Understanding Flux Magnets: Their Principles and Uses
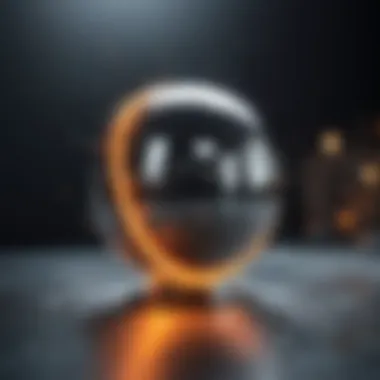
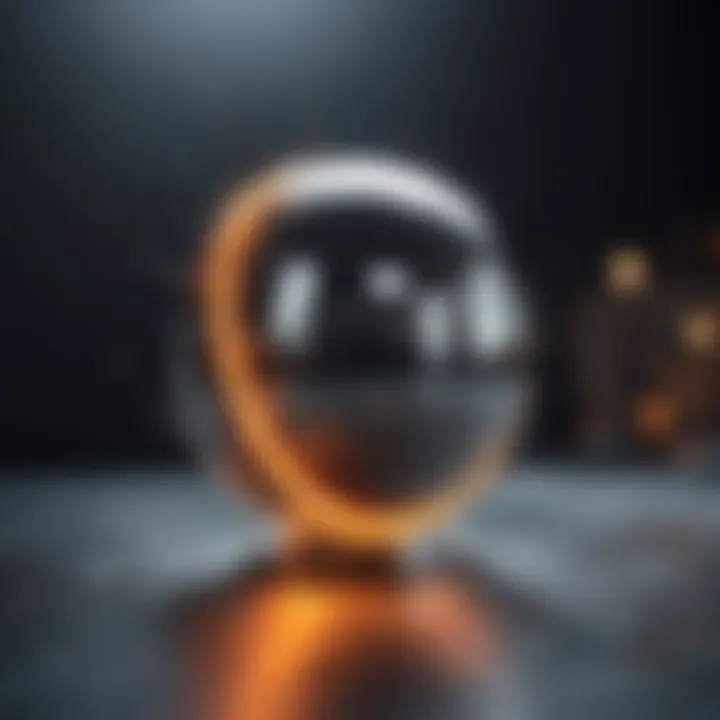
Intro
Flux magnets stand at the intersection of physics and practical technology, offering unique properties that have far-reaching implications in various fields. As we delve into their workings, we encounter a blend of intricate physics and real-world applications. This exploration not only takes us through the basic concepts of magnetism but also leads us to understand how these entities can influence modern innovations.
Flux magnets are fundamentally interesting. They carry the potential to transform both scientific theories and practical applications. From electric vehicles to advanced robotics, the significance of these magnets cannot be understated. An in-depth understanding is essential for students, researchers, educators, and professionals seeking to harness this technology.
As we peel back the layers, we will uncover how these magnets operate and the vital role they play in contemporary research and industry. The journey through the mechanisms, properties, and applications of flux magnets paints a vivid picture of what they truly are and how they shape our technological landscape.
Prelude to Flux Magnets
When diving into the realm of magnetic technology, flux magnets emerge as a topic of considerable intrigue. Their significance spans various domains, from industrial applications to cutting-edge scientific research. In this article, we will meticulously dissect the principles behind flux magnets and their far-reaching implications.
Understanding the basic principles of flux magnets is crucial, as they govern not just the behavior of electrical devices but also play a pivotal role in phenomena we experience daily. For instance, flux magnets serve as the backbone for electric motors, effectively converting electrical energy into kinetic energy. Their influence extends to transformers and generators, crucial components in our power infrastructure, making their study all the more relevant.
Definition and Basic Concepts
Flux magnets, fundamentally, are types of magnets where magnetic flux density falls within certain operational parameters. Their characteristics can vary widely based on material composition and structural design. The exploration starts with understanding key terms like magnetic flux, which refers to the total magnetic field passing through a given area. In practical terms, this can be seen in how these magnets behave under different conditions, such as temperature or physical stress.
Key Concepts:
- Magnetic Flux Density: This is defined as the amount of magnetic flux through a unit area.
- Permeability: The ability of a material to support the formation of a magnetic field within itself.
