Understanding the Formation of Metals
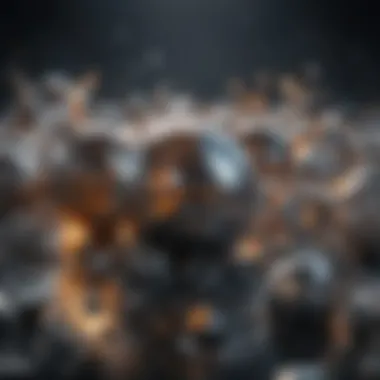
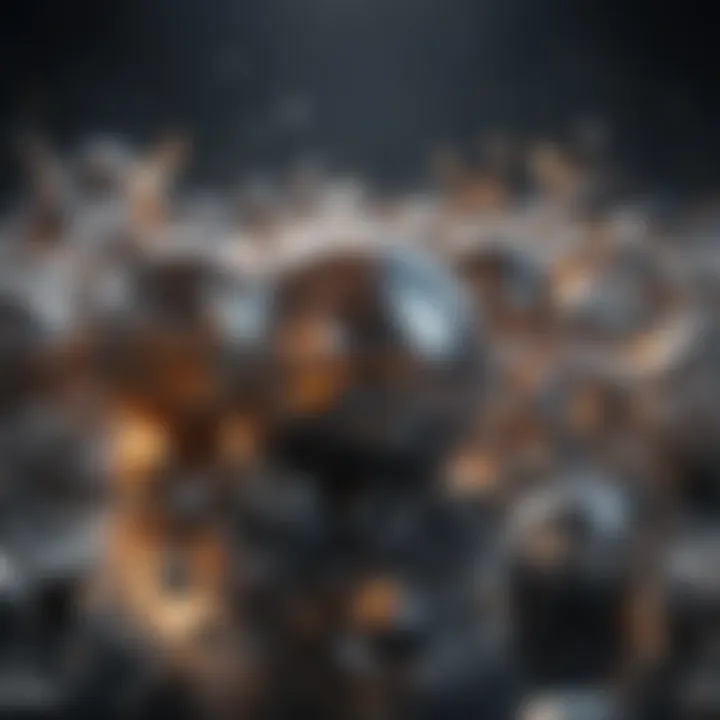
Research Background
The scientific problem surrounding metal formation includes how primordial elements evolve into distinct metal types through geological processes over billions of years. Past studies have examined various aspects of metal formation, from the crystallization processes to the influence of temperature and pressure on structural properties. This historical perspective offers a foundation for current research in metallurgy and its applications.
Historically, studies have highlighted natural metal deposits, often tied to volcanic activity, sedimentation, and other geological phenomena. Researchers such as Frank C. Hawthorne have contributed significantly to our understanding of mineral structures and their implications for metal properties. These insights are foundational for exploring how metals behave under different physical conditions.
Findings and Discussion
Current research on metal formation reveals several key results. First, the bond strengths and geometries of metal atoms are influenced by environmental factors, including temperature and chemical composition. For example, metals like gold and copper exhibit strong metallic bonding, which greatly affects their malleability and electrical conductivity.
Furthermore, phase transformations play a crucial role in determining metal characteristics. As temperature and pressure vary, metals may exist in different crystalline phases, which can affect both their physical and chemical behaviors. For instance, iron transitions from a face-centered cubic structure to a body-centered cubic structure, altering its properties notably.
"The understanding of bonding and phase transitions in metals is vital for optimizing their use in various applications."
Interpretation of these findings underscores the significance of both natural processes and advanced metallurgy techniques. Understanding how metals form has implications for resource extraction, sustainable development, and innovation in material design. Today, methods like electrolysis, smelting, and thermochemical processes are used to create metals with specific desired properties, crucial for industries such as aerospace, automotive, and electronics.
Ultimately, the intricate journey of metals from elemental formation to industrial utility remains a rich and dynamic field of study.
