Terahertz Sources: An In-Depth Examination of Innovations
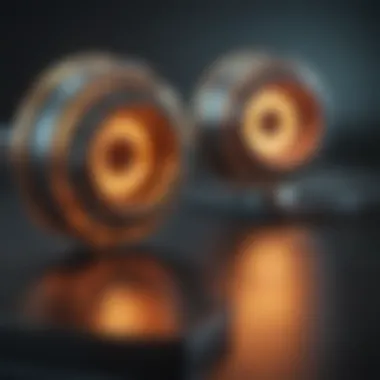
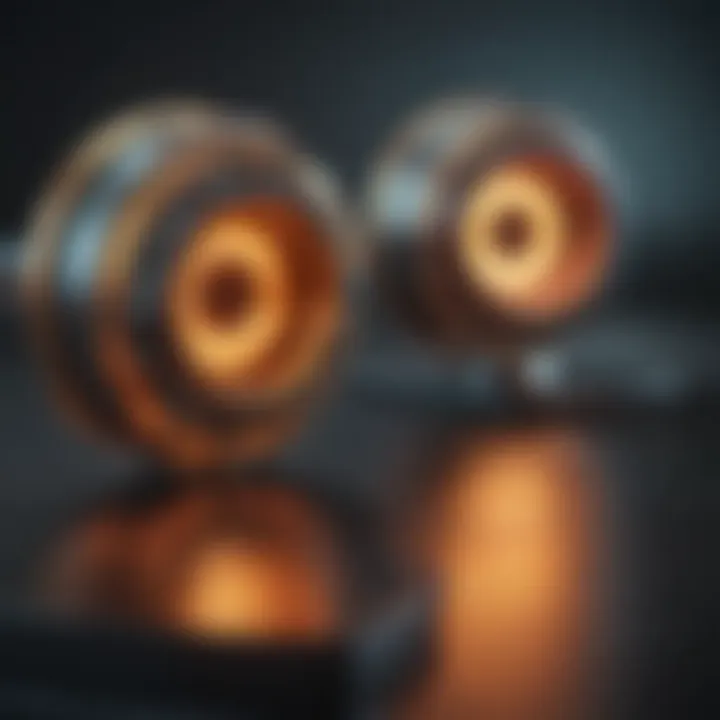
Research Background
Terahertz (THz) radiation occupies a unique position on the electromagnetic spectrum, bridging the gap between microwaves and infrared light. The scientific problem addressed by this area of study lies in the limited availability of reliable sources and detectors for terahertz waves. This limitation has hindered significant advancements in applications spanning telecommunications, security, and imaging technologies. Understanding the potential of terahertz sources can lead to transformative changes across various fields.
Historically, the exploration of terahertz radiation began in the late 20th century, coinciding with advancements in photonic and electronic technologies. Early studies primarily focused on developing terahertz time-domain spectroscopy and its capabilities in material characterization. Subsequently, researchers have shifted their attention towards creating efficient terahertz sources. This is essential for both fundamental research and practical applications.
The combination of various materials and innovative population mechanisms has propelled this research forward. Methods such as photoconductive antennas, quantum cascade lasers, and semiconductor devices have emerged as critical contributors to the development of terahertz sources. In the past decade, notable progress has been made, yet challenges persist, such as efficiency and operational range improvements.
Findings and Discussion
Current research indicates that terahertz sources can be classified into several categories based on their mechanisms and output properties. Notable types include:
- Photoconductive antennas - these utilize short laser pulses to generate terahertz radiation via the movement of charge carriers in semiconductor materials.
- Quantum cascade lasers - these devices generate terahertz radiation through a series of electron transitions in tailored semiconductor structures, providing highly controlled and coherent output.
- Dielectric resonators - these exploit resonances in dielectric materials to produce terahertz radiation effectively.
Key results from recent studies highlight that terahertz radiation has potential applications in diverse areas. It is being explored in future wireless communication systems, which could exceed current data rates significantly. Terahertz imaging holds promise for non-invasive security screening and biomedical applications, offering insights into structural anomalies without physical contact.