- Hysteresis: The lagging of the magnetic properties of a material when magnetic fields change.
Understanding these concepts leads to a nuanced comprehension of how magnets operate in conjunction with various electrical components.
Historical Context and Development
The journey of flux magnets is steeped in rich history, dating back to the early scientists and inventors who laid the groundwork for our understanding of magnetism. The work of renowned figures like Hans Christian Oersted, whose experiments in the early 19th century demonstrated the relationship between electric current and magnetic fields, provided vital insights that inform modern applications.
Over the years, the development of flux magnets has also been shaped by technological advancements. The inception of materials like neodymium iron boron (NdFeB) in the 1980s revolutionized magnet performance, leading to smaller, lighter, and more powerful magnets than ever before. This pivotal moment in history illustrated how innovation and research can drive technological leaps, impacting everything from consumer electronics to industrial machinery.
As we tread deeper into the examination of flux magnets, we will uncover both their physical principles and their practical applications, setting the stage for a deeper discussion of their role in various fields of technology. Understanding these elements is crucial for anyone engaged in fields such as engineering, physics, or applied sciences.
The Physics of Flux Magnets
Exploring the physics behind flux magnets is not just a cerebral exercise; it's the very foundation of how these devices function and their widespread applications. Understanding the physics can illuminate how energy is created, transferred, and utilized in various technologies today. The principles guiding this field are pivotal for advancing both science and industry, making this discussion imperative.
Electromagnetic Principles
Magnetic Fields and Flux
A crucial concept when we talk about flux magnets is the relationship between magnetic fields and the concept of magnetic flux. Magnetic fields represent a vector field around magnets where magnetic forces come into play. The term flux, in this context, refers descriptively to the total magnetic field passing through a certain area. One key characteristic of magnetic fields is how they can be manipulated; a change in magnetic field can induce electric current, which is practical for many devices, from generators to transformers.
The benefits of delving into magnetic fields and flux are multifaceted. For instance, they enable the efficient transfer of energy, critical in power generation and electric vehicle technologies. However, getting a grip on magnetic flux entails acknowledging its disadvantages as well. For instance, the complexity in calculating the exact flux in non-linear media can sometimes lead to inefficiencies in design and manufacturing.
A unique feature of this area is its applicability in modern technologies where high-efficiency energy transfer is sought. Innovations such as flux concentrators have demonstrated marked improvements in performance, showing how understanding this principle can lead to tangible advancements.
Maxwell's Equations in Context
Maxwell’s equations serve as the bedrock of electromagnetism, describing how electric and magnetic fields interact in terms of mathematical relationships. These equations are not just theoretical constructs; they are practical tools used to model different electromagnetic phenomena. Their key characteristic lies in encompassing all known electromagnetic interactions. Because of this, they are immensely valuable for practitioners in both academia and industry.
Integrating Maxwell's equations within the flux magnet dialogue is important for several reasons. Primarily, they help predict how field configurations affect performance efficiency in flux magnets. Their ability to describe dynamic systems allows for better designs and optimization. Yet, it's essential to mention the complicated nature of these equations. Although they offer precise frameworks, they can be daunting to navigate without adequate mathematical training. This could be a barrier when trying to implement effective flux magnet solutions in some applications.
Material Properties
In addition to electromagnetic principles, understanding the material properties of flux magnets is key. Here, we delve into two significant categories: ferromagnetism and ferrites, and superconducting materials.
Ferromagnetism and Ferrites
Ferromagnetism presents a specific feature of certain materials where they can be magnetized permanently. This characteristic underpins many practical applications in motors and generators and is essential in providing stability and reliability to devices that utilize flux magnets. Ferrites, which are ceramic compounds mostly made of iron oxide, exhibit ferromagnetic properties that are particularly useful in high-frequency applications.
The notable advantage of incorporating ferromagnetic materials is their high magnetic permeability, allowing them to sustain strong magnetic fields without excessive energy inputs. However, challenges lie within lower Curie temperatures, which can restrict their usability in high-temperature environments. In light of this, it's vital to consider the working conditions of flux magnets to choose materials that won't temper their effectiveness.
