Exploring Techniques for Graphene Production
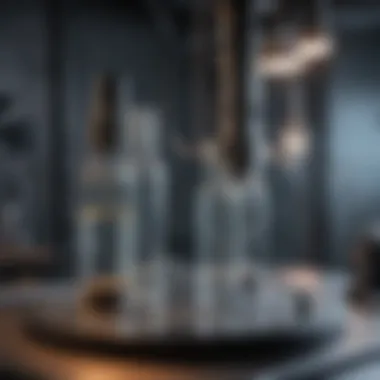
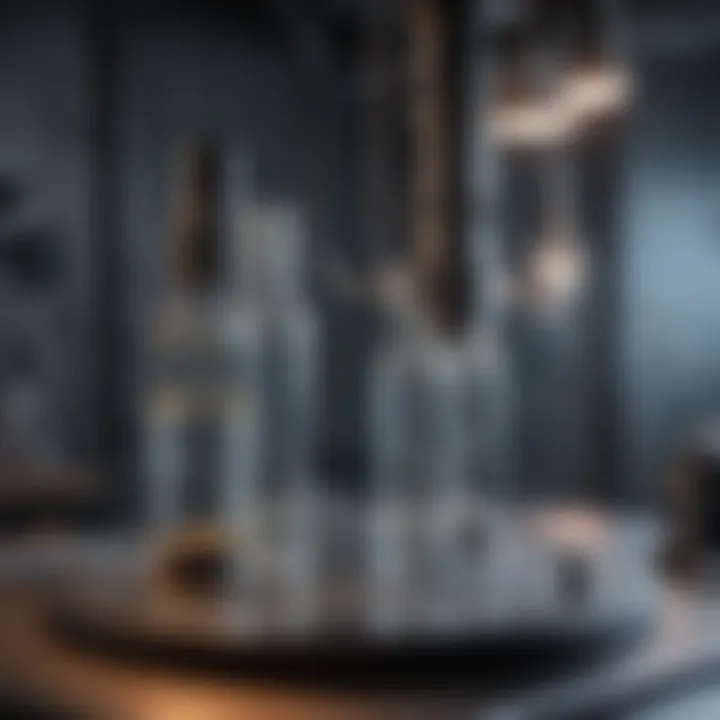
Intro
Graphene, a two-dimensional material composed of a single layer of carbon atoms, has attracted significant attention from researchers and professionals alike. Its unique properties, such as high conductivity, exceptional strength, and flexibility, have opened up numerous avenues for research and various applications. However, the challenge lies in producing graphene efficiently and sustainably. The article presents a detailed overview of different methods used in graphene production, including their advantages and challenges. Understanding these methodologies not only sheds light on the production process but also highlights the potential future implications for technology and industry.
Research Background
Overview of the Scientific Problem Addressed
The quest for economical and scalable methods of producing graphene is a pressing issue in material science. Traditional techniques often struggle to deliver high-quality graphene at scale. As industries increasingly look to integrate graphene into products—from electronics to composites—the demand for robust production techniques grows. The scientific problem hinges on balancing quality, yield, and scalability.
Historical Context and Previous Studies
Graphene was first isolated in 2004 by Andre Geim and Konstantin Novoselov, winning them the Nobel Prize in Physics in 2010. Initially, methods like mechanical exfoliation allowed for small quantities of graphene, but advancements occurred rapidly. Other techniques, such as chemical vapor deposition (CVD) and liquid phase exfoliation, gained recognition over time. Research has focused on optimizing these methods for better output and quality. Studies have revealed that while CVD produces high-quality graphene suitable for electronic applications, liquid phase exfoliation is more suitable for large-scale production with a compromise on quality. This historical context sets the stage for examining current methodologies and their implications.
Findings and Discussion
Key Results of the Research
The study of graphene production methods has yielded some significant findings. Key production techniques include:
- Chemical Vapor Deposition (CVD): This technique provides high-quality graphene suitable for numerous applications but is more complex and expensive.
- Mechanical Exfoliation: While this delivers high-quality graphene, the scalability is limited.
- Liquid Phase Exfoliation: This method is scalable but typically produces graphene with defects, affecting its properties.
Interpretation of the Findings
The interpretation of these results indicates a trade-off between quality and scale in graphene production. While techniques like CVD are preferable for applications requiring high-performance materials, traditional methods can suffice in applications where some compromise on quality is acceptable. Ongoing research aims to refine these methods further, potentially leading to hybrid techniques that enhance both quality and yield. The findings are crucial as they guide the path forward for more widespread applications of graphene across various industries.