Intro to Metal Formation
Definition of Metals
Metals can be defined as substances characterized by their ability to conduct electricity and heat, as well as their malleability and ductility. They typically possess a shiny appearance and exist in solid form at room temperature, with the notable exceptions of mercury and gallium. The atomic structure of metals is primarily based on metallic bonding, where electrons are shared among a lattice of atoms, creating a strong cohesive force that results in their distinct physical properties.
Historical Context
The history of metal formation is intertwined with the evolution of human civilization. Early humans utilized naturally occurring metals like copper and gold, leading to the development of metallurgy as a crucial craft. The discovery of smelting processes, allowing extraction of metals from ores, marked significant advancements.
The Bronze Age saw the first major use of alloys, combining copper and tin to create bronze, which enhanced tools and weapons. The subsequent Iron Age further transformed societal structures as iron became the dominant metal. Understanding this historical context emphasizes not only the technological evolution but also the socio-economic impacts of metal usage throughout history.
Elemental Composition of Metals
Understanding the elemental composition of metals is crucial when exploring their formation. Each metal is defined by its unique atomic structure, which directly influences its physical and chemical properties. The characteristics of metals such as hardness, conductivity, and malleability are rooted in their elemental makeup. By examining these properties, we gain insights into how metals can be used in various applications, from construction to electronics.
Common Elements in Metal Formation
- Iron: Known for its strength and durability, iron is the main component of steel. Its widespread use in construction and infrastructure is well-documented.
- Copper: This element is highly conductive, making it ideal for electrical applications. It is also resistant to corrosion.
- Aluminum: Lightweight yet strong, aluminum is prized for its versatility in aerospace and automotive industries.
- Zinc: Often used as a protective coating for iron and steel, zinc enhances corrosion resistance.
- Lead: Historically significant, lead is used in batteries and radiation shielding, although it has seen reduced use due to health concerns.
The elemental composition of these metals not only defines their utility but also influences their environmental impact and recyclability in metallurgical practices.
Rare Metals and Alloys
Rare metals, such as platinum, gold, and tungsten, along with their alloys, represent a specialized segment of metal formation. These metals are less abundant but have superior properties that make them invaluable in many high-tech and industrial applications.
- Platinum: Notable for its resistance to corrosion and high melting point, it is used in catalytic converters and jewelry.
- Gold: Beyond its aesthetic value, gold plays a critical role in electronics due to its excellent conductivity and resistance to oxidation.
- Tungsten: Known for having the highest melting point of any metal, tungsten is essential in filament production for light bulbs and in various heavy-duty applications.
Alloys, which are combinations of two or more metals, can enhance their properties beyond what each metal can achieve alone. For instance, stainless steel, made from iron, nickel, and chromium, is highly resistant to rust and corrosion, expanding its usage in automotive and medical industries.
In summary, the elemental composition of metals and their alloys not only affects their properties and applications but also shapes the metallurgical processes used to extract and manipulate them. Such understanding is necessary for innovations in material science and sustainable practices.
Geological Processes of Metal Formation
Igneous Processes
Igneous processes involve the cooling and solidification of molten rock, known as magma or lava. They are responsible for the formation of many metal ores. As magma rises through the earth's crust, it can bring with it dissolved metals, which may later crystallize as the magma cools. For instance, copper, nickel, and platinum can be concentrated through this mechanism.
