Understanding Terahertz Signal Generators and Their Uses
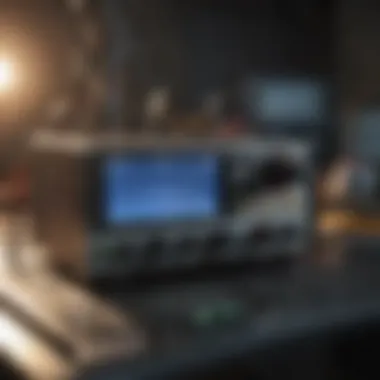
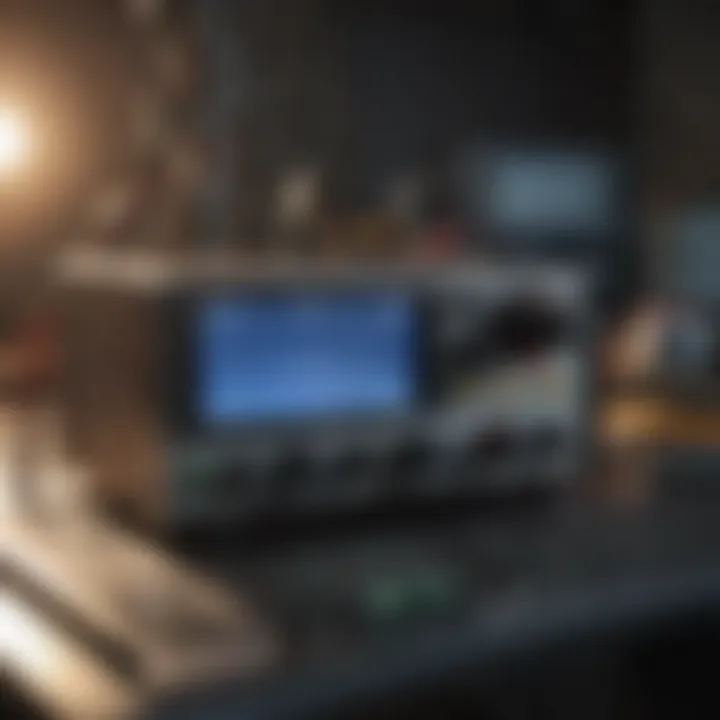
Intro
The terahertz frequency range, wedged between microwave and infrared radiation, has generated considerable interest in recent years. Exploring the potential of terahertz signal generators reveals a fascinating intersection of physics, engineering, and practical applications. With frequency ranges from about 0.1 to 10 THz, terahertz waves are capable of penetrating various materials, making them invaluable in fields such as material science, communications, and imaging.
An emerging area of research involving terahertz technology underscores its versatility. Its ability to provide non-destructive testing, high-resolution imaging, and secure communication solutions positions it as a game-changing tool in several scientific and industrial arenas. The quest for advancements in terahertz signal generation has propelled investigations into numerous principles and methodologies, paving the way for exciting breakthroughs. Through this deep dive, we will illuminate the mechanisms driving these technologies and assess their transformative potential across various disciplines.
Prelims to Terahertz Signal Generation
The exploration of terahertz signal generation stands at the crossroads of advanced technology and innovative research, marking a significant milestone in our scientific capabilities. Terahertz frequencies—ranging roughly from 0.1 to 10 THz—fall between microwave and infrared frequencies on the electromagnetic spectrum, holding unique properties that make them invaluable across diverse fields such as telecommunications, imaging, and material sciences. In this context, understanding how terahertz signals are generated is crucial for grasping their applications and implications.
Defining Terahertz Frequency
To comprehend the significance of terahertz frequency, it is essential to clarify its position in the electromagnetic spectrum. Terahertz frequencies occupy a domain that is often overlooked yet vitally important. Frequencies below 0.1 THz are typically classified as microwave, while those above 10 THz are regarded as infrared. This band is characterized by its ability to penetrate various materials, providing a unique advantage for sensing and diagnostic purposes. For instance,
- Non-destructive testing: Terahertz signals can pass through fabrics, plastics, and other materials, allowing for analysis without damaging the sample.
