Understanding Mass Spectrometry: Principles and Applications
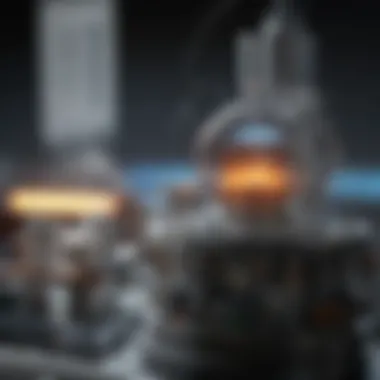
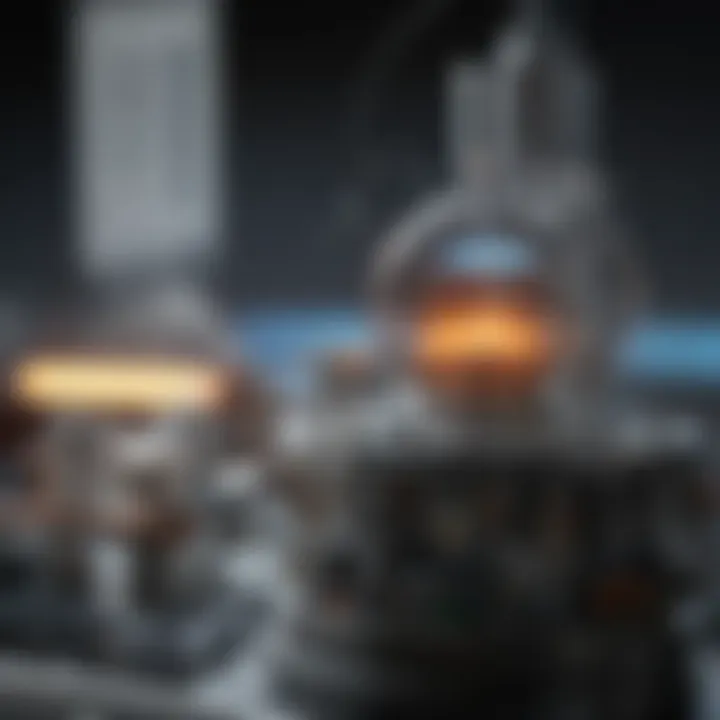
Intro
Mass spectrometry stands as a significant analytical tool within the realm of chemistry and beyond. Its ability to break down and analyze chemical compounds provides essential insights across various scientific fields. This technique enables researchers to identify molecular structures, quantify substances, and even detect trace materials. The relevance of mass spectrometry is underscored by its diverse applications, from pharmaceuticals to environmental science.
This article aims to delve into the fundamental principles and intricate workings of mass spectrometry. We will explore its types, operational mechanisms, and essential components that make it work. Recent advancements in mass spectrometry and their implications for research and industry will also be discussed. By understanding these elements, readers can gain a comprehensive overview of mass spectrometry's transformative impact on modern science.
Research Background
Overview of the scientific problem addressed
In a world where accurate chemical analysis is crucial, mass spectrometry addresses the pressing problem of how to effectively separate and identify compounds in complex mixtures. Traditional methods often struggle with sensitivity and specificity. Mass spectrometry excels in these areas, offering a solution that is both reliable and efficient. Its ability to analyze small amounts of substances makes it invaluable in various contexts.
Historical context and previous studies
The history of mass spectrometry traces back to the early 20th century when J.J. Thomson first introduced the concept of the mass spectrometer. Since that time, the technology has seen significant developments. Early versions were limited by their capabilities and resolution. However, over the decades, advancements in instrumentation and techniques have vastly improved the efficacy and versatility of mass spectrometry.
Prominent studies have expanded the understanding of mass spectrometry's applications, from the detection of biomolecules in biological research to its role in environmental monitoring. Key insights from these studies underscore the technique's flexibility in addressing diverse analytical challenges.
"Mass spectrometry has revolutionized analytical chemistry by enabling researchers to analyze complex substances with unprecedented accuracy."
This backdrop lays the foundation for exploring mass spectrometry in greater detail, thus paving the way for a deeper understanding of its principles and applications.
Preamble to Mass Spectrometry
Mass spectrometry stands at the forefront of analytical chemistry, serving as a pivotal technique for the identification and quantification of chemical substances. Its importance stems from its capacity to provide detailed insights into the mass-to-charge ratio of ions, enabling the study of complex mixtures, small molecules, and biomolecules alike. This method is indispensable in various scientific disciplines, including pharmaceuticals, environmental analysis, and proteomics.
The introduction of mass spectrometry to this article sets the stage for a deeper understanding of its operational specifics and broad applications. By dissecting the principles that underpin this technology, we aim to equip students, researchers, and professionals with a solid foundation in mass spectrometry's functionalities.