In interpreting these findings, one must consider the ongoing challenge of generating terahertz waves at room temperature and efficiently. As technology advances, the efficiency of sources will likely improve, enabling broader suitability in practical applications.
"The development and optimization of terahertz sources will be essential for truly realizing their transformative potential in industry and research."
Ultimately, understanding terahertz sources not only contributes to solidifying scientific foundations but also illuminates pathways for innovation. By staying aware of advancements in material science, device engineering, and photonics, the implications of terahertz radiation can be better understood, opening new avenues in technology.
Preface to Terahertz Sources
Understanding terahertz sources is essential in todayβs advancing technological landscape. Terahertz radiation, which falls between microwave and infrared radiation on the electromagnetic spectrum, presents unique opportunities across various fields. As the capabilities of terahertz technologies grow, so does their significance in applications ranging from wireless communications to medical imaging.
The advent of terahertz technology is an evolving narrative in scientific research. The ability to generate and manipulate terahertz radiation has implications for improving communication systems, providing deeper insights into material properties, and allowing for non-destructive testing. This exploration of terahertz sources will analyze the different types of sources, their generation mechanisms, and their practical applications. This narrative context is not only vital for students and researchers eager to uncover new technological potentials but also for professionals who may seek innovative solutions in their respective fields.
Definition of Terahertz Radiation
Terahertz radiation refers to electromagnetic waves with frequencies ranging from 0.1 to 10 THz. This range corresponds to wavelengths between 3 millimeters and 30 micrometers. The unique position of terahertz radiation within the spectrum allows it to penetrate various materials. It can pass through fabrics, plastics, and even some biological tissues without causing harm. This property makes terahertz radiation highly suitable for applications that require non-invasive probing and imaging.
Due to its ability to interact with matter at a molecular level, terahertz radiation serves as a valuable tool in spectroscopy. It enables researchers to identify and characterize different materials based on their spectral fingerprints. This has broad implications for several industries, such as pharmaceutical analysis, chemical detection, and quality control in manufacturing.
Historical Context
The exploration of terahertz radiation began in earnest during the late 20th century. Initial developments in the field were primarily linked to advancements in electronics and photonics. Early terahertz sources relied on simple techniques such as photoconductive switches and optical rectification. The discovery of quantum cascade lasers in the 1990s marked a significant breakthrough, enabling scientists to produce terahertz radiation more efficiently and controllably.
As research progressed, applications for terahertz technology began to emerge. Interest surged in the early 2000s when researchers discovered the potential for terahertz systems in areas like telecommunications and imaging. Since then, investment and research in terahertz technologies have increased, paving the way for more sophisticated sources and applications.
Terahertz technology stands at the forefront of research, combining innovation across various scientific disciplines to explore new realms of possibility in technology and industry.
As the field continues to evolve, the significance of terahertz sources becomes even more pronounced. Overall, understanding the historical context enriches the comprehension of current advancements and future directions in terahertz research.
Types of Terahertz Sources
Understanding the different types of terahertz sources is vital for grasping their applications and functions in modern technology. These sources play a foundational role in generating terahertz radiation used across various fields including communication, imaging, and material science. Recognizing the various categories helps researchers and professionals make informed choices when selecting the appropriate source for specific tasks.
Solid-State Sources
Solid-state sources are a prominent category in terahertz technologies. These sources utilize semiconductor materials to produce terahertz radiation. The primary benefits of solid-state sources include their compactness and the ability to integrate them into existing systems easily. Applications range from spectroscopy to imaging, providing flexibility across industries. The development of materials such as gallium arsenide has led to significant advances in these sources.
In many cases, solid-state sources offer high reliability and comparatively lower costs than other types of terahertz sources. However, their output power may not match that of bulkier sources. This limitation can impact their effectiveness in high-demand scenarios.