Superconducting Materials
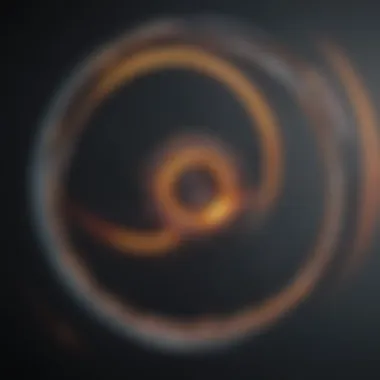
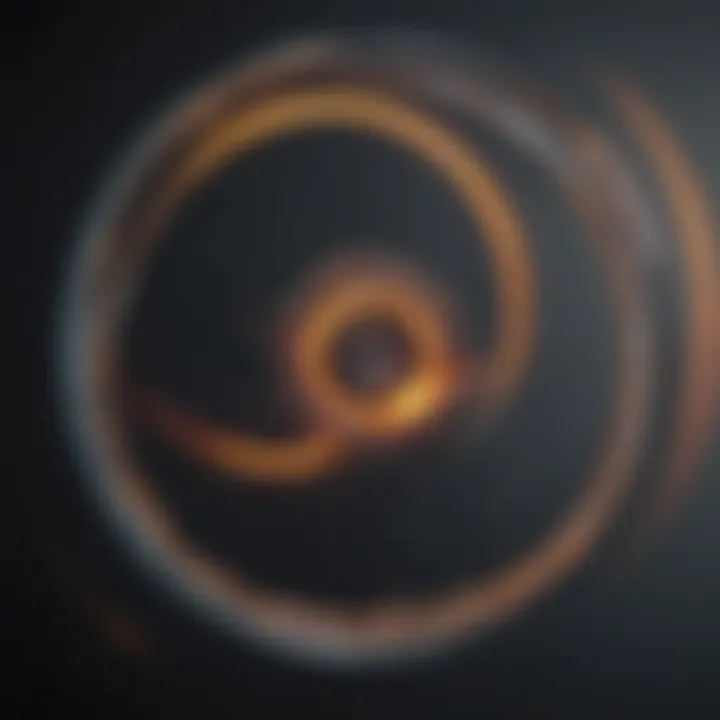
Superconducting materials add another layer of complexity and innovation onto the discussion of flux magnets due to their unique property of zero electrical resistance below certain critical temperatures. These materials can carry large currents without energy loss, opening new pathways in the realm of high-efficiency energy systems.
One major highlight of using superconducting materials is their ability to drastically reduce energy loss, making them appealing for applications like Magnetic Resonance Imaging and electromagnetic propulsion systems. However, the substantial cooling requirements necessary to achieve superconductivity can be a stumbling block, essentially adding cost and complexity to the systems developed.
Understanding both ferromagnetism and superconductivity is indispensable for any budding engineer or scientist interested in flux magnets.
Above all, integrating this foundational knowledge is paramount; recognizing the synergy between these concepts can foster advancements that resonate throughout technology and research.
Design and Structure of Flux Magnets
The design and structure of flux magnets play a pivotal role in determining their efficiency and capability. This section examines the geometrical configurations and component materials that go hand in hand to create a flux magnet that meets specific operational needs. Focusing on optimal designs leads to enhanced performance, better reliability, and increased application versatility. It's not just about how a magnet looks on paper, but how its structure interacts with its environment to deliver its purpose effectively.
Geometrical Configurations
Circular vs. Rectangular Designs
When addressing the geometrical configurations of flux magnets, the debate between circular and rectangular designs often arises. Circular designs are frequently praised for their uniform magnetic field distribution. This uniformity makes them ideal for applications requiring consistent magnetization, such as in electric motors or magnetic resonance systems. Meanwhile, rectangular designs allow for easy integration into tighter spaces, making them a popular choice for devices where space constraints line up with performance needs.
"The choice between circular and rectangular designs can be the difference between simply functional and exceptionally efficient applications of flux magnets."
The distinctive feature of circular designs lies in their symmetry, providing a balanced magnetic field that is less likely to cause fluctuations. In contrast, rectangular designs may lead to edge effects that can sometimes result in a less efficient magnetic coupling. The increasing need for designs that fit specific machinery often sways manufacturers toward rectangular options. The benefits of rectangular designs, particularly in constrained layouts, can outweigh their potential downsides if properly managed.