- Spectroscopy: By utilizing terahertz frequencies, researchers can identify chemical compounds, providing insights into material compositions with remarkable precision.
The growing body of research surrounding terahertz technology highlights its potential in numerous applications, thus making the understanding of terahertz frequency a fundamental aspect of modern physics and engineering.
Importance of Terahertz Signals
As we delve deeper into the significance of terahertz signals, it becomes clear that their value stretches far beyond just scientific curiosity. These signals are crucial in enhancing various technological solutions:
- Imaging technologies: This realm uses terahertz waves to produce detailed images of objects, which is especially beneficial in the medical field for imaging tissues and organs.
- Data transfer: With the advent of high-speed communication systems, terahertz frequencies offer the promise of greater bandwidth and improved data rates, paving the way for next-gen wireless technologies.
Furthermore, their ability to offer information-rich spectra allows researchers to glean insights that are not perceptible through other means. Analysts and researchers can leverage terahertz signals not only for basic research but also for practical applications ranging from security screening to quality control in manufacturing processes.
"Witnessing the potential of terahertz signals reshaping industries and sparking innovations is nothing short of extraordinary."
In essence, terahertz signal generation is more than just a technical endeavor; it's about exploring new frontiers that can merge scientific research and practical applications into one seamless entity, driving future innovations.
Throughout this article, we will examine the principles and applications of terahertz signal generators, revealing their transformative power and unique capabilities.
Fundamentals of Terahertz Signal Generators
Understanding the fundamentals of terahertz signal generators is crucial, as they embody the bridge between theoretical concepts and practical applications in various scientific fields. This section serves to unravel the core ideas behind these devices, ultimately illustrating how they facilitate advancements in areas like spectroscopy and imaging.
Firstly, it's essential to appreciate that terahertz signal generators create electromagnetic waves in the terahertz frequency range, which plays an integral role in studies related to molecular inspection, material characterization, and even biomedical applications. By providing insights into the principles of operation and different types of signal generators, we can highlight their unique capabilities and limitations, adding texture to our knowledge about this fascinating domain.
Basic Operating Principles
The basic operating principle of terahertz signal generators can be simplified into a two-step process: generation and manipulation. In layman's terms, these devices convert energy from one form to another, often starting from electrical signals or optical sources.
- Energy Conversion: The primary technique involves the conversion of electronic energy into terahertz radiation. This can be accomplished using various materials or specialized components, each with its operational quirks. For instance, when certain semiconductors are subjected to an external field, they emit terahertz waves as they relax back into equilibrium states.
- Wave Manipulation: After the signal is generated, the terahertz waves may need adjustments, like amplification or modulation, to enhance signal quality. This manipulation may utilize lenses, crystals, or even other electronic devices, ensuring the end output meets the application needs.
Through this mechanism, terahertz signal generators hold immense promise, especially due to their ability to probe materials without causing damage.
Types of Terahertz Signal Generators
Several types of terahertz signal generators have emerged in the field, each utilizing distinct mechanisms with unique benefits and challenges. Understanding these types can provide clarity into the options available for specific applications.
Optical Pumping Techniques
Optical pumping involves using strong laser beams to excite the atoms in a material, leading to the generation of terahertz signals. One key characteristic of this technique is its exceptional efficiency in generating high-frequency signals. The reason this method garners attention is its capacity to produce tunable frequencies, making it adaptable to varied research needs.
However, the main drawback lies in its complexity; the setup can be somewhat cumbersome and may require precise alignment. Nevertheless, for applications demanding high performance, this technique remains a preferred choice.
Nonlinear Optical Devices
Nonlinear optical devices exploit the properties of certain materials to produce terahertz waves through, for example, frequency mixing or difference frequency generation. A notable aspect is their capability for generating terahertz signals at relatively low input power, which makes them popular when energy efficiency is a concern.