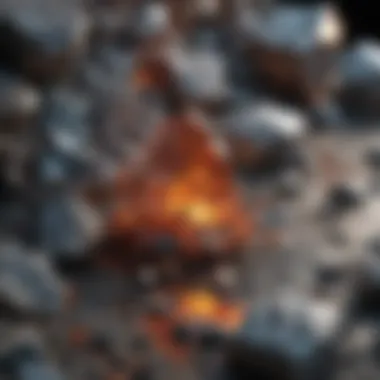
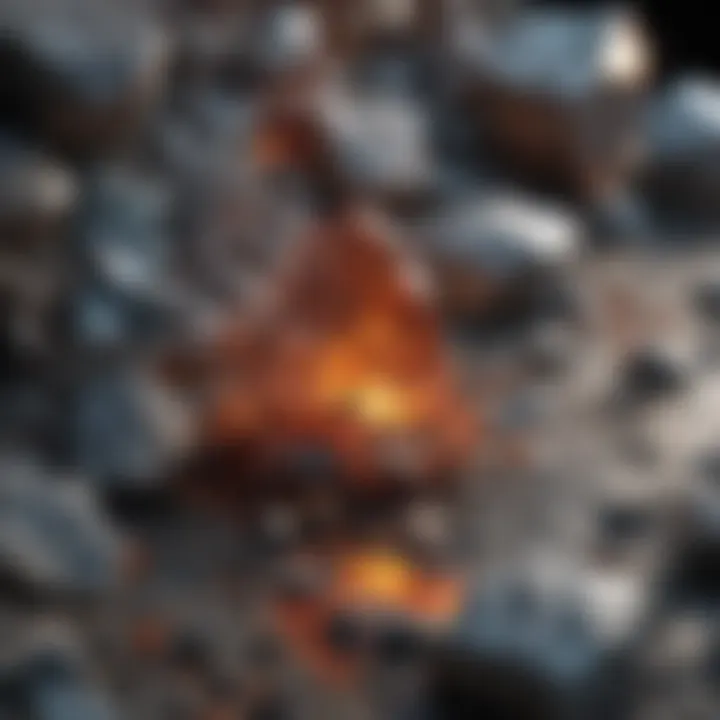
The process occurs in various environments, such as
- Intrusive igneous formations where magma cools slowly underground, leading to larger crystals.
- Extrusive formations, where lava cools rapidly on the surface, resulting in finer crystals.
Important deposits often form in specific mineral-rich zones, typically characterized by granite and other acidic rocks. These are significant for mining operations and economic viability.
Sedimentary Processes
Sedimentary processes play an important role in the transport and deposition of metals. This occurs largely through weathering and erosion, where rocks break down over time and metals are carried away by water or wind. Over time, these materials settle and form layers that can become concentrated in specific locations.
Sedimentary environments can vary widely, including rivers, lakes, and oceans. This diversity allows for the formation of different types of metal deposits, including:
- Banded Iron Formations (BIFs), primarily rich in iron.
- Sandstone-hosted deposits, which can contain zinc and lead.
The natural filtration processes within rivers or coastal areas allow for metals to accumulate in economically recoverable concentrations. As such, sedimentary processes are essential for understanding the large-scale dynamics of metal formations and accessibility.
Metamorphic Changes
Metamorphic changes involve the transformation of existing rocks under heat and pressure. This process can result in the recrystallization of minerals, which may generate new metal-rich deposits.
Common metamorphic processes include:
- Contact metamorphism, where rocks are altered by heat from nearby molten rock, often leading to increased metal concentrations.
- Regional metamorphism, where large areas of the earth’s crust are subjected to heat and pressure over long periods, which can also create significant metal deposits.
Metamorphic processes contribute to the understanding of ore genesis. They can enhance recovery methods through the alteration of the mineralogy and texture of metal-bearing rocks.
Understanding these geological processes is vital for those in metallurgy and extraction industries, as they provide the foundational knowledge needed to locate and economically extract metals from the earth’s crust.
Chemical Bonds and Metallic Structures
The topic of chemical bonds and metallic structures is crucial in understanding the nature of metals and their unique properties. Metals exhibit specific characteristics due to the way their atoms are arranged and bonded. This section delves into the significance of metallic bonding and crystal lattice formation, which together form the backbone of metallic structures.
Metallic Bonding
Metallic bonding is a type of chemical bonding that occurs between metal atoms. In metallic bonds, electrons are not bound to any specific atom. Instead, they form what is often referred to as an "electron sea". This sea of delocalized electrons enables metal atoms to slide past each other while still being bound together by their positively charged atomic cores.
The importance of metallic bonding can be summarized as follows:
- Electrical Conductivity: The presence of free-moving electrons allows metals to conduct electricity efficiently. As electrons move freely in response to an electric field, metallic substances can carry electrical current.
- Thermal Conductivity: Similarly, metallic bonds contribute to high thermal conductivity. The delocalized electrons can transfer energy, facilitating the movement of heat through the metal.