"Challenges in graphene production highlight the need for innovative methodologies that meet both quality and scalability to unlock its full potential."
As companies look forward to integrating graphene into their products, understanding these nuances provides a clearer map for navigating the complexities of material production.
Prolusion to Graphene
Graphene stands as a remarkable material in the realm of scientific research and industry. Its unique properties, such as exceptional electrical conductivity, mechanical strength, and thermal conductivity, have made it a focal point for numerous studies and applications. In this section, we will discuss why understanding graphene is paramount in the context of this article. The insights explored here lay the foundation for comprehending various synthesis techniques and their implications.
Graphene, as a two-dimensional allotrope of carbon, presents a vast array of applications and potentials across multiple disciplines. Researchers emphasize the importance of graphene in energy, electronics, and materials science due to its impressive characteristics. Moreover, the transition from theoretical possibilities to practical applications demonstrates the significance of mastering graphene production techniques.
The study of graphene is not just about its fundamental properties. It also involves exploring the synthesis methods that make the material accessible for use in various sectors. The techniques range from simple mechanical approaches to complex chemical processes. Each method carries its own set of challenges and benefits, which need careful consideration when aiming for scalable production.
Definition and Properties
Graphene is defined as a single layer of carbon atoms arranged in a hexagonal lattice. This atomic configuration contributes significantly to its extraordinary properties. For example, graphene is about 200 times stronger than steel while being incredibly lightweight, making it exceptionally appealing for structural applications. Additionally, its electron mobility is much higher than that of silicon, marking it as a potential game changer in the electronics sector.
Key properties of graphene include:
- High electrical conductivity: It allows for efficient electron transport, which is crucial in semiconductor and conductive applications.
- Excellent thermal management: This property is important for electronic devices that require good heat dissipation.
- Flexibility: Graphene can be bent without breaking, leading to innovative uses in flexible electronics.
Historical Context
The history of graphene dates back to the early 2000s when it was isolated for the first time. In 2004, Andre Geim and Konstantin Novoselov succeeded in isolating graphene using a method known as mechanical exfoliation. This groundbreaking work earned them the Nobel Prize in Physics in 2010. Their contributions opened new avenues for research and sparked interest in exploring graphene's properties further.
Since then, numerous synthesis techniques have been proposed, representing a significant evolution in our understanding of graphene. Each method's development reflects not only scientific ingenuity but also the growing demand for innovative materials across various industries. Understanding this historical backdrop is crucial to appreciating the contemporary landscape of graphene production.
"The unique properties of graphene signal a historic moment not merely for material science but for technological advancement across sectors."
In summary, the introduction to graphene establishes a clear context for its study. Its definition, properties, and historical evolution provide insights necessary for understanding the deeper aspects of graphene production techniques. This foundational knowledge sets the stage for a comprehensive exploration of synthesis methods and their relevance in practical applications.
Significance of Graphene in Modern Science
Graphene stands out in modern science due to its distinct physical and chemical properties. This carbon allotrope offers exceptional strength, conductivity, and flexibility. Because of these attributes, graphene is becoming essential in various fields, revolutionizing traditional approaches and creating opportunities for advancements.