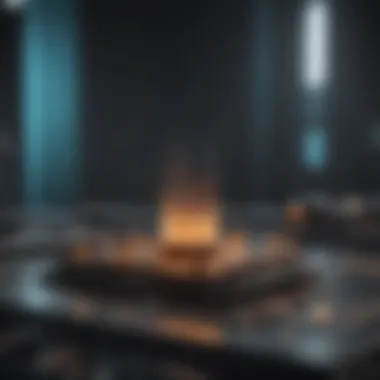
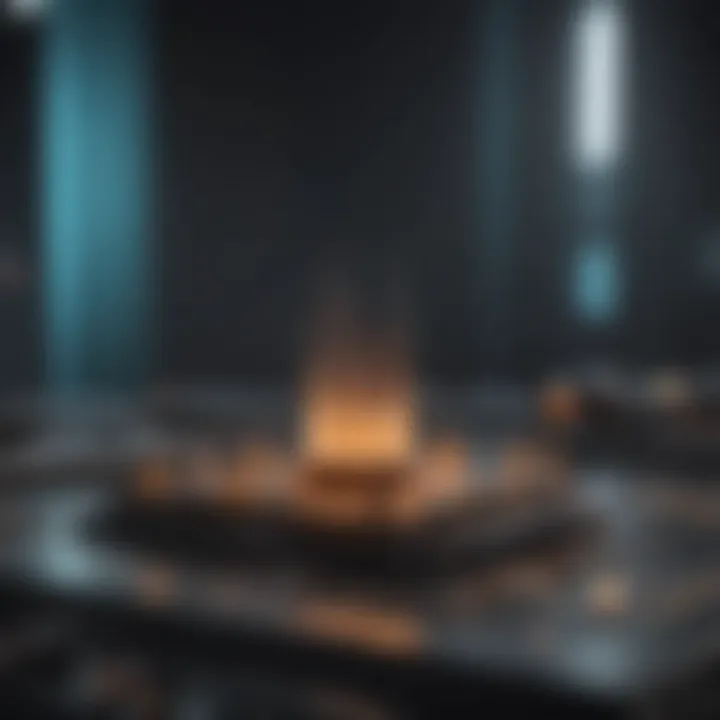
Also, these devices can produce signals in bulk, making them suitable for mass market applications. The downside, however, is that nonlinear effects can be sensitive to environmental conditions, which may complicate consistent output. That being said, their inherent efficiency often compensates for these hurdles in many scenarios.
Quantum Cascade Lasers
Quantum cascade lasers (QCLs) represent a significant leap in terahertz technology, offering a compact solution for signal generation. The fundamental principle driving a QCL is the intersubband transition between electron energy levels in a semiconductor material. Their key characteristic is the ability to provide continuous-wave operation and tunability over a broad range of frequencies.
The adoption of QCLs has surged due to their high output power and relatively small size, rendering them ideal for portable applications. However, the cost of fabrication can be higher, which poses a financial barrier. Even so, their advantages often overshadow the initial investment, merging performance with practicality in exciting ways.
Overall, grasping these fundamentals enhances appreciation for terahertz signal generators and their transformative potential across multiple domains. Understanding the specific operating principles and types sets the stage for further exploration into their materials, challenges, advancements, and eventual applications.
Materials Used in Terahertz Signal Generation
Understanding the materials utilized in terahertz signal generation is crucial for grasping the operational efficiency and versatility of these devices. Each category of material not only contributes uniquely to the generation process but also influences the overall performance, from the speed of signal generation to the clarity of the output. As technology evolves, the demands and applications of terahertz generators increase, necessitating the exploration of various materials that enhance their functionality.
Semiconductor Materials
Semiconductor materials are a cornerstone in the field of terahertz signal generation. They possess distinctive electronic properties that make them ideal for high-frequency applications. Key examples include Gallium Arsenide and Indium Phosphide. These materials are often used in quantum cascade lasers and other devices to facilitate the generation of terahertz signals through efficient electron transitions.
The ability of semiconductor materials to be doped significantly affects their conductivity and ultimately their efficiency. When impurities are added to these semiconductors, they can be tuned to optimize the energy levels where electrons and holes exist. As a result, the construction of terahertz devices can leverage specific semiconductor traits. Moreover, their compact size and potential for integration into hybrid systems make them valuable in practical applications.
