starting point for DNA amplification during polymerase chain reactions (PCR), making their design critical for obtaining accurate and efficient results. A well-crafted forward primer not only enhances the specificity of the amplification process but also influences the overall yield. By understanding both the sequence and functional aspects of these primers, researchers can increase the likelihood of successful outcomes in their experiments.
Identifying Target Sequences
The journey of creating an effective forward primer often begins with the careful identification of the target sequence. This means pinpointing the specific region of DNA that one aims to amplify. In practice, this could mean diving deep into genome databases or previous research to find sequences that are unique to the particular target; think of this as the launch pad for your PCR experiment. Having a clear target helps to prevent issues related to unwanted amplification of non-target sequences, which is critical for maintaining specificity.
Some practical considerations in this stage include:
- Length of the Target Sequence: Ideally, the target sequence should be of sufficient length to provide a reliable template for the primer.
- Uniqueness: It’s crucial to ensure that the target sequence does not have significant homology with other regions within the genome.
- Contextual Importance: Understanding the biological relevance of the target sequence can help in determining its priority in the experimental design.
Utilizing Primer Design Software
Once the target sequence is identified, the next step is to leverage primer design software that can streamline the design process. These tools harness algorithms and databases to assist researchers in creating primers that meet various specifications. Most establishments offering these tools provide users with guidelines around the optimal characteristics of primers, ensuring designs are fit for purpose.
Utilizing such software has several benefits:
- Efficiency: It significantly reduces the time spent on manual calculations and design, enabling quicker progression in experiments.
- Accuracy: Software like Primer3 or NCBI Primer-BLAST minimizes human error by applying precision in generating sequences that meet specified criteria.
- Customization: Many platforms allow users to input various parameters, such as GC content and melting temperature, providing tailored outputs.
A user-friendly interface can make navigating through settings and options a breeze, making it a worthwhile investment for serious researchers.
Evaluating Primer Candidates
Choosing the right forward primer is not just a one-and-done affair; it involves a meticulous evaluation process. After generating several potential candidates, researchers need to assess them against established criteria to determine their viability before proceeding to benchwork.
Criteria for evaluation often include:
- Binding Affinity: Primers should exhibit a high binding affinity for the target site. Tools like melting temperature calculators are often used to gauge this factor.
- Secondary Structures: Potential for forming hairpin loops or dimers can significantly impede PCR efficiency. Predictive models can help identify these hindrances.
- Specificity: Unlike a shot in the dark, the selected primer should ideally amplify only your target sequence, minimizing nonspecific products.
In summary, evaluating primer candidates is about balancing stringency with practicality, ensuring that the selected primer aligns seamlessly with both the experimental goals and the specific characteristics of the target DNA.
Designing Reverse Primers
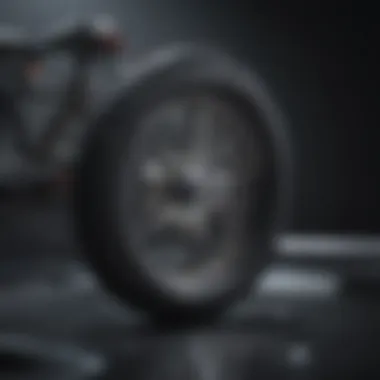
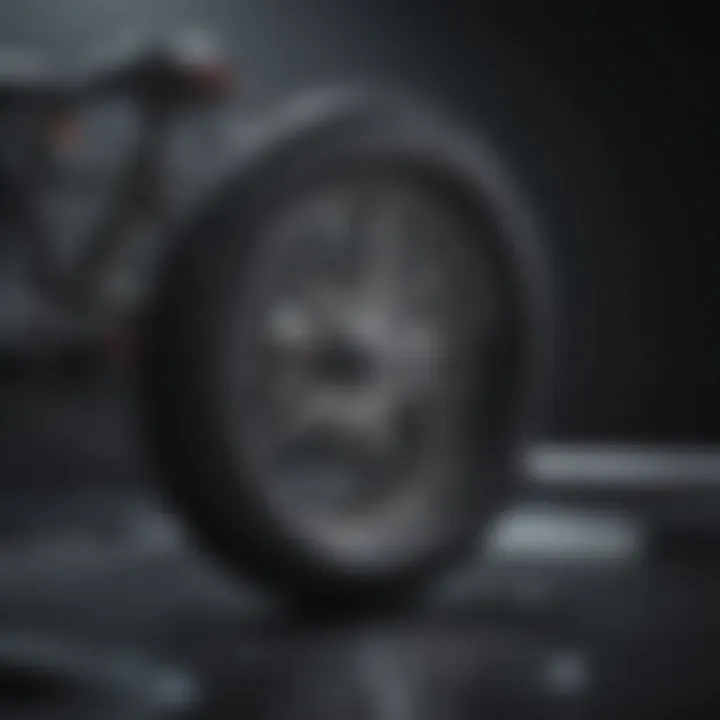
Designing reverse primers is a pivotal aspect of primer design in polymerase chain reaction (PCR) protocols. Often overshadowed by their forward counterparts, reverse primers are equally crucial for ensuring the specificity and efficiency of DNA amplification. One must grasp that while forward primers bind to the start of the target sequence, reverse primers lay down the groundwork for copying the complementary strand. This dual action is fundamental for achieving a balanced and effective amplification that drives both strands of the DNA template.