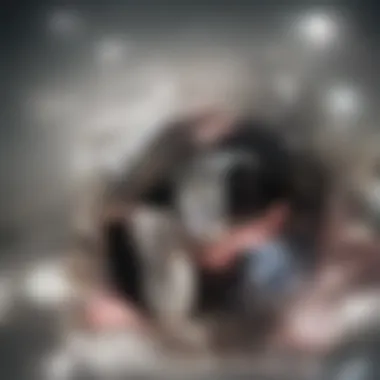
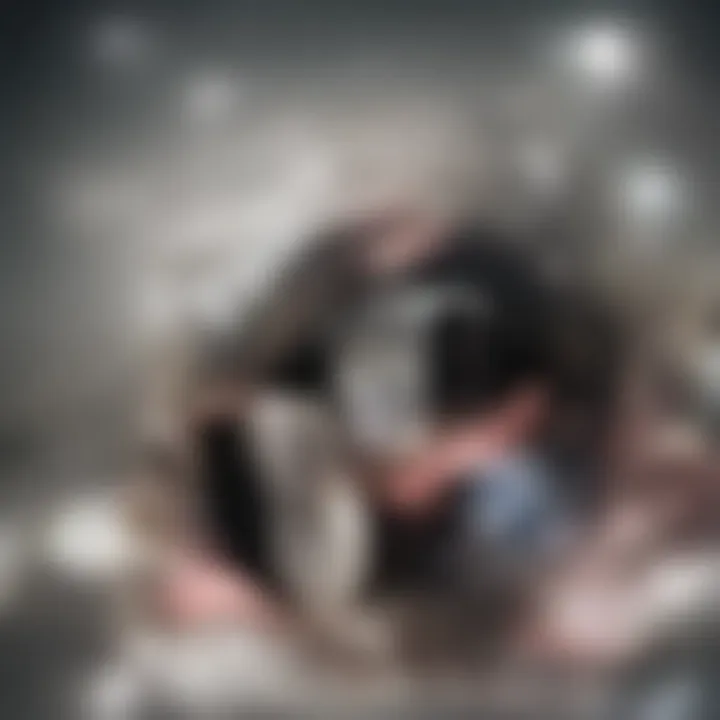
One of the key elements in the significance of graphene is its versatility. Researchers are investigating a range of applications, from electronics to materials science. This adaptability ensures that the relevance of graphene persists as technologies evolve. Furthermore, its atomic thickness gives it unique interactions with light and matter, leading to potential applications in optics and photonics.
Graphene-based materials have shown promise in enhancing energy storage solutions. For instance, when integrated into batteries, they can significantly improve charge capacity and charging speed. This feature is crucial, considering the global pivot toward renewable energy and the quest for highly efficient energy storage systems.
"Graphene’s unique properties are enabling advancements across numerous fields, impacting everything from consumer electronics to advanced medical devices."
Moreover, sustainability remains a vital consideration in the discussion around graphene. As concerns about environmental impact grow, the eco-friendly synthesis of graphene becomes more important. Studies demonstrate that certain methods, such as liquid-phase synthesis, are more sustainable compared to traditional methods, reducing chemical waste and energy consumption.
Buoyancy of Graphene Applications
Graphene applications are numerous and expand daily. Projects leveraging graphene's physical properties are underway in various industries. The electronics field, for example, benefits from graphene's conductivity. This material allows for lighter, more efficient components, enhancing the performance of devices.
Key areas utilizing graphene include:
- Flexible electronics: Graphene's flexibility enables the creation of bendable screens and wearable technology.
- Conductive inks: Utilized in printing electronics, these inks can be implemented for low-cost production of electronic circuits.
In energy sectors, graphene improves the efficiency of solar cells and supercapacitors. These capabilities represent an essential step in producing greener technologies.
Additionally, graphene's robustness finds it employed in composite materials. Industries like automotive and aerospace are exploring graphene’s potential to enhance strength without adding significant weight.
Role in Emerging Technologies
Emerging technologies often require innovative materials. Graphene is at the forefront of this need. Its atomic structure and unique properties open doors for new developments in quantum computing, photonics, and biomedicine.
In quantum computing, its properties could facilitate better qubit performance and stability, essential for building practical quantum systems.
In biomedicine, researchers are studying graphene for drug delivery and biological sensing. Its biocompatibility and surface-area properties allow for targeted medication delivery, improving treatment efficiency.
Overall, graphene is not merely an improvement over existing materials, but rather a catalyst for rethinking how technologies can evolve. As research continues, the range of applications will likely increase, demonstrating the far-reaching implications of this remarkable material.
Graphene Synthesis Techniques
Graphene synthesis techniques are crucial in the production process of graphene, affecting the quality, scalability, and cost-efficiency of the final product. Understanding these methods offers insights into choosing the appropriate technique based on desired applications and economic considerations. With the increasing interest in graphene's unique properties such as conductivity, mechanical strength, and flexibility, exploring diverse synthesis methods becomes paramount. This article focuses on key techniques, discussing their pros and cons in the context of producing high-quality graphene.
Exfoliation Methods
Exfoliation is among the most common techniques used to produce graphene. It involves separating layers of graphite to obtain monolayers or few-layer graphene. This section will detail three primary exfoliation methods: mechanical, chemical, and liquid phase.