Layered Structures
Layered structures represent another critical design aspect. The layering technique involves stacking multiple magnetic materials to capitalize on their unique properties. This strategy can significantly improve the magnetic performance and enhance the thermal management of flux magnets, as different materials can be specifically chosen to complement each other.
One key characteristic of layered structures is their ability to combine high permeability with insulation layers. Integrating ferromagnetic materials like iron with insulating layers can protect against thermal degradation, which could be detrimental in applications subjected to variable temperatures.
However, while this approach offers enhanced performance, it can also introduce complexities in manufacturing. The more layers one adds, the more meticulous the design process becomes, which can potentially increase production costs. Nonetheless, for applications in scientific equipment or aerospace components where performance consistency is paramount, the advantages of layered structures often justify these challenges.
Component Materials
Core Materials Selection
The core materials used in flux magnets are quite foundational to their operational efficiency. In selecting core materials, factors such as magnetic permeability, coercivity, and thermal stability must all be taken into account. Silicon steel is a common core material for electrical transformers due to its excellent magnetic properties. On the other side of the spectrum, neodymium magnets, belonging to the permanent magnet category, boast exceptional magnetization capabilities, making them strong contenders for compact applications.
The unique feature of core materials is their capacity to influence the overall behavior of the magnet. Utilizing high-quality core materials can mean the difference between standard and superior performance in both energy transformation and generation settings. Still, the selection of core materials must be balanced with cost considerations and application-specific requirements.
Insulating Materials
Insulating materials also play an indispensable role in optimizing flux magnets. The choice of insulation affects thermal stability, efficiency, and overall longevity of the equipment. Polyimide films, widely utilized for their excellent thermal resistance, enable magnets to operate under rigorous conditions without losing efficiency. On the other hand, ceramic insulators provide robustness, making them suitable for high-voltage applications.
The key characteristic of insulating materials is that they not only protect the core from excessive heat but also minimize energy losses due to stray magnetic fields. However, there could be trade-offs involved; for instance, heavier insulating layers may add bulk to a design, impacting overall compactness. Yet, neglecting insulation could lead to early failure of magnetic systems, making the weight issue a necessary compromise in pursuit of durability and reliability.
Manufacturing Processes of Flux Magnets
Manufacturing processes are vital for the development of flux magnets, as these methods directly affect the performance, efficiency, and longevity of the final product. Understanding how these processes work provides insight into their practical applications and helps establish a solid basis for advancements in technology.
To truly grasp the significance of flux magnets, it’s crucial to consider different manufacturing techniques and their limitations, as well as the benefits they offer.
Sintering Techniques
Sintering techniques play an essential role in the manufacturing of flux magnets. This process involves heating powdered materials below their melting points, causing the particles to bond together into a solid mass. Sintering offers several advantages, including higher density and improved magnetic properties, which are fundamental in the functional performance of these magnets, particularly in commercial and industrial applications.
Powder Metallurgy
Powder metallurgy is a specific aspect of sintering that has gained considerable attention. It involves the utilization of metal powders as the raw material, which are then compacted and heated to form a solid structure. One of the key characteristics of powder metallurgy is its capability to produce complex geometries with minimal waste material. This makes it a popular choice for flux magnets, especially when intricate designs are demanded by modern technological needs.
The unique feature of powder metallurgy lies in its ability to control porosity and microstructure, allowing for the design of magnets with tailored properties. This can result in enhanced magnetic performance, as well as better thermal and mechanical stability. However, there are also disadvantages to be mindful of, such as the potential for high production costs and variability in quality control.
Hot Pressing
On the other hand, hot pressing is another notable technique within the realm of sintering processes. This method involves applying pressure and heat simultaneously to compact the powdered materials, further enhancing their density. The primary characteristic of hot pressing is that it can lead to superior mechanical properties in the final product. This makes it highly advantageous in applications where strength and reliability are paramount.