Dielectric Materials
Dielectric materials might seem unassuming at first glance, but they serve an essential role in signal generation. These materials are characterized by their ability to store electrical energy when an electric field is applied, making them crucial for creating resonant cavities within terahertz devices. Common dielectrics include polymers like Polytetrafluoroethylene (PTFE) and ceramics such as Barium Titanate.
When used in conjunction with semiconductor materials, dielectric materials can enhance the performance of terahertz generators. They help shape the emitted signals by acting as a guide, effectively confining the electromagnetic waves within a specific region. This confinement often leads to improvements in signal strength and resolution, which are critical in applications such as spectroscopy and imaging.
Metamaterials and Their Role
Metamaterials, often described as man-made materials that exhibit properties not typically found in nature, have sparked significant interest in the realm of terahertz technology. These materials can be engineered to affect electromagnetic waves in unconventional ways, which can result in unusual refractive properties or even negative indices of refraction.
In the context of terahertz signal generation, metamaterials can enhance the capability to manipulate terahertz waves, enabling the development of more compact and efficient devices. Their applications range from creating superlenses that surpass traditional diffraction limits to improving the sensitivity of terahertz detectors.
"The combination of metamaterials with traditional materials opens a new frontier in terahertz technology, offering prospects for next-generation applications!"
In summary, the materials used in terahertz signal generation — from semiconductors to metamaterials — play a vital role in shaping the future of this technology. By understanding the unique properties and potential applications of each material, researchers and developers can push the boundaries of what is possible in the terahertz domain. This knowledge not only fuels innovation but also paves the way for advancements in spectroscopy, imaging, and communication technologies.
Challenges in Terahertz Signal Generation
The landscape of terahertz signal generation is illuminated by its vast applications and potential within fields ranging from medical imaging to advanced communications. However, beneath the surface, several challenges lurk that can impede progress and innovation. Addressing these hurdles is essential for researchers and engineers seeking to refine the technology further and unlock its full capabilities. Understanding the limitations can pave the way for novel solutions and innovations, thus enhancing the overall usage of terahertz technology.
Signal Loss and Attenuation
One of the prevalent challenges faced in terahertz signal generation is signal loss and attenuation. When terahertz waves traverse through various materials—be it in the atmosphere or within solid substrates—there are numerous factors influencing how much of the signal gets absorbed or scattered.
For instance, water vapor can significantly attenuate terahertz signals, particularly in humid conditions. This phenomenon poses limitations for applications that rely on long-range transmission and precise measurements. The intrinsic nature of terahertz frequencies, residing between microwave and infrared ranges, often results in higher susceptibility to atmospheric disturbances.
To combat these losses, researchers are exploring different methodologies to enhance transmission efficiency. Several avenues include:
- Utilization of metamaterials: These engineered materials can manipulate terahertz waves, aiding in reducing losses and enhancing signal strength.
- Shielding and controlling environmental conditions: Implementing technologies that minimize exposure to moisture or interference, which can increase the reliability of terahertz applications.
Technological Limitations
Despite the exciting advancements in terahertz technology, several technological limitations present a stumping reality. The generation of terahertz signals often demands specialized components that can be both intricate and expensive. Moreover, the speed and efficiency of current signal generators might not meet the demands of real-time applications, stalling their integration into mainstream technologies.
The complexity associated with designing and fabricating terahertz components leads to:
- High power requirements: Many terahertz signal generators require significant power to operate, making them less feasible for portable applications.
- Limited bandwidth: Devices may struggle to cover a wide range of frequencies effectively, hindering the quality of spectral data collected.