Mechanical Exfoliation
Mechanical exfoliation is a straightforward method where bulk graphite is physically peeled apart. One significant aspect of this method is its ability to produce high-quality graphene sheets. This characteristic makes mechanical exfoliation a popular choice, particularly in research settings. The unique feature of this method lies in its simplicity; it does not require complex chemical processes.
However, mechanical exfoliation comes with limitations. It is not easily scalable for industrial production. This makes it suited mainly for small quantities or laboratory experimentation. Despite that, its high yield of defect-free graphene remains a major advantage in this context.
Chemical Exfoliation
Chemical exfoliation utilizes chemical reactions to break apart graphite layers. This technique is essential in producing graphene at a larger scale compared to mechanical means. Its key characteristic is the introduction of functional groups, which can enhance the properties of graphene.
A notable advantage is efficiency; large quantities can be synthesized within a short time. This method is flexible, allowing for various approaches depending on desired outcomes. However, a downside is the potential introduction of chemical residues which may affect graphene's properties, complicating post-processing steps.
Liquid Phase Exfoliation
Liquid phase exfoliation employs solvents to disperse graphite and facilitate the separation of layers. The main contribution of this method is its scalability; it can produce significant amounts of graphene suitable for industrial applications.
The key feature of liquid phase exfoliation is its adaptability. Various solvents can be used, allowing for tuning of the process. An advantage of this method is its capability to yield graphene in suspension, which can be easily processed afterward. Nevertheless, the quality of graphene produced may vary, and impurities can be a concern.
Chemical Vapor Deposition ()
Chemical Vapor Deposition is a favored method for producing high-quality graphene. CVD involves the deposition of carbon atoms onto a substrate through gas-phase precursors. The strength of this technique lies in its ability to produce large areas of high-quality graphene with fewer defects. CVD is used extensively in electronic applications because the resulting material exhibits excellent electrical properties.
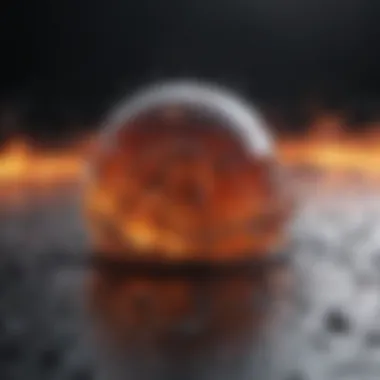
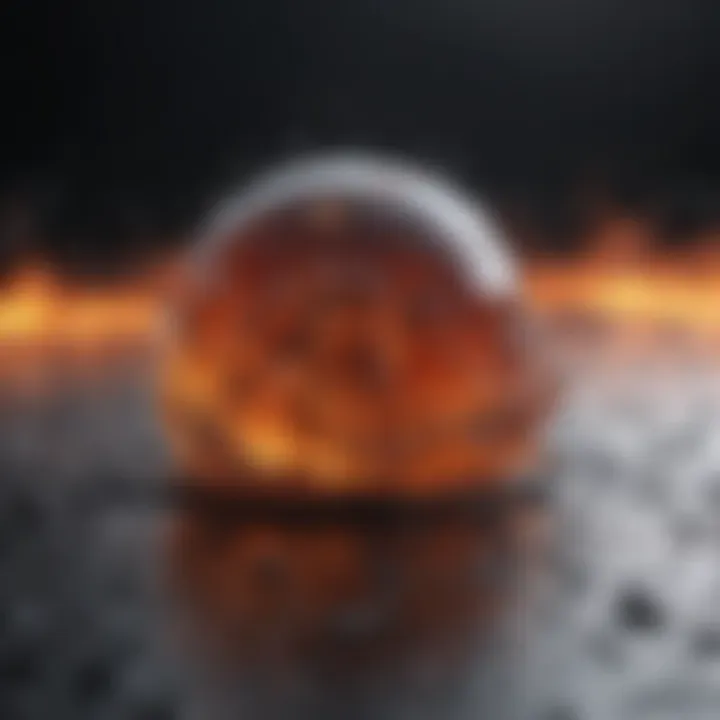
This method, however, demands significant technical expertise and sophisticated equipment, which can elevate production costs. Nonetheless, its scalability and ability to precisely control the thickness of the graphene make it invaluable for advanced applications.
Reduction of Graphene Oxide
The reduction of graphene oxide aims to restore the conductive properties of oxidized graphene. This technique involves chemically reducing graphene oxide to produce reduced graphene oxide. A key characteristic is its adaptability for various end uses, particularly in energy storage applications.