In dissecting the concept of mass spectrometry, we focus on how it transforms scientific inquiry and research. As an analytical tool, it enhances our ability to analyze materials quickly and accurately, streamlining processes that would otherwise require lengthy methods. Moreover, the flexibility it offers in analyzing diverse samples enhances its relevance across multiple fields.
By grasping the fundamental aspects, readers can appreciate the nuanced applications that follow, paving the way for more advanced discussions about challenges and innovations in mass spectrometry.
Definition of Mass Spectrometry
Mass spectrometry is defined as an analytical technique that measures the mass-to-charge ratio of charged particles. This process involves the generation of ions from chemical substances, followed by the sorting and detection of these ions based on their mass. The result is a mass spectrum, which is a graphical representation displaying the relative abundance of detected ions against their mass-to-charge ratios. These spectra serve as fingerprints for identifying chemicals and determining their concentrations in a given sample.
Historical Context
The history of mass spectrometry can be traced back to the early 20th century, with key advancements shaping its evolution. The initial development emerged in the 1910s when J.J. Thomson created the first mass spectrometer, marking the convergence of physics and chemistry.
In the subsequent decades, important refinements were made. In 1946, the introduction of the first significant commercial mass spectrometers occurred, facilitating broader use in laboratories. The 1980s heralded a new era with the advent of soft ionization techniques, such as Electrospray Ionization, that expanded mass spectrometry’s applicability to larger biomolecules, including proteins and nucleic acids.
Today, mass spectrometry is a staple in modern laboratories, thanks to continuous advancements and innovations. This historical trajectory reflects not only technological improvements but also a growing understanding of its capabilities across various scientific domains.
Fundamental Principles of Mass Spectrometry
Understanding the fundamental principles of mass spectrometry is essential for grasping how this analytical technique operates. The foundation of mass spectrometry is based on the behavior of charged particles in an electric and magnetic field. This knowledge helps in developing methods for identifying and quantifying compounds. Among the key aspects are ionization techniques, mass analysis, and detection methods. Each of these elements plays a critical role in generating reliable data from mass spectrometry analyses.
Ionization Techniques
Ionization is the first step in mass spectrometry and it determines the type of ions generated from the sample. There are several techniques, each with its own strengths and weaknesses. Customizing the ionization method according to the sample type can significantly improve analysis outcomes.
Electron Ionization
Electron ionization is a widely used technique in mass spectrometry. It is renowned for producing well-defined spectra that facilitate the identification of compounds. One of the key characteristics of electron ionization is that it involves bombarding gas-phase molecules with electrons to create ions. This method is particularly beneficial when working with volatile compounds.
However, the unique feature of electron ionization is its tendency to fragment molecules extensively, which can serve both as an advantage and disadvantage. While fragmentation aids in structural elucidation, it can complicate the interpretation of the data, requiring expertise in understanding the resulting spectrum.
Chemical Ionization
Chemical ionization provides a softer ionization technique compared to electron ionization. In this method, ions are formed by chemical reactions between reagent gases and analyte molecules rather than direct electron bombardment. This process generally results in less fragmentation due to the milder conditions used.
The key characteristic of chemical ionization makes it a popular choice when analyzing large biological molecules where retaining the molecular structure is crucial. However, it is essential to consider that its reliance on specific reagents can limit versatility compared to more universal methods.
Electrospray Ionization
Electrospray ionization is especially suitable for analyzing polar compounds and biomolecules. This technique employs a high electric field to create intact ions from liquid samples, generating ions in a solution. Its ability to produce multiple charging states is particularly advantageous for large molecules like proteins.
However, the disadvantage here is the need for careful control of experimental conditions. Changes in the solvent or flow rate can alter the ionization efficiency significantly, leading to variability in results.
MALDI (Matrix-Assisted Laser Desorption/Ionization)
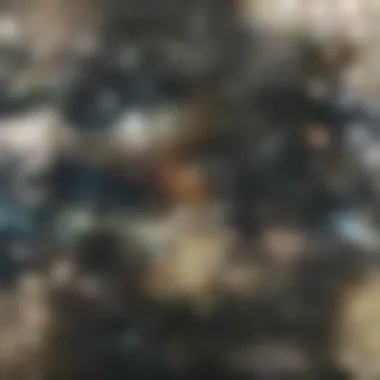
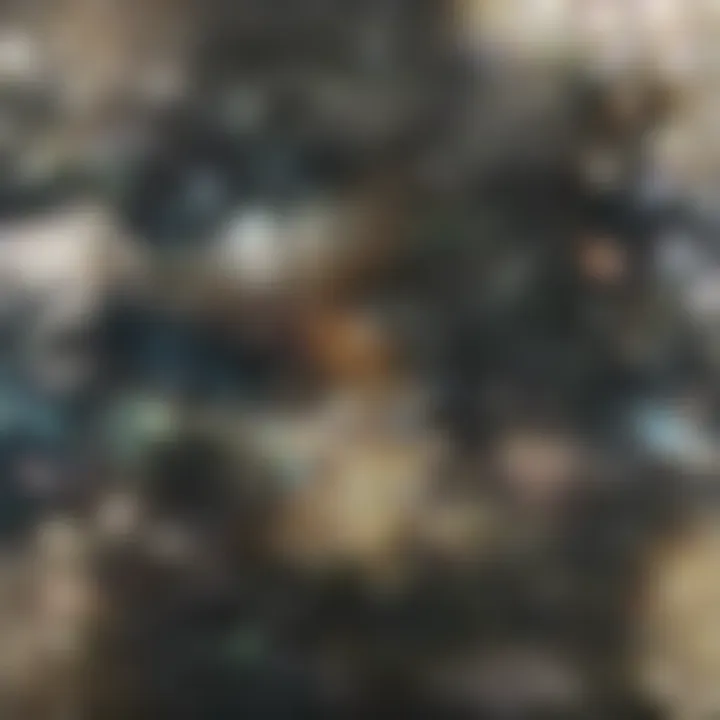
MALDI is another powerful ionization method particularly useful for analyzing large biomolecules like peptides and proteins. In this technique, the sample is mixed with a matrix material and irradiated with a laser. The matrix absorbs the laser energy, aiding in desorbing and ionizing the sample.
