Understanding the Size Range of Nanoparticles
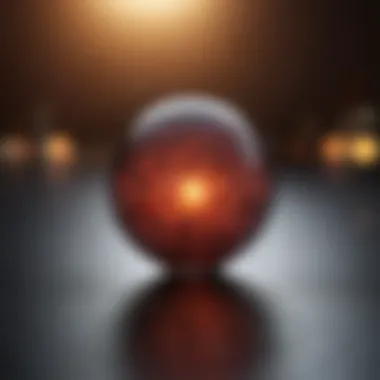
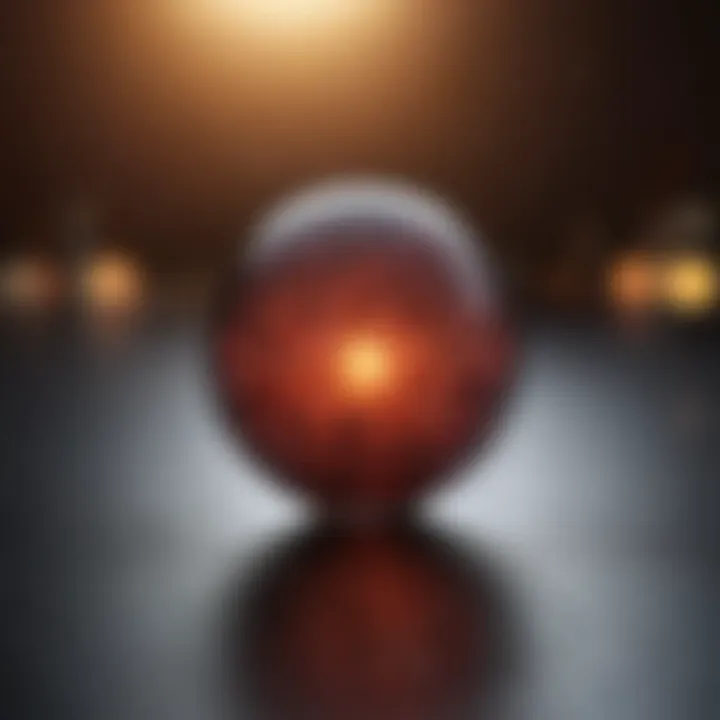
Intro
Nanoparticles, defined as particles measuring less than 100 nanometers, have garnered significant attention in recent years for their unique properties. These tiny structures are at the center of research and innovation across various fields, particularly in medicine, electronics, and materials science. Understanding the specific size range of nanoparticles is crucial, as it influences their functionalities and interactions at the molecular level.
Research Background
Overview of the scientific problem addressed
The wide array of applications for nanoparticles raises questions about how their size affects physical and chemical properties. Researchers often face challenges in characterizing these particles accurately. The dilemma lies in differentiating between nanoparticles and larger particles, which can lead to vastly different behavior. The need for precise size measurement methods is tied directly to the effectiveness of nanoparticles in real-world applications.
Historical context and previous studies
The concept of nanoparticles is not entirely new. Precursors to modern nanotechnology can be traced back to ancient practices. Artists in the 1200s utilized nanoparticle-based colors in stained glass. Fast forward to the 20th century, significant strides were made with the development of electron microscopes, enabling scientists to observe materials at the nanoscale. Subsequent research focused on understanding how size influences characteristics like reactivity, stability, and toxicity. Key studies in this area have established foundational knowledge while also highlighting remaining challenges in the field.
Findings and Discussion
Key results of the research
Research consistently shows that nanoparticle size can dictate behavior in multiple settings. For instance, in drug delivery, smaller nanoparticles tend to circulate longer in the bloodstream, improving bioavailability. In electronic applications, adjusting particle size can modify electrical conductivity. Furthermore, materials science has revealed that size impacts structural integrity and strength, necessitating careful consideration in engineering processes.