Cost of Production
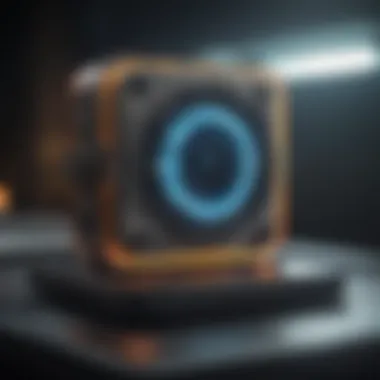
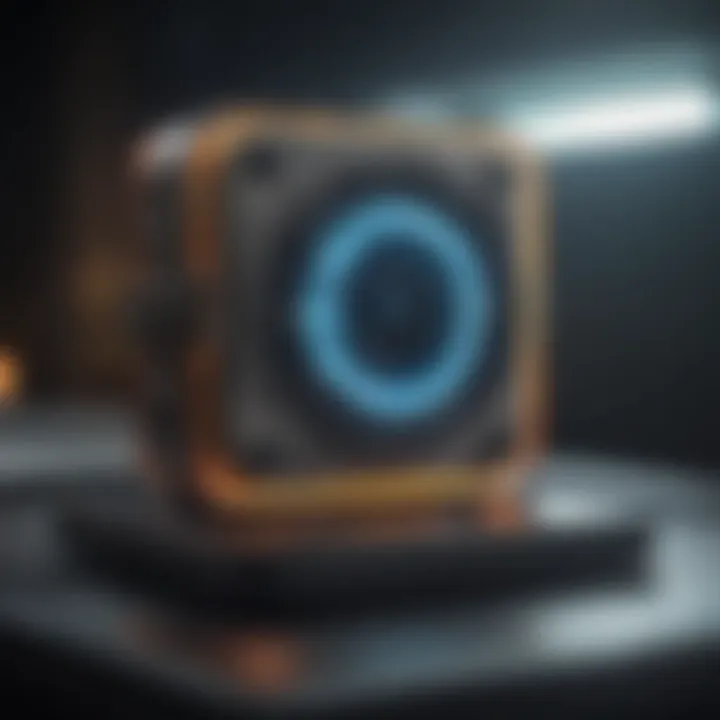
While interest in terahertz signal generators has grown, the cost of production remains a considerable hurdle. The intricate materials and precision engineering necessary to produce functional components contribute significantly to overall costs.
This financial burden can restrict wider adoption in commercial applications and push potential innovators to consider alternative technologies. Considerations regarding safety standards, certifications, and ongoing research investments inflate the costs further.
Some of the factors leading to high production costs include:
- Sophisticated manufacturing processes: High precision manufacturing techniques often require advanced technology, further driving expenses.
- Research and development investments: The initial phase of developing new terahertz technologies demands significant capital investments, potentially limiting access to smaller organizations or startups.
As the field evolves, a concerted effort to mitigate these challenges can reshape the future landscape of terahertz signal generation, fostering wider accessibility and heightened capabilities.
Advancements in Terahertz Technology
The field of terahertz technology has seen remarkable progress in recent years, with innovations pushing the boundaries of what these devices can achieve. These advancements are not just about increasing performance; they focus on enhancing the versatility of terahertz signal generators. With growing applications across diverse sectors, understanding these improvements is crucial for researchers and industry professionals alike.
Recent Innovations
Recent innovations in terahertz signal generators span several dimensions, from materials development to enhanced design techniques. One significant innovation has been the development of more efficient semiconductor materials. This is vital because higher efficiency leads to improved signal output and reduced energy consumption, ultimately making devices more sustainable. The application of graphene has emerged as a game-changer due to its exceptional electronic properties. By utilizing graphene, researchers have been able to generate terahertz signals with much lower power requirements than traditional materials.
Moreover, advancements in quantum cascade lasers have also propelled the effectiveness of terahertz generation. These lasers are capable of producing continuous wave terahertz signals, which is crucial for practical applications such as imaging and communications. The latest designs feature nano-structured semiconductor layers that allow for fine-tuning emissions to specific frequencies, thereby expanding the range of potential uses.
In addition to these hard innovations, there is also a trend toward integrating terahertz technology with existing electronic and optical systems. For instance, novel configurations allow terahertz devices to work seamlessly with photonic systems. As a result, this leads to sophisticated devices that combine the advantages of both worlds—terahertz and optical technologies—offering greater functionality and versatility.
Integration with Optical Technologies
The integration of terahertz signal generators with optical technologies holds substantial promise for various applications. This synergy allows for the merging of high-frequency terahertz waves with optical signals, facilitating new capabilities. The hybrid systems can enable faster data transmission rates and enhance the overall performance of communication networks.