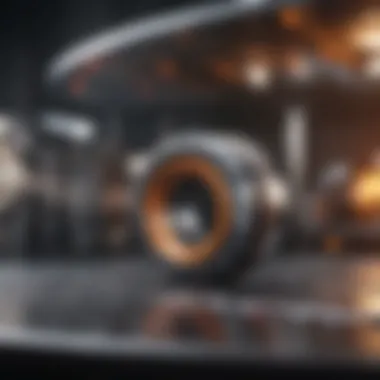
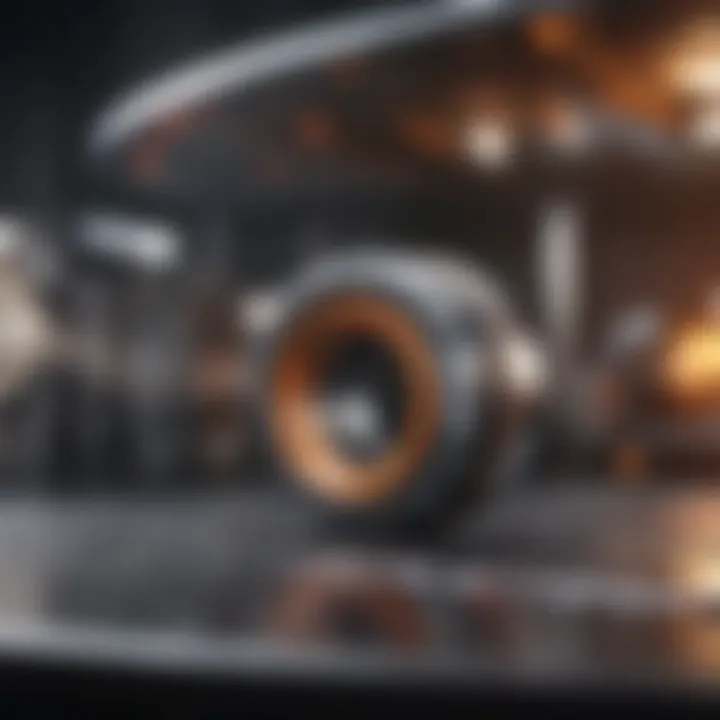
Hot pressing also introduces unique features such as the reduction of grain size, which can improve magnetic coercivity, a crucial factor in enhancing the stability of magnets under varying conditions. Nevertheless, this method can be resource-intensive, requiring careful design considerations and control over variables such as temperature and pressure to achieve desired results.
Magnetization Methods
In addition to manufacturing techniques, the subsequent magnetization of flux magnets is critical to their function. This process can significantly influence their performance characteristics, determining how effectively they can be integrated into various applications.
Magnetization
DC magnetization involves applying a steady direct current to the material, effectively aligning the magnetic domains within the magnet. This method is particularly powerful due to its ability to create strong and stable magnetic fields. A notable advantage of DC magnetization lies in its simplicity and effectiveness, allowing manufacturers to produce magnets with high consistency in their magnetic properties.
However, one must consider the limitations as well. DC magnetization can lead to the saturation of magnetic materials, thus capping the maximum achievable magnetic strength. Furthermore, it may not always be suitable for materials that are sensitive to direct current application.
AC Magnetization
In contrast, AC magnetization applies an alternating current to the material, which can induce a dynamic alignment of magnetic domains. This technique enjoys the benefit of being suitable for a wider variety of materials, including those that might not respond well to DC methods. The oscillations can also lead to a better uniformity of magnetic domains, enhancing performance across different operational contexts.
However, AC magnetization has its own challenges. Its effectiveness can be limited by frequency and other factors, leading to less predictability in final magnetic strength compared to DC. Furthermore, there can be additional equipment costs associated with the higher complexities of AC magnetization processes.
Applications of Flux Magnets
The role of flux magnets in both industry and scientific research cannot be overstated. These magnets have shaped numerous technologies and play vital roles in various applications, from powering devices to enabling complex experiments in labs. Understanding their applications not only emphasizes their importance but also sheds light on their impact on modern advances.
Industrial Uses
Electric Motors
Electric motors rely heavily on flux magnets to convert electrical energy into mechanical energy. They are the backbone of countless devices, from household appliances to industrial machinery. The key characteristic of electric motors is their efficiency; they can achieve a high level of performance with relatively low energy loss. This makes them a predominantly beneficial choice in contexts where energy conservation is a priority. Moreover, the unique feature of electric motors is the ability to deliver instant torque.