Interpretation of the findings
The findings underline a pivotal conclusion: size matters. The implications of size go beyond mere quantification; they resonate through effectiveness in application, safety profiles, and regulatory considerations. As the body of knowledge expands, researchers and professionals must remain informed about measurement techniques and the challenges involved in nanoparticle characterization. Accurate understanding of size is imperative for leveraging the capabilities inherent to nanoparticles.
"Nanoparticles represent a marvellous intersection of science and technology, where small scale can lead to big innovations."
In summarizing the significance of nanoparticle size, we can conclude that ongoing research is essential for unlocking new potentials in various industries. The continuous evolution in nanoparticle applications heralds a promising future, but requires rigorous understanding and scrutiny of size and its implications.
Foreword to Nanoparticles
Nanoparticles play a fundamental role in the realm of nanotechnology. Understanding their characteristics is essential, as they have unique properties that differ markedly from larger particles. This section aims to provide a concise overview of nanoparticles, their definitions, and their historical significance within the scientific community. These discussions lay the groundwork for grasping the implications of size in the fields of materials science, medicine, and electronics.
Defining Nanoparticles
Nanoparticles are particles that typically measure between 1 and 100 nanometers in size. A nanometer is one billionth of a meter, illustrating how minute these particles are. Due to this small size, nanoparticles exhibit phenomena that can be very different from those of bulk materials. For example, their huge surface area relative to their volume boosts interactions with other materials, enhancing their reactivity and strength.
Nanoparticles can be classified based on various criteria such as material composition. Common types include metal nanoparticles, semiconductor nanoparticles, and carbon-based nanoparticles like graphene. The definition extends beyond mere measurement, encompassing the behavior these particles exhibit at the nanoscale.
Historical Context of Nanotechnology
The history of nanotechnology dates back several decades. Notably, in the 1950s, physicist Richard Feynman delivered a famous lecture titled "There's Plenty of Room at the Bottom," presenting the idea of manipulating matter at the atomic level. However, practical advancements were slow until the late 20th century, when scientists developed techniques to synthesize and manipulate nanoparticles reliably.
The concept gained momentum through the 1990s, leading to increased research funding and academic interest. Breakthrough technologies, such as scanning tunneling microscopy, allowed scientists to observe and manipulate individual atoms and molecules, marking a significant stepping stone in the field.
Nanotechnology now encompasses a broad array of applications, from drug delivery in medicine to enhancing the properties of materials used in electronics. This evolution has paved the way for nanoparticles to become a crucial element of contemporary scientific inquiry and development.
The ongoing research in nanotechnology underscores its potential, influencing areas like renewable energy, environmental remediation, and targeted therapeutic treatments.
In summary, nanoparticles embody a fascinating intersection of size, material science, and application. As we explore their size range and implications in other sections of this article, the importance of understanding these foundations becomes evident.
Understanding Size in Nanotechnology
The size of nanoparticles plays a crucial role in their behavior and properties, which has significant implications for various fields such as medicine, electronics, and materials science. Understanding the size of nanoparticles allows scientists and researchers to manipulate their characteristics for specific applications. For instance, the surface area-to-volume ratio of nanoparticles increases as their size decreases, which influences reactivity and interaction with biological systems.
Moreover, the ability to precisely control the size of nanoparticles is vital in developing innovative technologies. By tailoring the size, researchers can enhance the effectiveness of drug delivery systems or improve the performance of electronic devices. Therefore, it is essential to delve deeper into the various size ranges and classifications of nanoparticles to comprehend their implications fully.