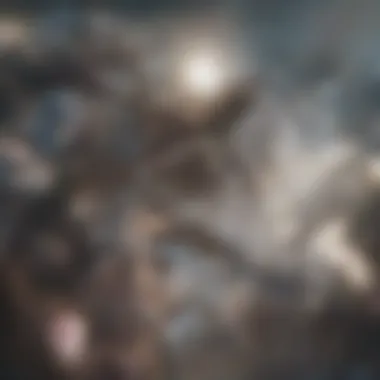
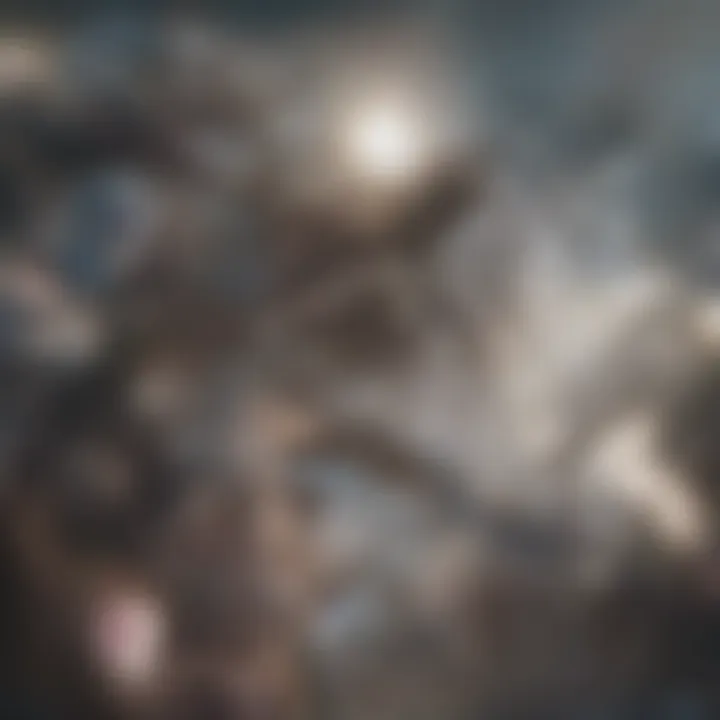
Quantum Cascade Lasers
Quantum cascade lasers (QCLs) represent a breakthrough in terahertz source technology. They generate terahertz radiation through electronic transitions within quantum wells. The ability to finely tune the output wavelength makes QCLs particularly valuable in spectroscopy and sensing applications. This specificity is beneficial for identifying chemical compositions effectively.
Furthermore, QCLs can operate at room temperature, enhancing their usability in various environments. Despite their advantages, the complexity of their fabrication can lead to increased costs. This makes them more suitable for specialized applications rather than mass-market solutions.
Photoconductive Antenna Sources
Photoconductive antenna sources leverage ultrafast laser pulses to create terahertz radiation. When a laser pulse hits the antenna structure, it generates a photocurrent that results in terahertz emission. This method allows for the generation of very short pulses, which is crucial for time-resolved measurements in materials science.
The main advantages of photoconductive sources are their ability to produce broadband terahertz signals and their adaptability for various scientific applications. However, the requirement for continuous laser excitation can pose operational challenges. These sources may not be suitable for all environments, particularly where portability is essential.
Optical Rectification Sources
Optical rectification sources use nonlinear optical processes to generate terahertz radiation. By interacting with intense laser fields, these sources can convert optical frequency signals into terahertz frequencies. This technique is valued for its capability to generate high-power terahertz waves, which find applications in material characterization and imaging systems.
However, optical rectification often requires precise alignment and sophisticated optical setups, which can complicate their use in practical applications. As research continues, innovations in this area may address some of these challenges, potentially broadening the usability of optical rectification sources.
"Innovations in terahertz source technology redefine how we approach imaging and material sciences, extending our capabilities far beyond current paradigms."
Overall, understanding the specific types of terahertz sources allows for informed decision-making in research and industry settings. Each method has its unique strengths and limitations, reflecting the diverse needs across various fields. As advancements continue, the importance of these technologies will only grow.