While useful, this method can introduce defects during the reduction process, affecting the electrical properties. Balancing the reduction to optimize performance is a challenge that researchers continue to address.
Liquid-Phase Synthesis
Liquid-phase synthesis incorporates various methods, including chemical and physical means, to create graphene directly in liquid environments. This technique allows for the easy manipulation of graphene characteristics during production. The flexibility in tailoring properties is its most significant contribution to the overall method.
A downside is that achieving high-quality graphene can be difficult. Control over the size and properties of the graphene produced often varies. Continuous advancements in the liquid-phase approaches aim to address these issues, making this an ongoing area of study.
Epitaxial Growth on Silicon Carbide
Epitaxial growth on silicon carbide involves growing graphene layers on a silicon carbide substrate. This method yields high-quality graphene and benefits from the compatibility of silicon carbide in electronic applications.
The primary advantage is the exceptional quality of graphene produced, as it often exhibits fewer defects and better electronic properties. However, the process requires expensive substrates and advanced techniques, which limits its broader applicability. The trade-off between quality and cost is a critical consideration when evaluating this method.
Overall, understanding these graphene synthesis techniques provides a foundation for further developments in its application across various industries. Each method serves specific needs and comes with its unique characteristics, advantages, and challenges. The choices made in the synthesis stage directly influence the quality and viability of graphene for future innovations.
Challenges in Graphene Production
The production of graphene, despite its potential, is fraught with challenges that can significantly impact both the viability and the scalability of its applications. Understanding these challenges is crucial for advancing research and development in this field. Addressing issues such as scalability, quality control, and cost can pave the way for more widespread implementation of graphene in a variety of technologies. Therefore, a nuanced discussion of these challenges illuminates the path forward for researchers and professionals engaged in material sciences and engineering.
Scalability Issues
Scalability in graphene production refers to the ability to produce large quantities of graphene consistently. This is essential for industrial applications but remains a significant bottleneck. Methods that work well at the lab scale often fail to translate to larger operations. For instance, mechanical exfoliation produces high-quality graphene but is not suitable for mass production due to its labor-intensive nature. Meanwhile, chemical vapor deposition (CVD) allows for larger-area graphene films but requires sophisticated equipment and conditions. The challenge here lies in finding a balance between quality and quantity, which is necessary for diverse applications. As production demands grow, researchers must innovate scalable methods that do not compromise on the properties of graphene, such as its strength and electrical conductivity.
Quality Control
Quality control is paramount in the production of graphene. Variations in material properties can lead to discrepancies in performance across applications. Key factors that affect quality include the presence of defects, layer thickness, and uniformity. For instance, graphene oxide may be easier to produce but lacks some of the superior properties of pristine graphene. Inconsistent quality can undermine the credibility of graphene products and their acceptance in the market. Developing robust techniques for quality assessment is essential. Advanced characterization methods such as Raman spectroscopy and atomic force microscopy can provide insights into the graphene’s structural integrity. Consistency in quality not only boosts reliability but also enhances the potential for commercialization in electronics and other fields.
Cost Considerations
The financial aspect of graphene production plays a critical role in determining its accessibility and adoption. High production costs can deter industries from investing in graphene technologies, despite their benefits. Factors contributing to these costs include raw material prices, the complexity of production processes, and scale. For example, while some methods may yield high-quality graphene, they may do so at exorbitant costs that are not feasible for most industries. As a response, researchers and companies are working on reducing production costs through innovative approaches. Techniques such as liquid-phase exfoliation and continuous production methods have emerged as potentially more cost-effective solutions. However, balancing cost reduction with the intrinsic properties of graphene remains a challenge.
Overall, the challenges in graphene production are multifaceted and interconnected. Addressing these hurdles requires a comprehensive strategy that combines technological innovation, rigorous quality assurance, and economic feasibility.
Applications of Graphene
Graphene has burgeoned into a material of significant interest due to its unique properties and versatility, leading to a vast array of applications. The importance of exploring these applications lies in understanding how graphene can revolutionize various industries. This section will discuss key areas where graphene's exceptional characteristics provide a competitive edge.
Electronics and Conductors
Graphene's electrical conductivity is among the highest of any known material. Its ability to carry electrical currents with minimal resistance makes it a prime candidate for various electronic applications. In the context of transistors, graphene can potentially replace silicon, which could enable the development of faster and smaller devices.