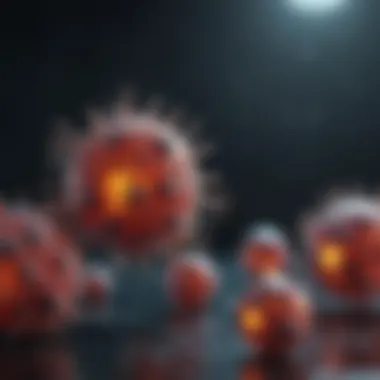
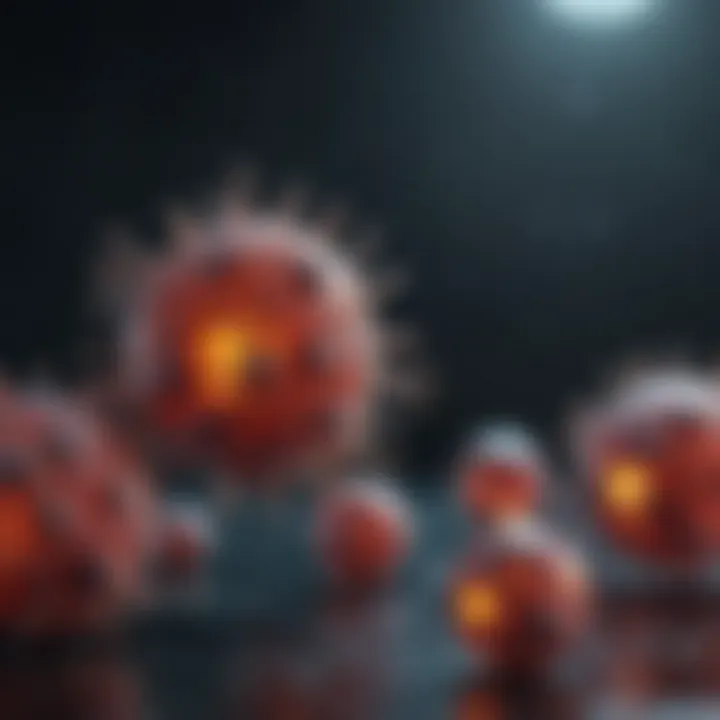
Nanoparticle Size Range
Nanoparticles generally range from 1 to 100 nanometers in diameter. This size range is critical because it distinguishes nanoparticles from bulk materials, which exhibit different physical and chemical properties. At the nanoscale, quantum effects become pronounced, leading to new and enhanced functionalities.
Particles in this range can influence their behavior in various environments. For example, nanoparticles in the lower end of the range often demonstrate increased mobility and can easily penetrate biological barriers, making them particularly useful in medical applications.
Additionally, size influences light absorption and scattering properties. This aspect is particularly relevant in applications like solar cells or imaging technologies, where tailored optical properties can be leveraged for performance enhancement.
Size Classifications
Fine Particles
Fine particles are generally classified as having a size range from 100 nanometers to 2.5 micrometers. While they are larger compared to nanoparticles, fine particles still exhibit unique properties that are useful in various applications. Their key characteristic is the ability to maximize surface area while maintaining relative structural integrity. This property makes them beneficial in fields such as pharmaceuticals, where they can enhance solubility and bioavailability of drugs.
One of the popular uses of fine particles is in the formulation of paints and coatings, where they can provide improved opacity and finish. However, fine particles may also pose challenges due to their tendency to agglomerate, leading to inconsistencies in material properties. Therefore, while fine particles offer certain advantages, they also require careful consideration in terms of formulation and application.
Ultrafine Particles
Ultrafine particles are smaller than fine particles, typically falling within the range of 1 to 100 nanometers. This specific size gives them distinct advantages, particularly in their high surface area-to-volume ratio. Ultrafine particles are increasingly used in diverse applications including catalysis, where their small size can greatly enhance reaction rates.
The unique feature of ultrafine particles is their heightened reactivity and ability to enter cellular structures, making them invaluable in targeted drug delivery systems. They can interact at a molecular level, often leading to effects that are not possible with larger particles.
However, the use of ultrafine particles comes with concerns regarding their environmental and health impacts. Due to their small size, they can easily enter the human body or the environment, leading to potential toxicity. Thus, while they offer substantial benefits, several factors must be considered, including safety and regulatory aspects.
Properties of Nanoparticles
The properties of nanoparticles are paramount in understanding their functionality and potential applications across various fields. When discussing nanoparticles, one must recognize that their unique attributes stem mainly from their small size and high surface area to volume ratio. These characteristics lead to notable differences in behavior when compared to bulk materials. In this section, we will delve into two primary areas: the influence of size on physical properties, and the chemical properties and reactivity of nanoparticles.