- Malleability and Ductility: The ability of metals to be hammered into thin sheets (malleability) or drawn into wires (ductility) is a direct result of metallic bonding. When stress is applied, the layers of atoms can shift without breaking the overall structure.
These characteristics are essential for the practical applications of metals in industry and technology.
Crystal Lattice Formation
Crystal lattice formation refers to the organized arrangement of atoms in a crystal structure. In metals, this structure emerges from the metallic bonding that governs their behavior. The crystal lattice contributes to the stability and overall properties of metals.
Key aspects of crystal lattice formation include:
- Types of Lattice Structures: Metals can form various types of lattice structures such as face-centered cubic, body-centered cubic, and hexagonal close-packed. Each of these structures results in different mechanical properties.
- Influence on Properties: The arrangement and type of lattice influence important physical properties including density, melting point, and overall strength. For example, face-centered cubic structures tend to be denser and are generally more malleable than body-centered cubic structures.
Understanding crystal lattice formation is vital for scientists and engineers when devising new materials or improving existing ones.
"The crystallography of metals not only determines their physical properties but also their potential uses in various advanced applications."
Investigating Metallic Properties
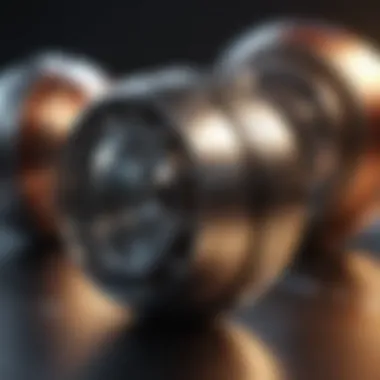
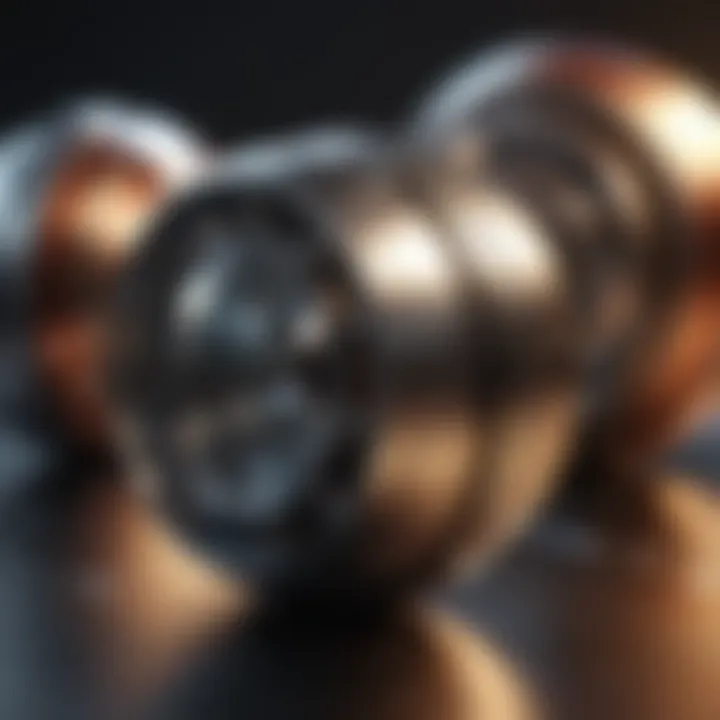
Investigating metallic properties is essential for understanding how metals behave in various applications. The characteristics of metals directly influence their usability in industrial processes and everyday products. Knowledge of these properties helps in selecting suitable materials for specific tasks, leading to more efficient and effective designs.
Electrical Conductivity
Electrical conductivity is one of the key characteristics of metals. Metals like copper and aluminum are renowned for their ability to conduct electricity. The presence of free electrons in metallic structures enables efficient electron flow, making metals ideal for electrical applications.
- Copper is the most widely used metal for electrical wiring due to its low resistivity and high conductivity.
- Aluminum, while less conductive than copper, is lighter and often used in high-voltage electrical transmission lines.