Mechanisms of Terahertz Generation
Understanding the mechanisms of terahertz generation is crucial in the broader context of this article. These mechanisms are foundational as they dictate how terahertz radiation is produced, influencing its efficiency, power, and overall utility in various applications. The terahertz range, which lies between microwave and infrared frequencies, presents unique challenges and opportunities. Grasping these mechanisms aids in optimizing terahertz sources and developing new technologies.
Free Electron Lasers
Free Electron Lasers (FELs) are a notable approach to generating terahertz radiation. These lasers utilize high-speed electrons traveling through a magnetic field. As these electrons move, they oscillate and emit radiation across a range of frequencies, including the terahertz spectrum. This method is particularly effective because it allows for tunable output, enabling researchers to select specific terahertz frequencies.
FELs have several advantages:
- High output power
- Wide tunability
- Ability to produce ultra-short pulsed radiation
These features make them a powerful tool in scientific research, particularly for applications requiring precise measurements or high peak power.
Phase-Locked Techniques
Phase-locked techniques represent another approach to terahertz generation. In essence, these methods involve using two or more lasers, which are synchronized in phase. This synchronization enables the coherent mixing of laser beams to generate terahertz waves through a process known as difference-frequency generation. This technique is valuable in that it allows for a stable and controlled output of terahertz radiation.
One major advantage of phase-locked techniques is:
- Enhanced beam quality
This aspect is crucial when considering applications in high-resolution imaging or spectroscopy. The coherence and control inherent in this method facilitate advanced studies in various fields, including material science and biological imaging.
Nonlinear Optical Processes
Nonlinear optical processes also contribute to terahertz generation, primarily through the interaction of intense laser light with nonlinear media. When an intense laser pulse interacts with a nonlinear medium, it can induce polarization changes that lead to the generation of terahertz radiation.
Such processes include:
- Optical rectification: In this method, a strong electric field from a laser pulse causes the medium's charge carriers to move, generating terahertz radiation.
- Difference frequency generation: This involves two frequencies combining in a nonlinear medium, producing new frequency components in the terahertz range.
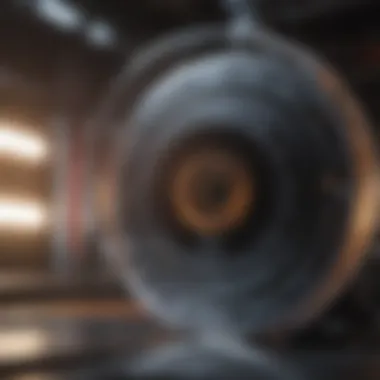
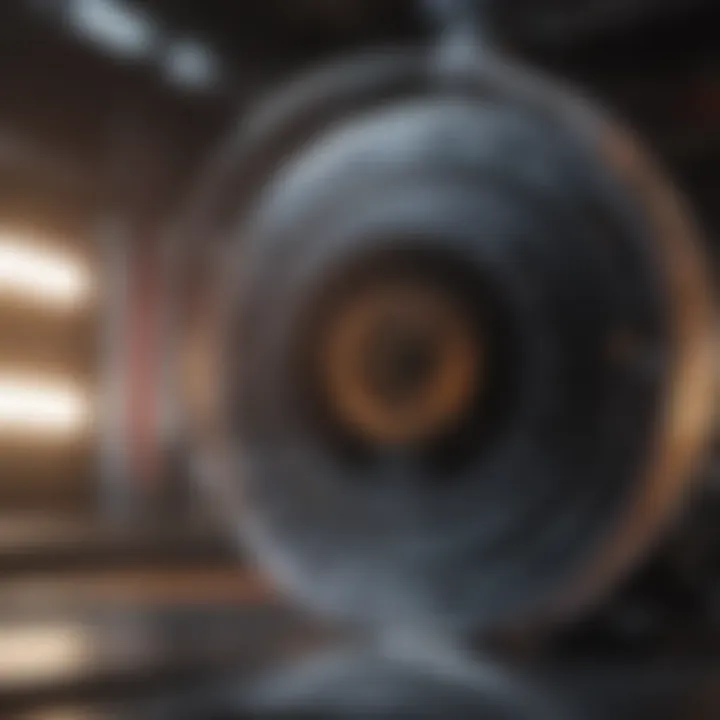
Nonlinear processes are particularly advantageous because they can generate THz waves at room temperature, allowing for practical applications without the need for complex cooling systems.