Influence of Size on Physical Properties
The size of nanoparticles has a profound impact on their physical properties. As the dimensions of a material decrease to the nanoscale, its characteristics can change significantly. Some physical properties that are commonly affected include:
- Melting Point: Nanoparticles often exhibit lower melting points compared to their bulk counterparts. This phenomenon arises due to the increased surface area relative to volume, resulting in greater energy fluctuations at the surface.
- Electrical Conductivity: The electrical properties of nanoparticles can vary dramatically. For instance, gold nanoparticles can be conductive at certain sizes, which makes them valuable in electronic applications.
- Optical Properties: Nanoparticles can display unique optical behaviors, such as size-tunable optical absorption and scattering. Quantum dots, for example, change color depending on their size, which is exploited in imaging and display technologies.
The implications of these changes in physical properties are vast. In fields like materials science and electronics, these attributes can be fine-tuned for desired outcomes. The study of nanoscale behavior allows researchers to design materials with specific characteristics, enhancing their functionality.
Chemical Properties and Reactivity
Nanoparticles exhibit distinct chemical properties compared to larger particles. Their high surface area promotes increased reactivity, making them highly effective in catalytic processes. Some key aspects of chemical properties include:
- Catalysis: Nanoparticles, such as platinum and palladium, are often used as catalysts due to their enhanced surface reactions. Their efficiency can lead to faster reaction times and lower energy usage in industrial processes.
- Surface Chemistry: The chemical behavior of nanoparticles is significantly influenced by their surface characteristics. Modifications to the surface can change interactions with other substances, offering avenues for applications like drug delivery or sensor technology.
- Biocompatibility: In medical applications, the chemical properties of nanoparticles determine their biocompatibility. The interaction between nanoparticles and biological systems is complex, where size, shape, and surface charge play critical roles in how they are received by cells.
"The uniqueness of nanoparticle properties opens new pathways for innovation across multiple disciplines."
Understanding these attributes of nanoparticles is crucial for their effective application in technology and medicine. As we proceed, we will explore specific applications and the implications of these chemical and physical properties in real-world situations.
Applications of Nanoparticles
Nanoparticles have significant applications across various fields. Their unique properties make them valuable in medicine, electronics, and environmental science. Understanding these applications enhances appreciation of their role in advancing technology and improving lives.
Medical Applications
Drug Delivery Systems
Drug delivery systems utilizing nanoparticles represent a transformative approach in medicine. These systems are designed to deliver medications precisely to affected cells, thereby maximizing therapeutic effects while minimizing side effects. The key characteristic of these systems is their ability to encapsulate drugs, protecting them from degradation. This ensures that the drug reaches its intended target effectively. Consequently, nanoparticles can increase bioavailability and enhance the solubility of poorly water-soluble drugs.
A notable unique feature of drug delivery systems is their ability to be functionalized. Different ligands can be attached to the surface of nanoparticles, enabling targeted delivery mechanisms. This advantage helps in treating conditions like cancer more effectively, where targeting tumor cells can result in better outcomes. However, it is essential to consider potential disadvantages, such as the complexity of formulation and the need for rigorous testing to ensure safety.