What sets these motors apart is their reliance on permanent and electromagnetic flux magnets, allowing them to generate powerful rotational forces. However, while the advantages are significant, there are also disadvantages to consider. For example, the use of certain materials in the construction of motors can raise costs, and magnetic interference can lead to inefficiencies if not managed properly.
Transformers and Generators
Transformers and generators are cornerstone technologies that harness the properties of flux magnets to transfer and convert electrical energy. A noteworthy aspect of these devices is their capacity to adjust voltage levels, which is crucial in optimizing energy transfer across long distances. This flexibility is precisely what makes transformers a popular choice in electrical grids.
The standout feature of transformers and generators is their use of magnetic flux to ensure that energy is efficiently stored or transferred. They leverage the principles of electromagnetic induction to function properly. However, one of the caveats includes potential losses during energy conversion, often termed core losses, which can hinder performance. Understanding these devices is essential not only for enhancing current technology but also for developing systems with improved efficiency.
Scientific Research Applications
Particle Physics Accelerators
In scientific research, particle physics accelerators are monumental. They utilize flux magnets to guide, focus, and accelerate subatomic particles to high speeds. A defining characteristic of these accelerators is their use of strong magnetic fields to control particle beams, making them pivotal for experiments in fundamental physics.
The unique attribute of particle physics accelerators lies in their ability to collide particles at unprecedented energies, allowing scientists to explore the building blocks of matter. However, there's a flip side; these high-energy environments can demand intricate designs and substantial infrastructure, which can escalate costs and complexity in operation.
Magnetic Resonance Imaging (MRI)
In the medical realm, Magnetic Resonance Imaging (MRI) represents a significant application of flux magnets, utilizing them to create detailed images of the body's internal structures. The core advantage of MRI technology is its non-invasive nature, which allows for profound insights into human anatomy without the use of radiation. This makes MRIs a popular choice for diagnostic purposes.
The unique aspect of MRI machines is their reliance on strong magnetic fields to align hydrogen atoms in the body, which is then manipulated to produce images. Although considered safe and effective, the drawbacks include high costs and lengthy scan times, which can be inconvenient for patients and healthcare providers. Nevertheless, the importance of MRI in modern medicine cannot be overstated; it has revolutionized how diseases are diagnosed and monitored.
"The impact of flux magnets spans various fields, confirming their significance in both technology and healthcare."
Through these applications, it's evident that flux magnets are not just passive components; they are active facilitators of innovation, influencing many aspects of our daily lives and the operations of vital technologies.
Challenges and Innovations in Flux Magnet Technology
The journey into the realm of flux magnets isn't devoid of hurdles. Challenges and Innovations in Flux Magnet Technology serve as a beacon for researchers and professionals alike, highlighting the constant evolution that accompanies this field. Understanding these complexities not only broadens our perspective on existing technologies but also paves the way for groundbreaking developments that can revolutionize various applications. Thus, recognizing performance limitations and recent advancements proves to be essential for navigating the future of magnet technology.
Performance Limitations
Thermal Limitations
One significant hurdle in flux magnet technology is thermal limitations. When operating at high temperatures, the performance of flux magnets can deteriorate significantly. The key characteristic that stands out here is the relationship between temperature and magnetic properties. Higher temperatures often lead to a reduction in magnetization, which can impact efficiency in devices like motors and generators. If we consider the way heat affects materials, it’s evident that increased thermal energy disrupts the alignment of magnetic domains, causing a decline in overall performance.