"The exploration of these mechanisms not only enhances our understanding of terahertz sources but also opens doors for innovative applications across various domains."
Applications of Terahertz Technology
The field of terahertz technology is advancing rapidly, revealing numerous applications that have profound implications across various industries. These applications enhance our understanding and capabilities in medicine, communications, and materials science. The versatility of terahertz radiation facilitates new methods for analysis and innovation, making it an essential subject within this article.
Medical Imaging
Terahertz technology offers exciting potentials in medical imaging. The ability of terahertz waves to penetrate biological tissues without causing harmful effects allows for non-invasive imaging techniques. This is crucial in detecting conditions such as skin cancer and other abnormalities.
An advantage of terahertz imaging is its ability to differentiate between healthy and diseased tissue based on the unique absorption characteristics at terahertz frequencies.
- Non-ionizing Nature: Terahertz radiation is non-ionizing, meaning it does not pose the same risks associated with X-rays. This feature allows for repeated examinations without significant risk to patients.
- Detailed Tissue Information: Terahertz systems provide real-time imaging, offering structural and chemical information that supports accurate diagnosis.
Overall, integrating terahertz imaging into clinical practice can lead to earlier detection and improved outcomes.
Secure Communications
Secure communications is another vital area benefiting from terahertz technology. The terahertz frequency band offers a vast bandwidth, significantly higher than that of microwave or millimeter-wave technologies. This characteristic is essential for transmitting data securely and quickly.
Applications in secure communications include:
- High Data Rates: Terahertz waves can support data transmission rates that are orders of magnitude faster compared to traditional methods, facilitating high-speed networks.
- Resilience to Eavesdropping: The unique propagation characteristics of terahertz waves make them less susceptible to interception, enhancing security.
Thus, as global reliance on data security grows, terahertz technology may emerge as a cornerstone for secure communication systems.
Material Characterization
The capability of terahertz radiation to probe materials on a molecular level is invaluable for material characterization. This technique enables the analysis of material properties, both in research and industrial applications.
Key benefits include:
- Non-Destructive Testing: Terahertz methods allow for the thorough analysis of materials without causing damage, making it ideal for quality control in manufacturing.
- Identification of Materials: By examining the spectral fingerprints that terahertz waves provide, researchers can identify and classify materials accurately and efficiently.
In material science, terahertz technology thus serves as a powerful tool that supports innovation and ensures material integrity.
"The integration of terahertz technology in these varied applications signifies a turning point, influencing not only how we conduct analysis but also improving the quality of life through enhanced technology."
In summary, the applications of terahertz technology are extensive and promising. Ongoing research and development in this field are likely to unlock further capabilities and extend its impact across multiple disciplines.
Challenges in Terahertz Source Development
The development of terahertz sources faces several challenges that affect not only the efficiency of production but also the integration into existing technologies. Understanding these challenges is crucial for advancing the field, ensuring that innovations can be applied effectively across various sectors. Here, I will discuss three major challenges: cost of production, efficiency and output power, and the integration with existing systems.
Cost of Production
The financial aspect of producing terahertz sources is a significant barrier. High-quality terahertz devices often require specialized materials and sophisticated manufacturing processes. For instance, the components used in quantum cascade lasers or photoconductive antennas are not only expensive but also need advanced fabrication techniques. The cost also increases with the demand for precision in engineering. Manufacturers are challenged to reduce costs without sacrificing performance.