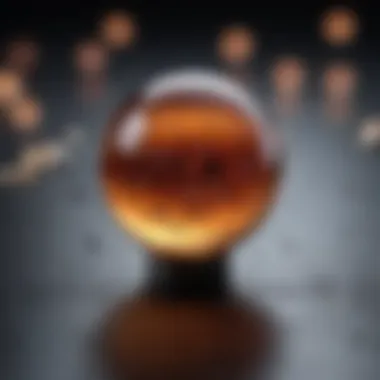
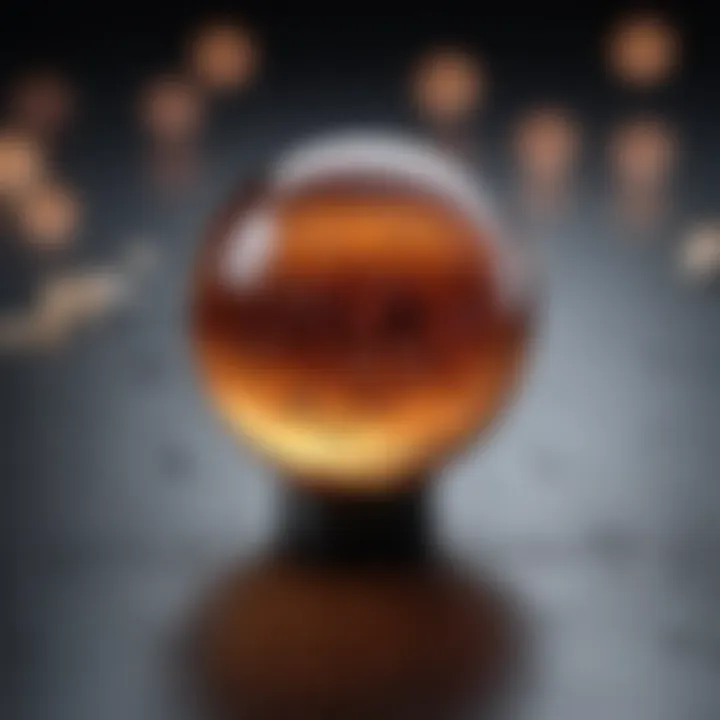
Diagnostic Tools
Diagnostic tools incorporating nanoparticles have revolutionized biomedical analysis. These tools assist in detecting diseases at an early stage, which is critical for timely treatment. For instance, nanoparticles are used in imaging techniques like magnetic resonance imaging (MRI) and positron emission tomography (PET). The key characteristic of these diagnostics is their high sensitivity and specificity, which enable the detection of low-abundance biomarkers.
A unique feature is their ability to provide real-time results, aiding quick clinical decision-making. This advantage often leads to better patient management and outcomes. However, challenges include potential false positives and the need for substantial validation to assure accuracy in clinical settings.
Nanoelectronics
The field of nanoelectronics examines the integration of nanoparticles in electronic devices. Nanoparticles can improve the performance of transistors, sensors, and other electronic components. Their small size and high surface-to-volume ratio allow them to exhibit novel electronic properties. This advantage can lead to miniaturized devices with superior performance metrics.
The overall potential of nanoparticles in enhancing electronic devices cannot be overstated. As electronic components become smaller and more efficient, nanoparticles will play a pivotal role in paving the way for advancements in information technology and communication.
Environmental Applications
Nanoparticles also hold great promise in environmental applications. They can be used for water treatment, remediation of contaminated sites, and as catalysts in chemical reactions. Their small size and high reactivity enable them to interact effectively with pollutants, aiding in their breakdown and removal from various environments.
Using nanoparticles for environmental cleanup not only improves efficiency but also reduces the need for harsh chemicals, making processes more environmentally friendly. As researchers explore new formulations and interactions, the application of nanoparticles in this area will continue to expand.
Measurement Techniques for Nanoparticle Size
Nanoparticle size characterizations are crucial in ensuring the desired properties and functionalities in diverse applications. The size of nanoparticles influences their behavior, reactivity, and interactions at the molecular level. Thus, precise measurement techniques are essential. By employing accurate methods, researchers can develop nanoparticles that meet specific criteria for their intended use. This section focuses on the principal techniques used for measuring nanoparticle size, outlining their importance, benefits, and key considerations.
Dynamic Light Scattering
Dynamic Light Scattering, commonly abbreviated to DLS, is a primary technique used for measuring the size of nanoparticles in suspension. DLS analyzes the scattering of laser light by particles in a liquid medium. As the nanoparticles move, the light scattering pattern changes. Analyzing these fluctuations provides vital information on particle size.