- Thin-Film Transistors: Graphene-based transistors exhibit higher switching speeds than traditional silicon counterparts.
- Flexible Electronics: The malleability of graphene allows for the development of flexible electronic devices, such as bendable smartphones and wearables.
- High-Frequency Applications: Due to its high electron mobility, graphene can operate efficiently at microwave frequencies.
The incorporation of graphene into existing technologies promises improved performance and energy efficiency.
Energy Storage Solutions
Energy storage capacities can be enhanced significantly through graphene applications. Graphene-based materials can improve battery and supercapacitor performance.
- Batteries: Graphene can facilitate faster charging and discharging cycles in lithium-ion batteries, leading to longer-lasting energy storage solutions.
- Supercapacitors: The symmetrical structure of graphene allows it to store large amounts of energy, making it a perfect candidate for next-generation supercapacitors, which have higher energy densities than conventional designs.
- Fuel Cells: Graphene can enhance the efficiency of fuel cells through its catalytic properties.
These advancements may help meet increasing energy demands while addressing sustainability concerns.

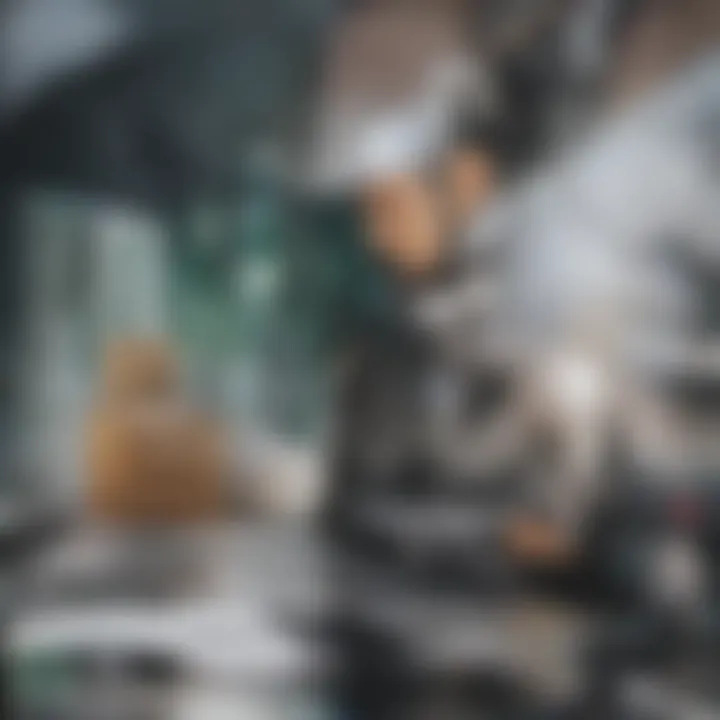
Biomedical Applications
Graphene's potential in biomedical fields is expansive, especially in drug delivery and biosensing.
- Drug Delivery Systems: Graphene can be engineered to carry drugs directly to targeted cells, improving treatment efficacy while minimizing side effects.
- Biosensors: The sensitivity of graphene enables the detection of biological markers with high precision and speed, which can revolutionize diagnostic processes.
- Tissue Engineering: Graphene’s biocompatibility makes it suitable for creating scaffolds in tissue engineering, potentially aiding in regenerative medicine.
Every application in this domain must consider safety and compatibility with biological systems.
Composite Materials
Graphene enhances the mechanical and thermal properties of materials when used as an additive.
- Strength Improvement: Composite materials reinforced with graphene exhibit significantly higher strength-to-weight ratios, ideal for aerospace, automotive, and construction applications.
- Thermal Conductivity: The addition of graphene increases thermal conductivity, allowing for better heat dissipation in electronic devices.
- Lightweight Materials: Graphene composites can reduce overall weight without sacrificing durability, which is crucial in various engineering sectors.
The development of graphene-enhanced composites may lead to breakthroughs in material science, with lasting impacts on design and engineering.
In summary, graphene demonstrates remarkable applications across diverse fields, presenting solutions that address contemporary challenges. The ongoing research and development will likely unveil even more innovative uses for this extraordinary material.
Future Trends in Graphene Production
Graphene stands at the frontier of materials science, and its production is evolving. Understanding these future trends is crucial for researchers, industry players, and educators. The emphasis on innovative production techniques, sustainability in synthesis, and regulatory considerations shapes this landscape. These elements not only redefine current methodologies but also pave the way for further advancements. They demonstrate the ongoing importance of graphene in various applications and how its production will likely adapt to meet future demands.