When embarking on the design of reverse primers, several key considerations must come into play. Firstly, the reverse primer must be complementary to the target region, mirroring the sequence from the opposite strand. This means that much like two peas in a pod, they not only need to match in sequence but also in their ability to effectively bind during the annealing phase of the PCR cycle. By having a clear understanding of the target sequence, one can create a reverse primer that works harmoniously with the forward primer, leading to robust PCR results.
Complementary to Target Sequences
A reverse primer should be tailored to complement the target sequence seamlessly. This requirement is akin to finding a missing puzzle piece; if the reverse primer does not match the complementary DNA strand, the entire amplification process risks being a flop. To achieve accurate binding, one needs to dissect the target sequence meticulously, identifying the appropriate region that lies at the 3' end of the strand being amplified.
When designing this primer, it is essential to consider the following:
- Sequence Alignment: The reverse primer should directly align with the specific target sequence. Software tools for bioinformatics can be a real godsend in checking alignment and ensuring that the target sequence is juxtaposed correctly with the designed primer.
- Avoiding Secondary Structures: A proper reverse primer should minimize the potential for secondary structures, such as hairpins or dimers, that might hinder efficiency. Shorter sequences are often beneficial in this aspect since they can reduce the chances of undesired interactions.
- Length and GC Content: Typically, a reverse primer should be around 18 to 25 nucleotides in length, with a balanced GC content ranging from 40% to 60%. This balance helps in maintaining stability during annealing.
The focus on complementary sequences ensures that the reverse primer operates effectively during the PCR, resulting in efficient amplification and specific binding.
Ensuring Proper Orientation
Beyond mere complementarity, ensuring the proper orientation of the reverse primer is fundamental for the integrity of the entire PCR process. This technicality, while sometimes overlooked, bears significant weight in influencing amplification outcomes. Essentially, the reverse primer must extend from the 3' end, pointing towards the target sequence.
To visualize this, consider the analogy of a train set: the forward primer lays down the track from the starting point, while the reverse primer must connect seamlessly from the end point back toward the beginning of the journey. If either primer is oriented incorrectly, it complicates the entire process, resulting in non-specific products or even complete failures in amplification.
Investigating the following criteria can assist in achieving proper orientation:
- Directionality: Confirm that the reverse primer is aligned in the right direction, towards the forward primer. Mismatches in this orientation can lead to inefficient PCR amplification.
- Using Bioinformatics Tools: Many bioinformatics platforms provide helpful design functions that can alert you to any misalignments or issues with primer orientation.
- Trial and Error: Sometimes, it may take a few iterations to find the optimal orientation and conditions for your reverse primer to work in tandem with the forward one.
In summary, designing reverse primers entails a careful balancing act. Both complementary alignment and proper orientation are crucial for the success of your PCR results. By keeping these considerations in mind, researchers can enhance their chances of getting accurate and specific amplification of their desired DNA sequences.
Optimization Techniques
The optimization of primers in polymerase chain reactions (PCR) goes beyond just their design; it's a critical phase that can determine the success of your experiment. Optimization techniques are essential not only for achieving the desired amplification of your target sequence but also for enhancing specificity and yield. Focusing on optimization ensures that the primers work efficiently under various experimental conditions, which can vary significantly based on the target, template, and the overall setup.