One significant benefit of DLS is its rapid measurement process. It allows for quick assessment of the size distribution of nanoparticles, which is particularly beneficial in quality control and research settings. DLS can measure sizes ranging from a few nanometers up to several micrometers, making it versatile for various applications. However, DLS may have limitations in accurately measuring polydisperse samples, where the presence of multiple sizes can complicate the data interpretation.
Electron Microscopy Techniques
Electron microscopy techniques are among the most powerful tools for visualizing and measuring nanoparticles at the nanoscale. There are two prominent methods in this category: Transmission Electron Microscopy (TEM) and Scanning Electron Microscopy (SEM).
Transmission Electron Microscopy (TEM)
Transmission Electron Microscopy (TEM) is a sophisticated technique that allows for high-resolution imaging of nanoparticles. It utilizes electrons transmitted through a thin sample to create detailed images. The high energy of the electrons provides exceptional resolution, enabling the observation of fine structural details at the atomic level.
A key characteristic of TEM is its ability to provide both morphological and dimensional information about nanoparticles. This makes it a beneficial choice for investigating the precise size and shape of nanoparticles, which is essential for understanding their properties and behaviors. The ability to directly visualize nanoparticles in their native state is a unique feature of TEM that distinguishes it from many other measurement techniques.
However, TEM has some disadvantages. Sample preparation can be complex, requiring the sample to be extremely thin. This may not always be feasible for all types of nanoparticles. Moreover, the technique can be time-consuming, which may be a consideration for research needing rapid results.
Scanning Electron Microscopy (SEM)
Scanning Electron Microscopy (SEM) is another valuable technique used to analyze nanoparticles. SEM scans a focused beam of electrons across the surface of a sample, generating detailed images based on the emitted secondary electrons. This method excels in providing three-dimensional images of the sample surface.
A prominent trait of SEM is its versatility and user-friendly operation. It can quickly give qualitative and quantitative information about the sample's surface morphology, composition, and size. This ability makes SEM a popular choice in various fields, especially in material science and life sciences.
Yet, SEM also has limitations. While it can provide detailed surface images, it does not provide internal structural information like TEM. Additionally, samples may need to be coated with a conductive material, which could alter the native properties of sensitive nanoparticles.
"Precise measurement techniques are vital in tailoring nanoparticles for applications, with each method offering unique advantages and limitations."
In summary, various measurement techniques exist for nanoparticle size characterization, each with specific advantages and drawbacks. Understanding these methods allows researchers to make informed decisions to ensure accurate nanoparticle development, leading to better applications in medicine, electronics, and environmental science.
Challenges in Nanoparticle Size Characterization
Characterizing the size of nanoparticles presents several challenges that have important implications for both research and application. Understanding these challenges is crucial for ensuring accuracy and reliability in nanoparticle studies. Size can influence the properties and behavior of nanoparticles significantly, which is why precise measurement is essential. In this section, we will explore the limitations of current measurement methods and the issues surrounding reproducibility.