One striking example is the use of terahertz devices in conjunction with fiber optic networks. By employing terahertz waves, the data capacity can be significantly increased compared to traditional methods. This integration opens new avenues in data management and telecommunications.
Furthermore, terahertz imaging has benefited from these advancements as well. By combining terahertz technology with established optical techniques, imaging systems can achieve finer resolution while maintaining speed. This is particularly promising for biomedical applications where detailed imaging of biological tissues and materials can lead to breakthroughs in diagnostics.
"Innovations in terahertz technology are not just about enhancing one aspect; they're about creating systems that work together seamlessly to achieve remarkable results across sectors."
Moreover, as researchers explore more innovative integration methods, potential stays high for improvements in areas like safety inspections, quality control in manufacturing, and even environmental monitoring. The blending of these technologies paves the way for smarter systems that are more robust and responsive to the challenges faced in various fields.
In summary, the advancements in terahertz technology, particularly the innovations and integrations with optical systems, highlight a significant trajectory for future developments. This lays the groundwork for leveraging terahertz signals to achieve unprecedented levels of efficiency and functionality.
Applications of Terahertz Signal Generators
Terahertz signal generators are becoming increasingly important across various scientific and engineering fields. Their capacity to produce signals in the terahertz range opens doors to applications that blend high-speed communication and precise analysis. One core advantage of terahertz technology lies in its ability to penetrate materials without causing damage, making it exceptionally valuable in spectroscopy, imaging, and even telecommunications. As we explore these applications, it’s quite clear that the versatility of terahertz signals enhances our capacity to investigate and innovate across multiple domains.
Terahertz Spectroscopy
Chemical Composition Analysis
The realm of chemical composition analysis is where terahertz signal generators shine. They provide a unique way to identify substances based on their molecular vibrations. What’s particularly striking about this method is how it can analyze materials in their natural state, minimizing sample preparation. Researchers can use terahertz spectroscopy for real-time monitoring, allowing for prompt identification of chemical changes.
The key characteristic of this analysis rests in its non-invasive nature. For many industries, especially pharmaceuticals and food production, having the ability to analyze without altering the sample can be a game changer. Another unique feature is the provision of high spectral resolution, which is essential for distinguishing between closely related compounds. However, one downside is that the acquisition of data can require significant time, especially for complex mixtures, which at times can be a drawback in fast-paced environments.
Material Characterization
When it comes to material characterization, terahertz signal generators again step to the forefront. They enable the detailed study of various materials, from semiconductors to plastics, helping researchers evaluate properties such as thickness, density, and uniformity. This characterization is critical for quality control in manufacturing processes, ensuring that products meet stringent specifications.
A major highlight of terahertz material characterization is its ability to provide bulk sensing. This implies that analysts can gather information about the entire volume of a sample rather than a surface scan. Notably, this can lead to more reliable results when determining material properties. However, the disadvantage is that it may not be as effective in screening very thin materials or coatings, which are sometimes essential in specific applications.
Imaging Techniques
Biomedical Imaging
In the field of biomedical imaging, terahertz signal generators are making waves. By providing images of tissue structures without the need for dyes or complicated preparations, they present a non-invasive approach that can significantly improve diagnostics. Terahertz imaging addresses various medical imaging needs, allowing for earlier detection of anomalies in tissue characterization.