The understanding of electrical conductivity is vital for advancements in technology. For instance, semiconductors and superconductors demonstrate how manipulation of metallic properties can lead to innovative applications in electronics and energy.
Thermal Conductivity
Thermal conductivity is another significant property of metals, defined as the ability of a material to conduct heat. This property is crucial in various industrial applications, including heat exchangers and cookware.
- Metals such as copper and aluminum again stand out due to their high thermal conductivity.
- High conductivity contributes to efficient thermal management in engines and electronic devices, directly impacting performance.
In construction, the thermal properties of metals influence energy efficiency. Builders must pay attention to a material's thermal conductivity to improve insulation and minimize heat loss or gain.
Malleability and Ductility
Malleability and ductility refer to a metal's ability to deform under stress without breaking. Malleable metals can be rolled into thin sheets, while ductile metals can be stretched into wires.
- Gold and silver are highly malleable, used in jewelry and electronics due to their ability to be shaped easily without fracturing.
- Steel showcases excellent ductility, making it suitable for construction and manufacturing industries.
The significance of these properties cannot be understated. They dictate how metals can be processed and utilized in various fields, from automotive to aerospace applications.
Understanding the properties of metals allows researchers and engineers to develop new materials and technologies, enhancing our industrial capabilities.
Modern Metallurgical Techniques
Modern metallurgical techniques are essential for understanding the current landscape of metal production and manipulation. These techniques have been developed to optimize the extraction and processing of metals, adapting to both environmental challenges and technological advancements. The importance of this topic lies in its implications for efficiency, sustainability, and innovation in metallurgy.
Extractive Metallurgy
Extractive metallurgy refers to the branch of metallurgical engineering that deals with the extraction of metals from their ores. This involves multiple processes that convert ore into pure metal. The primary methods of extraction include pyrometallurgy, hydrometallurgy, and electrometallurgy.
- Pyrometallurgy: This method employs high temperatures to extract metal. It is typically used for metals such as copper and lead. The process involves smelting ore to separate the metal from impurities.
- Hydrometallurgy: Involves using aqueous solutions to extract metals. It is effective for metals like gold and silver. During this process, leaching occurs, where reagents dissolve the desired metals from ore.
- Electrometallurgy: This technique uses electrical energy for extraction, commonly exemplified by electrolysis, where electric currents conduct chemical changes in a solution.
Each of these methods carries specific benefits. Pyrometallurgy can process a large volume of ore, while hydrometallurgy tends to be more environmentally friendly as it often requires less energy. Electrometallurgy is critical for obtaining high-purity metals, essential in advanced applications.
"Extractive metallurgy not only affects economic factors but also plays a significant role in environmental sustainability by reducing waste and energy consumption."
Recycling Processes
Recycling processes in metallurgy underscore the importance of sustainability and resource conservation. Recycling metal minimizes the need for new raw materials and helps reduce environmental damage caused by mining.
The recycling process typically involves:
- Collection and Sorting: Metals are collected from various sources such as scrap yards, manufacturing waste, and consumer products. Proper sorting is vital to ensure the recovery of specific metals.
- Processing and Refining: The collected metals undergo processing. This often includes shredding, melting, and refining to remove impurities and restore metallic properties.
- Reuse in Production: After refining, the recycled metals are ready to be reused in manufacturing new products.
Recycling leads to a significant reduction in energy usage and greenhouse gas emissions. Additionally, it contributes to the circular economy, where materials are reused rather than discarded. This is not just prudent for the environment; it's beneficial economically for industries aimed at lowering production costs.