With proper optimization, you minimize the risk of non-specific binding and other common pitfalls that can lead to wasted time and resources in the lab. Here’s a closer look at two vital optimization strategies: adjusting primer concentration and modifying cycling conditions.
Adjusting Primer Concentration
Primer concentration plays a pivotal role in the efficiency of PCR. Finding the right balance is akin to tuning an instrument; too much or too little can lead to discord.
- Low Primer Concentration: If the primer concentration is too low, there may not be enough primers to bind to the template DNA, which could yield a feeble or negligible amplification.
- High Primer Concentration: On the other hand, excessive concentrations can lead to non-specific binding, thereby increasing the chances of amplifying unintended sequences. This isn't just an inconvenience; it can muddle results and skew interpretations.
A common starting point is to use concentrations between 0.1 and 0.5 µM, but this can be adjusted based on specific needs and a hands-on understanding of the reaction dynamics.
To fine-tune this parameter:
- Begin with standard values and run a gradient PCR to assess the optimal concentration.
- Observe the amplification results, focusing on the clarity and intensity of bands on a gel.
- Adjust down or up based on these observations, as necessary.
“In PCR, precision is the name of the game.”
Modifying Cycling Conditions
Cycling conditions, typically dictated by the thermal cycler, are the backbone of any PCR. They encompass several variables that, when optimized, can greatly enhance the yield and specificity of your amplicons.
In a typical PCR, you pass through phases: denaturation, annealing, and extension. The duration and temperature of each phase are paramount for robust results:
- Denaturation Temperature and Time: Standard practice is to denature DNA at around 94°C. However, adjusting this can improve accessibility of primers to more complex templates, e.g., genomic DNA. A quick denaturation might work well for softer samples but can fail with tougher templates.
- Annealing Temperature: This temperature is critical for ensuring primers bind specifically to their target sequences. Too hot can cause them to leave too quickly; too cold may allow binding to non-target regions. A good starting place might be 5°C below the melting temperature (Tm) of the primers, but gradual optimization might reveal a better sweet spot.
- Extension Time: Generally, this is determined by the length of your target and the DNA polymerase's activity. Most enzymes extend at a rate of about 1 kb/min. If the target is particularly large, extend the time accordingly.
Ultimately, each adjustment may lead to a chain reaction of effects throughout the PCR process, making it crucial to monitor changes cohesively and iteratively.
In summary, tuning primer concentration and cycling conditions are powerful strategies that often set the stage for successful PCR amplification, and when merged with a precise understanding of the surrounding biochemistry, these steps become an indispensable part of primer design.
Troubleshooting Common Issues
Addressing complications that arise during PCR is an essential skill for any researcher or student involved in molecular biology. The next discussion centers on tackling common issues that may arise, particularly non-specific amplification and poor yield. Identifying and rectifying these problems benefits not just the immediate experiment, but all future work as well. Furthermore, understanding how to troubleshoot effectively can save wasteful resource expenditure and lessen frustration widely experienced in laboratory settings.
Non-specific Amplification
Non-specific amplification occurs when primers bind to regions outside the target sequence, leading to undesired PCR products. This scenario usually arises due to several factors, such as the characteristics of the primers themselves or the reaction conditions.
To minimize or prevent this issue, consider the following strategies:
- Design Specific Primers: Opt for primers that have minimal complementary sequences to other target regions. Tools like Primer3 or NCBI’s Primer-BLAST can be invaluable in this stage.
- Temperature Optimization: The annealing temperature is crucial. A temperature too low can allow non-specific binding, while excessive temperatures might hinder specific binding. Conduct a gradient PCR to identify the optimal annealing temperature.
- Improve Reaction Conditions: Adjusting salt concentrations or using specialized buffers can promote specificity. For instance, using a high-fidelity polymerase may also assist in reducing non-specific amplicons.
Innovatively, some labs are exploring the performance of dual-labeled probes to amplify only specific sequences, which can provide an answer to non-specific issues when faced with complex templates.
"A well-planned primer design can significantly reduce unexpected results during amplification."
Poor Yield and Specificity
Yield and specificity problems are like that thorn in your side you just can’t shake off. When you find yourself with low yields, it can be maddening, making it seem like all that hard work might as well just go down the drain. Poor yield often stems from various sources—primers, enzyme efficiency, or template quality and concentration. Here’s how to address these factors:
- Template Quality: Always assess the quality of your DNA or RNA template. Impurities, degradation, or low concentration can result in insufficient yields.