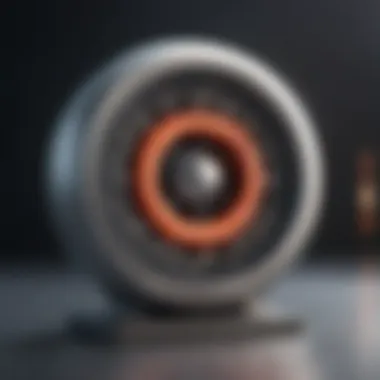
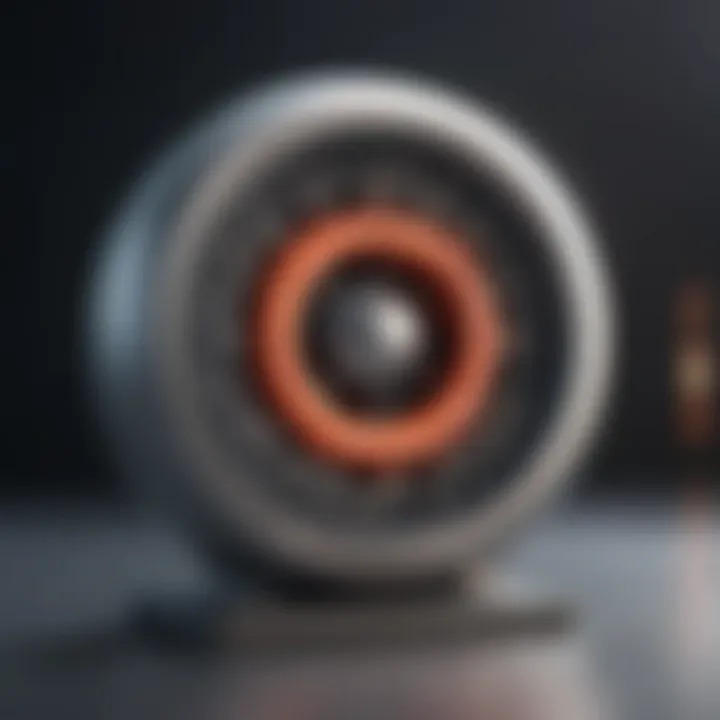
In this paragraph, the thermal limits underscore not just a challenge but also an opportunity for improvement. Researchers are keen to develop materials that offer stable magnetic properties under varying thermal conditions. This pursuit can enhance their usability across a broader range of industries, ultimately leading to better-designed products. However, the downside is that materials that withstand higher temperatures may be costlier or harder to manufacture.
Demagnetization
Another pressing issue is demagnetization. This phenomenon occurs when magnets lose their magnetic properties over time or due to external disturbances such as temperature fluctuations or mechanical stress. A notable aspect of demagnetization is its inevitability; it is a natural component of a magnet's lifecycle. Typically, the ongoing strain from operating conditions could lead to a loss of magnetization, which is quite detrimental in applications that rely heavily on consistent magnetic performance.
Yet, demagnetization also encourages innovation. For instance, researchers look into developing advanced magnet materials that exhibit greater resistance to demagnetization. This search brings us closer to creating robust systems that maintain effectiveness over longer periods. The flip side, however, is that the materials developed to counteract demagnetization can sometimes introduce new complexities in design and production.
Recent Advancements
Development of High-Temperature Superconductors
The development of high-temperature superconductors marks a significant stride in the realm of flux magnets. These materials leverage properties that allow them to conduct electricity with zero resistance at relatively higher temperatures compared to traditional superconductors. The key characteristic of high-temperature superconductors is their ability to generate substantial magnetic fields, which opens new avenues for applications in power systems and medical imaging technologies.
The benefit of using these superconductors lies in their enhanced efficiency and reduced energy costs. Additionally, the development of high-temperature superconductors diminishes the reliance on costly cooling systems, providing cost-effective solutions for various implementations. Yet, one must be cautious—these materials can be complex to fabricate and incorporate into existing systems.
Novel Manufacturing Techniques
Another area of innovation emerges with novel manufacturing techniques. Advances in production methods like 3D printing and additive manufacturing allow for more intricate designs in flux magnets that were previously unattainable. These techniques embody precision and customization, enabling inventors to create bespoke solutions tailored to particular needs.
Moreover, about the characteristics, these novel approaches facilitate the production of lighter, stronger magnets with improved performance profiles. There’s, however, a trade-off; with complexity comes the challenge of ensuring consistency and quality, which may hinder widespread adoption.
"The only limit to our realization of tomorrow will be our doubts of today."
In summary, where challenges exist, so too does innovation. The dynamics of flux magnet technology unveil a landscape ripe for exploration, with each limitation fostering a new idea or methodology. Recognizing the intricacies of thermal limitations and demagnetization, along with the burgeoning advancements, reveals a bright future that’s already unfolding in the realm of flux magnets.