Environmental Considerations in Metal Formation
Sustainability Issues
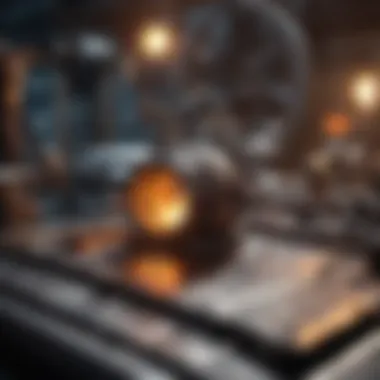
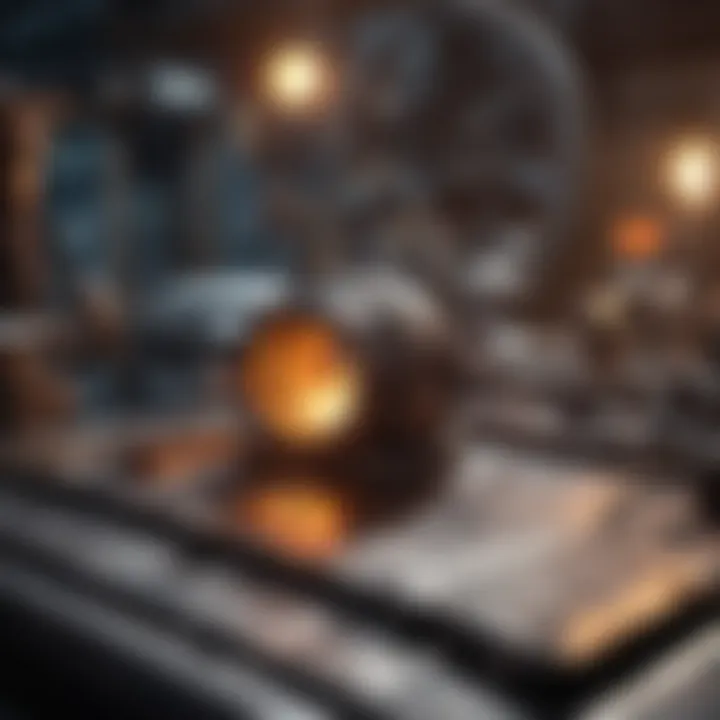
Sustainability in metal formation emphasizes the need to minimize ecological footprints while meeting the demand for metals. One key issue is the energy consumption associated with metal extraction. Processes like smelting and refining typically require substantial energy inputs, which often come from fossil fuels. This results in greenhouse gas emissions that contribute to climate change. To counteract this, many companies are now exploring renewable energy sources for their operations.
Another important factor is the efficient use of resources. Metals are finite, and their extraction has an inherent environmental cost. Practices such as recycling metals can reduce the need for new extraction, thus conserving natural resources and decreasing waste. Moreover, innovations in metal recovery techniques, including hydrometallurgy and biotechnological methods, have emerged to enhance sustainability while mitigating environmental harm.
"Sustainable practices in metallurgy are not just beneficial for the environment; they enable long-term profitability and resource security."
Impact on Ecosystems
The extraction and processing of metals can have profound impacts on ecosystems. The immediate effects are often visible through habitat destruction. Mining operations can lead to deforestation and soil degradation, which disrupt local wildlife habitats. Water sources can also be affected. Contamination from mining solutions can pollute rivers and streams, harming aquatic life.
Additionally, the runoff from mining sites may carry heavy metals into surrounding environments. This contamination poses risks not only to wildlife but also to human populations living nearby. It can accumulate in the food chain, leading to long-term health risks.
Efforts to mitigate these effects include implementing stricter regulations on mining practices and rehabilitation efforts post-extraction. Rehabilitation aims to restore the natural landscape and reestablish the flora and fauna originally present in the area. By adopting such measures, the metal industry can reduce its negative impacts on ecosystems, fostering a more balanced relationship with nature.
Case Studies in Metal Formation
Case studies in metal formation illustrate the practical applications and implications of metallurgical processes. Understanding how metals are extracted and produced can shed light on the complexities and nuances involved in the industry. This section focuses on two primary examples: copper and aluminum. Each metal presents its own set of challenges and methods which not only emphasize the advancements in technology but also highlight the environmental considerations and economic impacts linked to metal production.
Copper Extraction Methods
Copper extraction methods vary significantly based on the types of ores present and their geographical locations. One traditional method is pyrometallurgy, where copper is extracted from its ores through heating and melting processes. The main steps in pyrometallurgy include:
- Concentration: This involves crushing the ore and separating copper minerals from waste material using flotation methods. This initial step reduces the volume of the material to be processed.