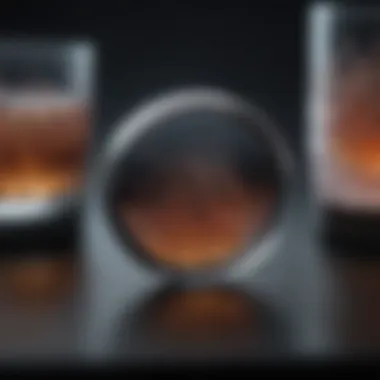
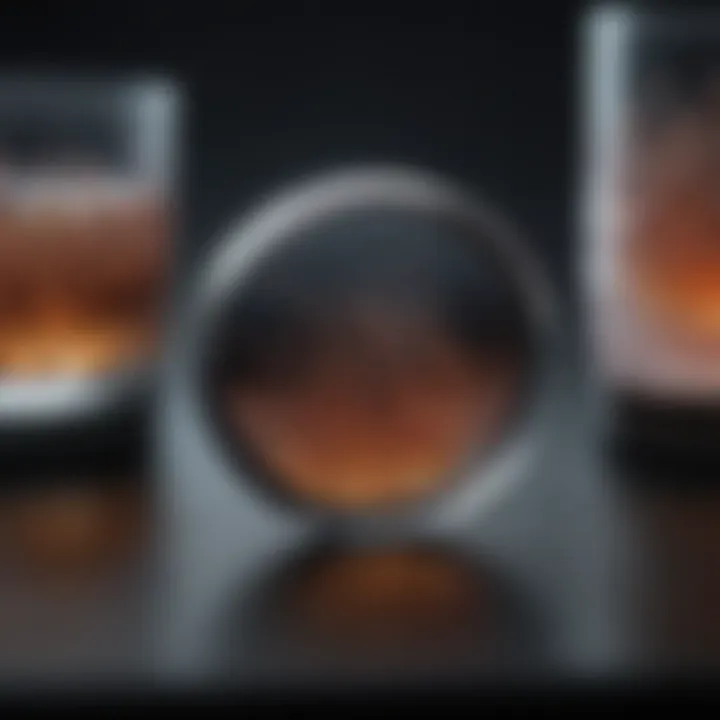
Limitations of Current Measurement Methods
Current measurement techniques for nanoparticle size characterization each have specific limitations. These methods commonly include Dynamic Light Scattering (DLS), Electron Microscopy (EM), Photon Correlation Spectroscopy (PCS), and others. While these approaches provide valuable insights, they are not without their drawbacks.
- Dynamic Light Scattering: This technique relies on the Brownian motion of particles. However, it may not effectively differentiate between aggregates and individual nanoparticles, leading to an overestimation of sizes.
- Electron Microscopy: Techniques like Transmission Electron Microscopy (TEM) can provide high-resolution images of nanoparticles. However, sample preparation for TEM can alter the nanoparticle sizes, introducing measurement bias. Additionally, the size distribution obtained may not represent the bulk sample accurately.
- Photon Correlation Spectroscopy: This method also shares issues related to particle aggregation and assumes spherical particles. Non-spherical shapes can lead to misinterpretation of size data.
These limitations indicate a necessity for better-standardized methods that can robustly characterize nanoparticle dimensions. A combination of methods may offer a clearer understanding, but that can complicate the analysis.
Reproducibility Issues
In scientific research, reproducibility is a significant aspect that validates experimental findings. However, when it comes to nanoparticle size characterization, issues consistently arise. Factors that contribute to reproducibility difficulties include the diversity of methods used, variation in the nanoparticle synthesis process, and inconsistency in the material properties.
Key elements contributing to these issues include:
- Preparation Variability: Changes in synthesis techniques can lead to different nanoparticle sizes, affecting results across experiments.
- Measurement Conditions: Parameters such as temperature and concentration can vary from one study to another, influencing the outcomes of size measurements significantly.
- Instrument Calibration: Differences in equipment calibration and settings can produce inconsistent results, leading to confusion in interpreting data.
Inconsistent measurements undermine the reliability of data, which is critical for advancing knowledge in nanotechnology.
Addressing these reproducibility issues requires strict adherence to standardized protocols and increased collaboration among researchers focusing on nanoparticle studies.
Having a clear understanding of these challenges and actively addressing them allows for improved characterization and facilitates advancements in nanotechnology research and application.
Future Perspectives in Nanoparticle Research
The future of nanoparticle research holds significant promise, anchored in ongoing innovations in measurement technologies and emerging applications. As we explore this evolving landscape, it will be critical to understand the potential benefits and considerations of these advancements, which can redefine how we approach various scientific and industrial challenges. Among the key elements are the continuous development of innovative measurement technologies that enhance our ability to characterize nanoparticles accurately, alongside the expanding horizon of their applications in diverse fields.
Innovative Measurement Technologies
As nanoparticles continue to gain traction in scientific research, the need for precise measurement techniques intensifies. Emerging technologies such as nanoparticles tracking analysis and high-resolution electron microscopy are pivotal. They allow researchers to obtain reliable size distributions and structural information that was previously challenging to achieve.