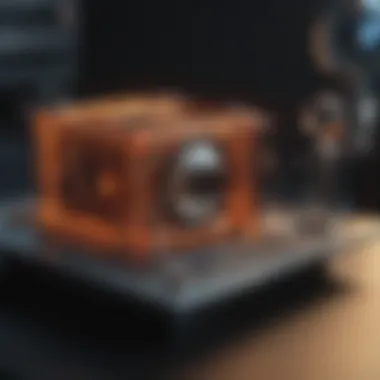
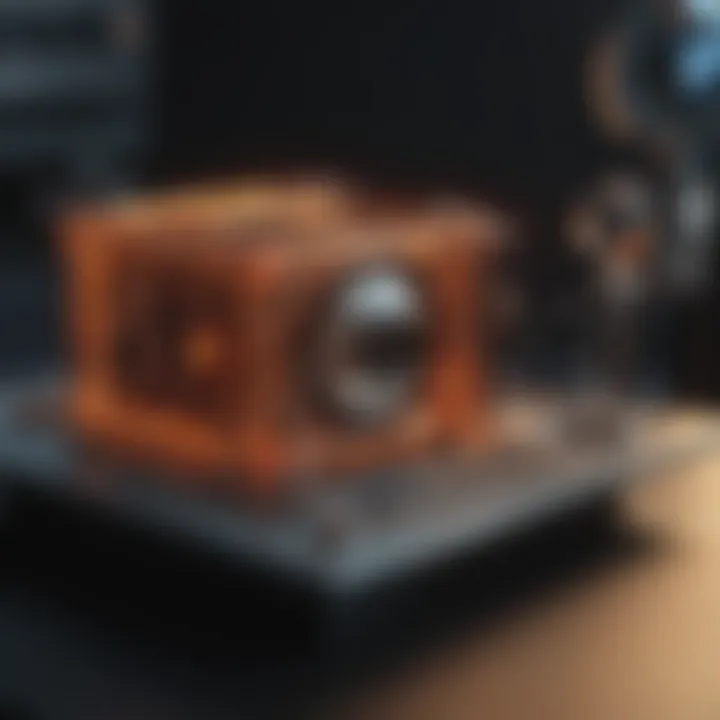
The key characteristic of biomedical imaging using terahertz technology is its capability to visualize biological tissues in a way that is distinct from traditional imaging techniques. For instance, terahertz waves can differentiate between healthy and unhealthy tissues based on their hydration levels. This unique feature can lead to breakthroughs in detecting tumors and other pathological changes. Still, some limitations exist, such as the low penetration depth in denser tissues, which can hinder its effectiveness in deeper examinations.
Industrial Inspection
Terahertz signal generators also have a strong foothold in industrial inspection. They are particularly effective in checking the integrity of materials, even those that are layered or coated. This application is vital in fields such as aerospace and automotive manufacturing, where ensuring that components meet specific safety standards is non-negotiable.
A notable characteristic of industrial inspection via terahertz technology is its capacity for real-time monitoring during production processes. This can drastically reduce the need for extensive post-production testing. The unique feature here is also the capability to identify hidden defects that typical optical methods might miss. However, one downside can be the higher initial setup costs, which may deter smaller enterprises from adopting this cutting-edge technology.
Communication Technologies
Terahertz Wireless Communication
As we step into the realm of communication technologies, terahertz wireless communication presents a revolution. The ability to transmit data at incredibly high rates means potential advancements that could reshape entire industries. Terahertz signals can carry large quantities of data over short distances, making them suitable for next-gen communication systems.
One key characteristic is the extensive bandwidth that terahertz communication offers, allowing simultaneous data transmission that outstrips current technologies. Another significant advantage is that terahertz waves can still function in an unlicensed frequency range, making them an attractive option for new communications infrastructure. However, environmental factors such as moisture can dampen signal clarity, which poses a challenge for widespread adoption.
Data Transmission Enhancements
Data transmission enhancements utilizing terahertz signals could pave the way for even faster and more reliable communications. By integrating terahertz technology into existing systems, it’s possible to improve the data rates significantly, facilitating faster downloads and more efficient connectivity.
This application stands out primarily because of its potential to optimize existing networks. Terahertz signals can be layered over current technologies to increase throughput without having to overhaul entire systems. Yet, challenges remain, such as developing suitable materials for effective transmission, which can create hurdles in making these enhancements commonplace.
Future Prospects of Terahertz Technology
The rapid development of terahertz technology opens vast opportunities, hinting at a future where terahertz applications are seamlessly integrated into various sectors. As these technologies advance, they promise to revolutionize fields ranging from telecommunications to medicine. This section highlights some of these directions while focusing on why this area deserves further exploration and investment.