Investing in research that leads to cheaper materials or simplified fabrication methods is essential. Collaboration between industry and academia can accelerate cost-effective solutions. Funding initiatives could focus on developing lower-cost alternatives while maintaining performance standards. Overall, if production becomes more economical, it might trigger broader adoption of terahertz technologies across different fields.
Efficiency and Output Power
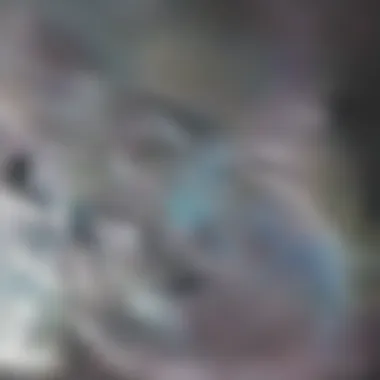
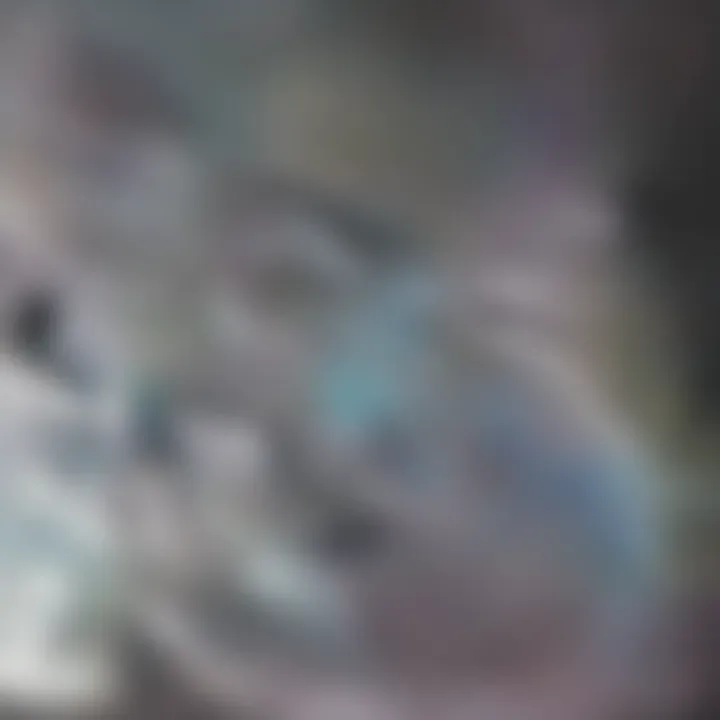
Efficiency of terahertz sources remains another hurdle. Devices like free electron lasers have significant power output but may require complex setups that limit their practical applications. Conversely, less powerful options might not suffice in demanding applications, leading to inefficiency in energy use.
Improving the efficiency involves engineering at a microscopic level to optimize how terahertz radiation is generated and emitted. Research into nonlinear optics processes has shown promise, but scaling these methods to produce higher output powers consistently is difficult. Further studies are needed to find the right balance between efficiency and the quality of the output. This is critical since industrial use often demands already reliable and robust systems.
Integration with Existing Systems
Integrating new terahertz sources into existing technological frameworks is also a challenge. Many established systems are not designed to work with terahertz technologies, which could render them ineffective. This can lead to higher costs in terms of retrofitting or even replacing existing systems.
The collaboration across various disciplines, such as material science and electrical engineering, is vital to make systems compatible. Moreover, there's a need for standardization to facilitate smooth integration. Development of adapters or bridges that allow older systems to utilize terahertz sources could ease the transition.
"Achieving effective integration involves not only technology advancements but also strategic planning and collaboration."
By addressing these challenges, the future of terahertz source development can be more promising. Enhanced affordability, improved efficiency, and compatible systems could widen the scope of applications in medical imaging, secure communications, and more.
Future Directions in Terahertz Research
Future directions in terahertz research are crucial for advancing the field and enhancing understanding of terahertz sources. As technology evolves, the potential applications grow, thus necessitating continued exploration of innovative methods of generation and utilization. This section will explore emerging technologies, expanding applications, and the significance of collaboration across scientific disciplines.