Future Directions in Flux Magnet Research
The study of flux magnets is continually evolving, driven by the need for advancements in performance and efficiency. As researchers and engineers delve into this complex field, it becomes clear that future directions in flux magnet research hold significant promise. By exploring novel materials and interdisciplinary approaches, we can enhance current technologies and facilitate innovations across various sectors.
Emerging Materials
Graphene and Two-Dimensional Materials
Graphene, often hailed as a miracle material, showcases remarkable electrical and thermal conductivity. In the context of flux magnets, its key characteristic lies in its incredibly thin structure, made up of a single layer of carbon atoms arranged in a hexagonal lattice. This property allows for minimal magnetic resistance, enhancing the performance of devices that rely on flux magnets.
Moreover, its lightweight and flexible nature makes graphene a compelling choice in developing advanced magnetic applications, such as wearable devices and portable sensors. The unique feature of graphene is its ability to support magnetism at room temperature, a significant ventaja over many existing materials. However, the challenge lies in its scalable production and integration with traditional magnet technologies.
Nanostructured Magnets
Nanostructured magnets represent another frontier in flux magnet research. These materials consist of particles at the nanoscale, which significantly influence their magnetic properties. Their primary characteristic is the increased surface area, which can lead to enhanced magnetic coherence and energy efficiency.
What makes nanostructured magnets particularly advantageous is their ability to remain stable even at elevated temperatures. This thermal robustness is critical in applications where traditional magnets tend to demagnetize. Yet, the complexity of synthesizing these materials can be a steep hurdle, requiring sophisticated techniques that might not be readily available in every laboratory.
Integrated Systems and Interdisciplinary Approaches
The notion of integrated systems emerges as vital in future flux magnet research, where the synergy of various disciplines comes into play. Combining insights from electrical engineering, material science, and computational modeling could yield powerful innovations. For instance, integrating machine learning algorithms can optimize the design process, predicting performance outcomes based on specific parameters.
By fostering interdisciplinary collaboration, researchers can tackle the multifaceted challenges associated with flux magnet technologies. As a result, we may witness breakthroughs that are not just piecemeal improvements but rather holistic advancements leading to the next generation of magnetic applications.
The way forward in flux magnet research is undeniably linked to exploring novel materials and embracing collaborative interdisciplinary approaches. This dual strategy could redefine the landscape of their applications, paving the way for advancements we can only begin to imagine.
End
In examining the realm of flux magnets, this article has traversed not just the principles underlying their operation, but also the intricate applications that underscore their importance. The primary objective throughout has been to unravel complexities and deliver a clear narrative tailored to students, researchers, educators, and professionals in the field. Therefore, the significance of understanding flux magnets cannot be overstated.
Summation of Key Points
First, it is crucial to highlight some key aspects discussed in the preceding sections:
- Definition and Basics: The foundational principles of flux magnets provide a groundwork for understanding their functionality. This includes relationship between electromagnetism and material properties.
- Physics Fundamentals: We explored how magnetic fields and Maxwell’s equations interplay within each flux magnet, establishing their behavior under varying conditions.
- Design Specifications: The geometrical configurations as well as component material choices are pivotal in tailoring flux magnets for specific applications, be it in industry or scientific research.
- Manufacturing Insights: Various sintering techniques and magnetization methods were examined, shedding light on production processes crucial for high-performance output.
- Challenges and Innovations: Performance limitations explored in this article bring to focus the creative approaches being developed to enhance flux magnet technology.
- Future Directions: Finally, emerging materials and interdisciplinary approaches present exciting avenues for future research and development in the flux magnet domain.
The Significance of Flux Magnets
Flux magnets serve as cornerstones in a variety of applications across sectors. In electric motors and transformers, their efficacy underpins energy efficiency and operational reliability. Within the context of scientific research, they are indispensable in tools like Particle Physics Accelerators and MRI machines, propelling advancements in both diagnostic medicine and fundamental science.
Their versatility allows for innovations that intertwine with emerging technologies, setting the stage for breakthroughs yet to come.
Understanding flux magnets translates to recognizing their potential impact on technology’s evolution. They not only facilitate current technological advancements but also pave the path for future discoveries and innovations.