- Smelting: The concentrated ore is then heated in a furnace, mixed with silica and limestone. This process produces molten copper and slag, which separates impurity materials.
- Refining: Molten copper undergoes electrorefining, where it is purified into a high-quality form, suitable for industrial use.
Another significant method is hydrometallurgy, which uses chemical solutions to extract copper from ores. Key components include:
- Leaching: A solvent, commonly sulfuric acid, helps dissolve copper from the ore into a solution.
- Solvent Extraction: The copper-laden solution is treated to remove impurities, resulting in a more concentrated copper solution.
- Electrowinning: Electricity is used to deposit pure copper metal from the solution, completing the extraction process.
This combination of methods allows for both efficiency and adaptability to various ore types. The economic significance of copper extraction plays an essential role in many industries, including electronics and construction.
Aluminum Production Processes
The production of aluminum is primarily dominated by the Bayer and Hall-Héroult processes, which work in tandem to extract aluminum from bauxite ore. These processes are crucial to the modern material landscape and have significant energy requirements, mainly due to the electrification needed. Here’s an overview:
- Bayer Process: This method starts with bauxite, which is treated with sodium hydroxide at high temperatures. This step leads to the dissolution of aluminum oxides and leaves behind impurities. The resultant solution is allowed to cool. As it does, aluminum hydroxide crystals precipitate out.
- Calcination: The precipitated aluminum hydroxide is then heated in rotary kilns or fluidized bed calciners, releasing water and producing alumina.
- Hall-Héroult Process: The alumina undergoes electrolysis in molten cryolite, where it is subjected to a direct current. This setup facilitates the reduction of alumina to aluminum metal, which collects at the bottom of the electrolytic cell. The remaining oxygen combines with carbon from the anode, producing carbon dioxide.
The challenges in aluminum production relate mainly to energy consumption and environmental impact. Recycling plays a pivotal role in mitigating these effects by significantly reducing energy usage compared to virgin aluminum production.
"Recycling aluminum saves 95% of the energy required to produce new aluminum from ore."
In summary, examining case studies such as copper and aluminum extraction provides key insights into the methodologies used in metal production. The implications of these processes resonate far beyond the laboratory or industrial setting, affecting economic growth and environmental sustainability in significant ways.
Culmination
First, it outlines the fundamental principles of how metals are formed from elemental origins, which is essential for both academic research and practical metallurgy. This knowledge aids in developing efficient extraction methods and refining processes.
Second, discussing the modern metallurgical techniques serves to highlight innovations in the industry. The recycling of metals, for example, significantly reduces environmental impacts compared to traditional mining, emphasizing the critical need for sustainable practices in metal production.
Third, the environmental considerations surrounding metal formation cannot be overlooked. Understanding the consequences of metal extraction and usage informs policy decisions and encourages responsible practices that minimize ecological disruption.
"Knowledge of metal formation provides essential insights for future technological innovations and environmental stewardship."
Summary of Key Points
- Understanding metallic properties is vital for applications in electronics, construction, and manufacturing.
- Sustainable practices in metal extraction and recycling are crucial for minimizing environmental impacts.
- The integration of multiple disciplines enhances our approach to metallurgy and encourages innovation.
Future Directions in Metallurgy
As the world progresses, the future of metallurgy must consider several advancements and changes:
- Green Metallurgy: The development of eco-friendlier extraction methods is imperative. This includes new technologies that minimize carbon emissions and reduce waste.
- Advanced Alloys: Research into lightweight and high-strength alloys will be essential. These materials can improve efficiency across several sectors, from aerospace to automotive.
- Recycling Innovations: Continued investment in recycling technologies and methods is necessary. Maximizing metal recovery from waste will create a circular economy in metallurgy.
- Interdisciplinary Collaboration: Future advancements will benefit from collaborations between scientists, engineers, and environmentalists. Sharing knowledge can lead to breakthroughs in material science.
The evolving landscape of metallurgy presents numerous opportunities for research and development. Staying informed and engaged with these changes is crucial for students, researchers, educators, and professionals in the field.