Emerging Technologies
The landscape of terahertz generation is continually changing, with numerous emerging technologies that promise to revolutionize the field. Researchers are investigating new materials that might provide higher output power and improved efficiency. For example, advances in semiconductor technology, particularly with materials like graphene and topological insulators, have opened up new possibilities. These compounds exhibit unique electronic properties that can be harnessed to create efficient terahertz sources.
Some other interesting technologies include:
- Metamaterials: These artificially structured materials can manipulate electromagnetic waves in ways not possible with natural materials, potentially leading to innovative terahertz devices.
- Integrated Photonics: The integration of photonic components on a single chip could result in more compact and effective terahertz systems.
Investing in these emerging technologies can lead to substantial benefits. Innovations might not only enhance performance but also reduce costs, thus making terahertz applications more accessible.
Expanding Applications
As our understanding of terahertz sources deepens, so does their range of applications. From non-destructive testing to security screening, the potential uses are extensive. Medical imaging, for instance, stands to benefit significantly. Terahertz waves can penetrate tissue without causing damage, offering a new way to diagnose diseases, like skin cancer, at early stages.
In industry, terahertz technology is being explored for:
- Quality Control: It allows detailed analysis of materials without invasive techniques.
- Telecommunications: Higher data rates for wireless communication could be achieved using terahertz frequencies.
The expansion of applications is not only important for technological growth but also for economic benefits. As terahertz systems become more widely adopted, they can drive innovation and create new markets.
Collaboration Across Disciplines
The multifaceted nature of terahertz research necessitates collaboration across various scientific disciplines. This includes physicists, engineers, and medical researchers, each bringing unique perspectives and expertise. Interdisciplinary collaboration fosters innovation and accelerates breakthroughs.
Such collaborations can occur through:
- Joint Research Initiatives: Universities and industries can develop programs that focus on specific challenges within terahertz research.
- Conferences and Workshops: These events bring together experts from different fields to share knowledge and ideas, creating potential partnerships.
Furthermore, interdisciplinary efforts can lead to more robust funding opportunities and greater visibility for terahertz technology. Engaging various fields enhances the technological, practical, and commercial potential of terahertz sources.
"The future of terahertz research lies not only in technology development but also in the cooperation of diverse fields to unlock its full potential."
In summary, focusing on future directions in terahertz research is essential for embracing the rich opportunities present in this evolving field. Emerging technologies, expanding applications, and interdisciplinary collaboration serve to propel the discipline forward, enabling advancements in both science and technology.
The End
The conclusion of this article on terahertz sources holds great significance. It encapsulates the key findings and insights drawn from the various sections discussed. This synthesis of information not only reinforces the understanding of terahertz technology but also highlights its critical role in advancing scientific research and technological applications.
Summary of Key Points
In summary, terahertz sources are fundamental to a wide range of applications, from medical imaging to secure communications. Each source type, including solid-state sources, quantum cascade lasers, and photoconductive antennas, presents unique benefits and challenges. Understanding these aspects is vital for researchers and professionals aiming to innovate and enhance existing systems. Moreover, the mechanisms of generation, such as free electron lasers and nonlinear optical processes, shed light on the intricate processes that make terahertz technology possible. The article also addressed the current challenges in production costs and efficiency, emphasizing the hurdles that must be overcome for wider adoption.
Implications for the Future of Terahertz Technology
Looking forward, the implications of continued research into terahertz sources are vast. Emerging technologies suggest a potential for enhanced efficiency and applicability across diverse fields. As applications expand, particularly in medical diagnostics and security, the demand for efficient and cost-effective terahertz sources will likely increase. Collaboration among different disciplines can foster innovative approaches to overcoming existing challenges in integration and performance. Ultimately, the ongoing exploration and investment in terahertz technology could unlock transformative solutions that significantly impact various industries.