Innovative Production Techniques
Research in the synthesis of graphene is continually advancing. New techniques emerge that could enhance production efficiency and quality. One such technique is the use of chemical vapor deposition (CVD), which can produce high-quality graphene films. Recent modifications in CVD have increased substrate compatibility and reduced defects, thus broadening its applications in electronics. Additionally, laser scribing has gained attention as a method for producing graphene from carbon sources. This technique uses focused laser energy to convert materials like carbon-based polymers into graphene sheets.
Another promising direction is the extraction of graphene from biomass. This aligns with the trend towards bioinspired materials, creating graphene from organic waste. Utilizing such resources not only addresses waste management but also taps into renewable perspectives in production. Each of these techniques carries the potential to significantly reduce costs and increase scalability.
Sustainability in Synthesis
Sustainability represents a vital focus in modern production methods. As industries increasingly prioritize environmental responsibility, graphene synthesis must evolve accordingly. This trend looks toward minimizing energy consumption and waste generation. Processes like liquid-phase exfoliation are considered more environmentally friendly than traditional methods. These techniques often use water- or solvent-based solutions and can lead to lower energy inputs.
Further, the exploration of green solvents has risen. Solvent use contributes to environmental pollution, so the development of eco-friendly options is essential. Researchers are investigating new materials that reduce toxic byproducts while maintaining effective yields. Sustainable production pathways for graphene are essential to its acceptance and integration within industries where eco-standards are stringent.
Regulatory Considerations
Regulatory frameworks will play a significant role in shaping future graphene production trends. As graphene technologies advance, authorities must develop guidelines that ensure safety, quality, and environmental protection. Defining standards for graphene production is necessary, especially when integrated into consumer products or industrial applications.
Moreover, intellectual property rights and patent issues may emerge in this space, complicating collaboration across sectors. Foundations must be laid for regulatory environments that encourage innovation while protecting public interests. The balance between fostering breakthrough technologies and ensuring user safety will determine the pace at which graphene is adopted across applications.
"As graphene research progresses, the intersection of innovation, sustainability, and regulation will define the future of production."
In summary, future trends in graphene production hinge on innovative techniques, sustainability efforts, and regulatory frameworks. Each area not only addresses the challenges associated with current production but also anticipates the needs of tomorrow's applications. Through these concerted efforts, the full potential of graphene can emerge, leading to transformative changes in technology and industry.
Epilogue
In this concluding section, we emphasize the importance of the various insights and techniques surrounding graphene production. The exploration of this topic not only reveals the complexity inherent in synthesizing graphene but also highlights its significance in multiple domains. Understanding graphene’s production methods and their related challenges is crucial for both academic pursuits and industry applications.
Summary of Findings
This article has provided an in-depth look at the numerous techniques for producing graphene, detailing established methods like mechanical exfoliation and chemical vapor deposition as well as newer approaches such as liquid-phase synthesis. Each technique presents its own advantages and challenges that are critical for scaling applications. Key findings include:
- The mechanical exfoliation method is known for producing high-quality graphene, though at a limited scale.
- Chemical vapor deposition can achieve larger quantities, but quality control remains a concern.
- The reduction of graphene oxide offers a cost-effective, albeit less controlled, means of production.
The insights gathered underscore the dynamic nature of graphene research and the importance of continuous innovation. The interplay between different synthesis techniques and their respective applications reveals a path forward for effectively utilizing graphene's remarkable properties.
Implications for Future Research
Future research directions will likely focus on enhancing production rates while maintaining the quality of graphene. Some pivotal areas for investigation include:
- Innovative Synthesis Techniques: Exploration into novel synthesis methods may lead to breakthroughs in efficiency and application.
- Sustainability: As environmental concerns grow, developing greener synthesis processes will be paramount.
- Scalability Solutions: Addressing the challenges related to scalability will allow for broader commercial adoption of graphene.
Researchers are also encouraged to investigate the regulation and standardization of graphene production to ensure the safe and sustainable use of this material. Each advancement in this field carries the potential to revolutionize technologies across electronics, energy, and biomedical sectors, highlighting the profound implications of graphene on future innovations.
"Graphene’s unique properties make it a focal point for advancing numerous technologies ranging from ultrafast electronics to cutting-edge energy solutions."