- Optimize Primer Design: While designing, ensure that your primers are not self-complementary or prone to dimerization. Such issues can affect the overall reaction output.
- Increase Template Quantity: If you’re consistently getting low yields and your primers are on point, consider upping your template amount slightly. However, avoid going overboard as this could lead to other complications.
Ultimately, both troubleshooting non-specific amplification and overcoming poor yield are pivotal for effective PCR. Familiarizing yourself with these common issues and their solutions can empower you to conduct more precise experiments, directly impacting your results and their application in research.
Innovations in Primer Technology
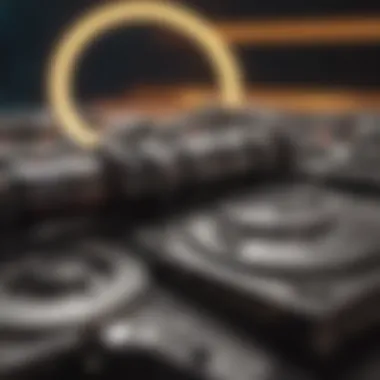
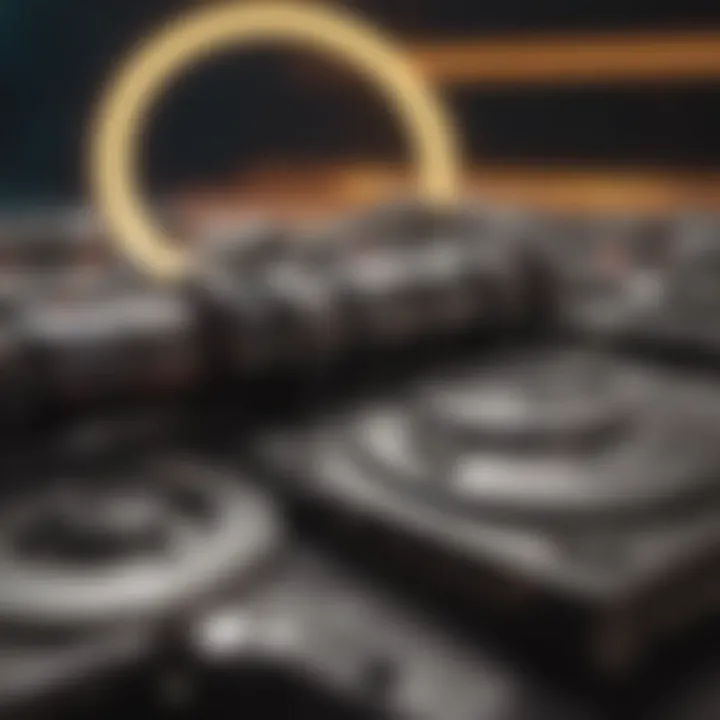
In the arena of molecular biology, the pace of advancement is staggering. Innovations in primer technology play a pivotal role in enhancing the efficacy and specificity of polymerase chain reactions (PCR). This section will unpack how modern breakthroughs in primer technology not only streamline the amplification process but also offer researchers unparalleled tools for genetic analysis.
One of the most significant aspects of advancements in primer technology is their ability to tackle the challenge of specificity. As PCR is sensitive to even minor variations in primer design, innovations have focused on improving primer performance in complex genetic environments. In this way, the selection and application of innovative techniques can aid in reducing the likelihood of non-specific amplification, which is often a headache for researchers.
As we journey through this section, we'll delve into two key innovations: dual-labeled probes and modified nucleotides. Both of these technologies bring distinct benefits to the forefront, allowing scientists to better navigate the often murky waters of genetic amplification. Let's explore them in detail.
Dual-labeled Probes
Dual-labeled probes have emerged as a game-changer in achieving precise detection of target sequences. These probes combine both a fluorescent dye and a quencher in the same strand of nucleic acid, which dramatically improves quantitative PCR (qPCR). The principle is simple yet effective: when the probe binds to the target sequence, the configuration changes, separating the fluorescent dye from the quencher. This separation leads to a measurable increase in fluorescence, which correlates directly to the amount of target DNA present in a sample.
The significance of dual-labeled probes lies in their ability to increase sensitivity and specificity. Traditional primers can struggle in complex mixtures where similar sequences abound; dual-labeled probes, however, offer enhanced discrimination. The benefits include:
- Increased sensitivity: Allows for detection of low-abundance targets.