The significance of terahertz technology is evident not only in its immediate applications but also in how it challenges existing paradigms. With its unique ability to penetrate various materials and yield rich information about their properties without causing harm, it positions itself as a game-changer in multiple disciplines.
Potential Research Directions
Research in the terahertz field is buzzing with potential. Here are some avenues worth exploring:
- Enhanced Imaging Techniques: Future research could iterate on terahertz imaging capabilities, perhaps improving resolution or depth of penetration. This can be vital in sectors like security scanning and medical diagnosis.
- Biomolecular Applications: Delving deeper into how terahertz radiation interacts with biological molecules opens doors for advancements in early disease detection and drug development.
- Integration with Artificial Intelligence: Merging AI with terahertz technology may yield more efficient data analysis and interpretation, potentially accelerating product development in various markets.
Research in these areas can push boundaries and provide new insights, addressing some unresolved challenges while simultaneously fostering innovation.
Industry Trends and Expectations
As the industry continues to adapt to global shifts, several trends are shaping the future of terahertz technology:
- Cost Reduction in Technology Manufacturing: With advancements in fabrication techniques, the expenses associated with producing terahertz devices are slowly decreasing, making them more accessible for widespread use.
- Miniaturization and Portability: There’s a strong push for smaller, more portable terahertz devices. This feedback loop of innovation could see these technologies integrated into everyday gadgets like smartphones or handheld scanning devices.
- Telecommunications Advancements: Companies are exploring terahertz communications as a means to enhance wireless networks, potentially setting the stage for new data transmission standards that facilitate faster and more reliable connectivity.
"The future of terahertz technology holds promise not just in refining existing applications but also in discovering entirely new arenas of possibility."
With continuous evolution in these areas, stakeholders should watch closely. The advances that come from these efforts can reshape markets and elevate standards in numerous fields.
Closure and Final Thoughts
The exploration of terahertz signal generators offers a window into a rapidly advancing realm of technology that promises to reshape numerous fields—from communications to material science. This discussion has peered into the mechanics behind these devices, laying the groundwork for understanding their significance in today’s technological landscape.
In summary, terahertz signal generators harness unique principles of physics and materials science to produce signals in an often-overlooked frequency range. The diverse types of generators—be it through optical pumping techniques, nonlinear optical devices, or quantum cascade lasers—highlight the versatility and adaptability of this technology.
"The hallmark of advancement in terahertz technology is not merely in the tools themselves, but in their applications that challenge the boundaries of current knowledge."
These valves of technology open new doors. The contributions made in spectroscopy, imaging techniques, and communication technologies illustrate the potential for enhancing existing systems and developing new methodologies.
Summary of Key Insights
- Technological Foundations: Understanding the basic operating principles of terahertz signal generators is pivotal to grasp how they function in real-world applications.
- Materials Matter: The choice of materials significantly affects signal integrity and generation efficiency; thus, exploring innovative materials remains a central focus.
- Challenges Present Opportunities: Navigating issues such as signal loss and the cost of production is crucial for realizing the full potential of terahertz technology.
- Applications are Vast and Growing: Whether in biomedical imaging, industrial inspection, or improving wireless communication, the applicability of terahertz signals extends beyond traditional boundaries.
The Importance of Continued Research
The drive for innovation in terahertz signal generation is not static; it thrives on curiosity and the continuous pursuit of knowledge. Continued research is fundamental for several reasons:
- Expanding Knowledge: As researchers delve deeper into terahertz technologies, they unlock new methodologies that could revolutionize current applications.
- Material Advancements: Ongoing studies into novel semiconductor and dielectric materials can lead to improved efficiencies and capabilities.
- Adapting to Real-World Needs: With industries constantly evolving, research in terahertz technology allows for the adaptation of solutions to meet emergent challenges.
- Collaboration is Key: Interdisciplinary research encourages collaboration among scientists, engineers, and industry professionals, creating a nexus of knowledge that can pave the way for groundbreaking advancements.
Ultimately, the journey through the terahertz spectrum is just beginning. By unraveling its nuances and fostering continuous exploration, we can expect a ripple effect across various sectors, ensuring that the future of technology remains bright and promising.