- Nanoparticle Tracking Analysis (NTA): This method utilizes light scattering and video microscopy to determine the size and concentration of nanoparticles in solution. It allows for real-time insights into particle behavior, offering a significant advantage in dynamic studies.
- Atomic Force Microscopy (AFM): AFM provides topographical mapping at the nanoscale. This allows scientists to visualize the surface characteristics of nanoparticles, providing essential information on their mechanical properties.
- X-ray Diffraction (XRD): A relevant method for crystallography, enabling the determination of crystalline structures of nanoparticle samples. XRD enhances understanding of their physical properties through structural identification.
These innovations make significant strides in elucidating the characteristics of nanoparticles, helping foster advancements in a variety of applications.
Emerging Applications and Trends
Nanoparticles are poised to revolutionize numerous industries, reflecting broader trends in research and technology. As we navigate this shifting terrain, several emerging applications stand out:
- Smart Drug Delivery Systems: Nanoparticles can encapsulate therapeutic agents, enhancing targeted delivery and reducing side effects, which marks a shift in pharmaceutical development.
- Sustainable Energy Solutions: The integration of nanoparticles in solar cells and catalysts has the potential to increase efficiency and reduce environmental impact. For instance, titanium dioxide nanoparticles are employed to improve solar energy conversion rates.
- Novel Materials Development: Nanoparticles are facilitating the creation of lighter, stronger materials. The incorporation of carbon nanotubes in composites is a prime example, leading to advancements in aerospace and automotive sectors.
- Environmental Remediation: Using nanoparticles in filtering systems is gaining attention. They can effectively remove contaminants from water and soil, addressing critical environmental issues.
"The rapid evolution of nanoparticle technologies fosters not only innovation but also the potential for transforming industries through enhanced performance and sustainability."
Closure
The conclusion of this article encapsulates the critical insights gained regarding nanoparticles and their size. Understanding the size range of nanoparticles is paramount to appreciating how these tiny particles interact with the surrounding environment. The unique properties displayed by nanoparticles are often size-dependent. This characteristic underscores the significance of accurate size determination techniques.
In summary, nanoparticle size influences various aspects of their behavior, including reactivity, stability, and effectiveness in applications. Researchers and practitioners benefit from a clear grasp of these principles, allowing them to innovate and tailor nanotechnology solutions that meet specific needs, particularly in fields like medicine and materials science.
"Size is not just a number; it can determine a nanoparticle's function and efficiency."
By synthesizing the key findings of this article, we can infer that a well-rounded understanding of this subject is essential for further advancements in nanotechnology. The implications of nanoparticle size range extend into every aspect of research and development, from basic scientific exploration to real-world applications.
Summary of Key Findings
The detailed examination of nanoparticle size reveals several significant points:
- Definition: Nanoparticles are defined by their size, typically ranging from 1 to 100 nanometers.
- Properties: The size of nanoparticles plays a crucial role in determining their physical and chemical properties.
- Applications: Nanoparticles find utility across various domains, including medical applications such as targeted drug delivery and in electronics for enhanced performance.
- Measurement Techniques: Techniques such as dynamic light scattering and electron microscopy are vital for accurately determining nanoparticle size.
- Challenges: Current measurement methods face limitations that affect reproducibility and reliability in size characterization.
Implications for Future Research
Future research in nanoparticles must address several key areas to enhance the overall understanding and usefulness of these materials:
- Innovative Measurement Techniques: Developing more precise and reproducible methods of measuring nanoparticle size can improve research outcomes significantly.
- Broadening Applications: Investigate unexplored areas where nanoparticles can provide solutions, particularly in fields facing challenges like environmental issues or energy conservation.
- Interdisciplinary Approaches: Encourage collaboration across disciplines to enhance the knowledge base and application for nanoparticles.
- Standardization: Work towards establishing universally accepted guidelines for nanoparticle size measurement and characterization.