- Real-time monitoring: Progress of the PCR can be tracked in real time.
- Multiplexing capability: Several targets can be monitored simultaneously using different fluorescent dyes.
Still, it’s vital to consider the optimal design of these probes, as poor pairing can lead to reduced efficiency. The balance of length and optimal melting temperatures must not be overlooked.
Modified Nucleotides
Modified nucleotides bring another layer of innovation to primer design. These nucleotides, which can incorporate subtle changes to the standard A, T, C, G bases, offer researchers the ability to enhance the performance of PCR reactions. Examples of modified nucleotides include phosphorothioate nucleotides, which confer stability against exonucleases, and locked nucleic acids (LNA), which increase binding affinity to target sequences.
Utilizing modified nucleotides can provide several distinct advantages:
- Enhanced specificity: Modified bases can improve binding to target sequences, reducing nonspecific amplification.
- Stability: They can enhance the longevity of primers during storage and in reaction conditions.
- Versatile applications: Facilitates the design of probes and primers tailored to specific detection methods, including SNP detection and gene expression analysis.
However, incorporating modified nucleotides must be done judiciously, as the cost can rise, and there's a learning curve associated with designing effective assays incorporating these novel elements.
"Investing in primer technology advancements could be seen as honing a craftsman's tools before embarking on a complex task. The right tools can elevate outcomes."
As the field continues evolving, both dual-labeled probes and modified nucleotides showcase the potential to not only refine PCR protocols but also broaden the scope of what can be achieved through molecular diagnostics. The integration of these technologies into standard practices indicates a bright future in genetic research, pointing towards greater accuracy, specificity, and efficiency in PCR applications.
Applications of PCR in Research
The polymerase chain reaction (PCR) has become a cornerstone in molecular biology, making significant waves in research and applied sciences. It's not just a lab technique but a powerful tool with diverse applications ranging from medical diagnostics to environmental studies. Understanding these applications is crucial as it sheds light on the practicality and impact of PCR in various fields. With the capability to amplify specific DNA sequences, PCR paves the way for innovations and breakthroughs.
There are a few specific elements and benefits to highlight:
- Enhanced Detection: PCR can identify minute amounts of DNA, a feature that is invaluable in clinical settings for diagnosing diseases. This sensitivity is something traditional methods often lack.
- Speed: Compared to conventional techniques, PCR drastically reduces the time needed for amplification and analysis. What once took days or weeks can now often be completed in a few hours.
- Versatility: The adaptability of PCR allows it to be utilized in various biological and environmental samples. From clinical blood tests to ancient DNA retrieved from fossils, PCR can cover a wide spectrum.
- Cost-Effectiveness: As the technology behind PCR has advanced, the costs associated with it have also seen a decrease, making it more accessible for routine laboratory use.
Above all, the precision and reliability of PCR are what make it a go-to method in research. It’s essential to consider the significance of optimizing primers in this process; effective primer design directly influences the accuracy of the assay that follows.
Genetic Testing and Diagnosis
In the realm of genetics, PCR stands as a vital technique for testing and diagnosis. When dealing with hereditary diseases or genetic disorders, PCR acts as a first responder. It simplifies the identification of genetic markers associated with diseases.
When clinicians suspect a genetic condition, they can use specific primers to target sequences known to be linked with the disorder. For instance, in cystic fibrosis, a common practice involves using PCR to amplify the CFTR gene to look for mutations. This targeted approach provides clinicians more options for diagnosing effectively and addressing the patient's needs.
The implications for patient care are profound. Early detection through PCR allows for timely intervention, which could be life-saving in certain conditions.
Also vital to consider is the role of PCR in prenatal genetic diagnosis. By analyzing fetal DNA present in maternal blood, doctors can identify possible genetic anomalies without invasive procedures. This non-invasive aspect of PCR has revolutionized how medical practitioners approach prenatal care.
"PCR serves not just to identify diseases, but it offers a pathway for preventing potential health crises."
Environmental DNA Analysis
Shifting the lens toward environmental science, PCR has transcended typical laboratory boundaries to play a critical role in ecological conservation and biodiversity studies. Environmental DNA (eDNA) is genetic material obtained directly from environmental samples like soil, water, or air, and PCR is pivotal in analyzing this DNA.
For instance, eDNA can be used to monitor populations of endangered species. By collecting water samples from a lake, researchers can detect DNA from fish species present in that area without ever needing to capture them. This non-invasive method is beneficial not only for the species being studied but also for maintaining their habitat.
The applications extend to assessing ecosystem health. PCR analysis of eDNA can help scientists understand the presence and distribution of invasive species, helping to manage and mitigate their impact. Furthermore, it aids in tracking disease outbreaks among wildlife populations. Identifying pathogens through eDNA is invaluable for creating timely conservation strategies.
In summary, the applications of PCR in research are vast and varied, from genetic testing that impacts individual lives to environmental analyses that protect ecosystems. The development and refinement of primer design not only enhance our understanding but also drive innovations that can transform various fields of study.
Culmination and Future Directions
The conclusion and future directions of primer design encapsulate the essence of what has been discussed and set the stage for what lies ahead in this ever-evolving field. It is essential to step back and weigh the significance of effective primer design not just for today’s research, but also for the prospects of tomorrow’s scientific advancements. Primer design is foundational to the success of PCR and has a profound impact on various applications such as genetic testing and environmental monitoring.
In reviewing key elements of primer design, it becomes clear that understanding the underlying biochemical properties allows researchers to tailor their approaches effectively. Primers act as the essential starting block for amplification, and the precision with which they are crafted directly influences the specificity and yield of PCR products. Thus, the importance of meticulous design cannot be overstated—it’s not merely about sticking a few nucleotides together; it’s about strategic planning and consideration of myriad factors, including target sequence variability, secondary structure, and interaction with other components in the reaction milieu.
Looking ahead, it’s crucial to consider integrative and adaptable strategies to meet the challenges posed by complex biological systems. Innovations in technology, such as next-generation sequencing and real-time PCR, demand that primer design evolve too. Adopting flexible methodologies and computational tools could facilitate quicker iterations in design, allowing for real-time adjustments based on ongoing experimental data and findings.
"In the world of molecular biology, the only constant is change; adapting to these shifts is key in maintaining efficacy and relevance."
Thus, the journey of primer design is anything but static. To stay ahead, researchers must embrace continuous learning and development of best practices, validating their designs through rigorous testing and adopting cutting-edge technologies.
Ultimately, the path forward should involve collaboration between researchers, bioinformaticians, and technologists to push the boundaries of what PCR can achieve, ensuring that primer design not only addresses current challenges but also anticipates the needs of future scientific inquiry.
Summary of Key Points
In this article, we have traversed essential dimensions of designing forward and reverse primers, with a focus on framing the overarching significance as follows:
- Role of Primers: They are essential for the amplification of target sequences in PCR, highlighting their critical role in various scientific applications.
- Characteristics of Effective Primers: Successful primers possess specific attributes, including optimal length, melting temperature, and specificity to the target sequence.
- Design Techniques: Identifying target sequences, utilizing software tools, and evaluating candidates are crucial steps in crafting effective primers.
- Optimization and Troubleshooting: Adjusting conditions and addressing issues like non-specific amplification play vital roles in successfully executing PCR.
- Technological Innovations: The advent of modified nucleotides and dual-labeled probes expands the capabilities of PCR, altering how researchers approach primer design.
- Applications in Research: The utility of PCR spans genetic analysis, disease diagnosis, and even studying biodiversity through environmental DNA analysis.
Emerging Trends in Primer Design
Emerging trends in primer design reflect the rapidly advancing landscape of molecular biology and its applications. Key points worthy of note include:
- Integration with Bioinformatics: The role of computational biology is growing increasingly critical. Bioinformatic tools can automate and refine primer design, thus making the process more efficient and reducing the potential for human error.
- CRISPR-Cas Systems: Primer design relevant to CRISPR technologies is becoming vital. Researchers must design primers that accommodate the editing tools, leading to diverse applications in medicine and agriculture.
- Sustainable Approaches: The push for more eco-friendly methods in laboratory settings may also influence primer design, encouraging practices that reduce waste and enhance sustainability in scientific protocols.
- Adaptive Strategies: Real-time PCR necessitates dynamic adjustments in primer design based on input data from ongoing experiments which indicates the importance of flexibility in the design process.
All these trends usher in new possibilities, making it imperative for researchers to strategize and innovate continuously. Keeping abreast of these developments will not only enhance PCR efficacy but may also lead to breakthroughs in various fields, from genomics to environmental science.