The key characteristic of MALDI is its ability to analyze samples with minimal fragmentation, thereby preserving the integrity of large molecules. However, one downside is that the ionization process can be influenced by the choice of matrix, which may necessitate trial and error during method development.
Mass Analysis
Mass analysis is where the mass-to-charge ratio of generated ions is determined. Various types of analyzers offer unique benefits, allowing for the optimization of this step based on specific requirements for analysis.
Quadrupole Analyzers
Quadrupole analyzers are among the most common mass analyzers in use today. They utilize four parallel rods to filter ions based on their mass-to-charge ratio. A significant reason for their popularity is their robustness and ability to operate in multiple modes such as scanning and selected reaction monitoring.
However, their limited resolution compared to advanced analyzers can be a downside in applications requiring precise measurements.
Time-of-Flight Analyzers
Time-of-flight (TOF) analyzers measure the time taken by ions to travel a specified distance. This speed directly correlates with their mass-to-charge ratio. TOF analyzers are favored for their rapid analysis capabilities and high mass resolution, which is an advantage in various research applications.
The disadvantage of TOF analyzers is their sensitivity to the initial energy distributions of the ions, which can lead to variability in the results without stringent control of experimental conditions.
Orbitrap Analyzers
Orbitrap analyzers are sophisticated instruments that can achieve high-resolution measurements, providing detailed insights into complex samples. They trap ions in an electrostatic field and then measure their oscillation frequency to determine mass.
The key characteristic that sets Orbitrap analyzers apart is their ability to analyze a wide mass range with high resolution in a compact format. However, the potential for high costs and technical complexity are disadvantages to consider when selecting this type of analyzer.
FT-ICR (Fourier Transform Ion Cyclotron Resonance)
FT-ICR is known for its exceptional mass resolution and accuracy. It utilizes a strong magnetic field to trap ions and measures their cyclotron frequencies, translating this information into mass spectra. This method is extremely beneficial in detailed molecular characterization.
However, the need for large magnets and complex instrumentation makes FT-ICR less accessible and can complicate routine analyses.
Detection of Ions
The detection stage is crucial for quantifying and observing ions after mass analysis. Different detection methods offer unique advantages, impacting the overall efficiency of mass spectrometry.
Electron Multiplier
Electron multipliers are known for their sensitivity in detecting low-abundance ions. They work by amplifying signal current from each ion, making them particularly useful in applications requiring detection of trace components.
However, the drawback of this method lies in the potential for non-linearity at high ion currents, which means calibration is essential for accurate quantification.
Microchannel Plate
Microchannel plates enable detection of ions over a wide range of masses and are often used in combination with other systems. Their high temporal and spatial resolution adds value to many applications requiring rapid detection.
Despite their advantages, microchannel plates may present challenges regarding ion counting accuracy in very high-speed applications due to potential saturation effects.
Faraday Cup
The Faraday cup represents a robust and reliable approach for ion detection. It measures the total current produced when ions strike the cup surface. This method is especially effective for high-ion currents and has long operational lifespans.
The main disadvantage of Faraday cups is their inability to provide detailed information about individual ions, which might be needed in comprehensive analyses.
Types of Mass Spectrometry
Understanding the types of mass spectrometry is crucial for grasping the analytical capabilities of this technique. Each type serves unique purposes in various scientific applications, which highlights its versatility and relevance. The different modalities of mass spectrometry can be tailored to address specific analytical needs, enhancing both sensitivity and specificity.
Single Mass Spectrometry
Single mass spectrometry is often seen as the foundational technique in mass spectrometry analysis. It involves a straightforward process where ions generated from a sample are introduced into the mass analyzer. Here, the mass-to-charge ratio of ions is measured, providing essential information about the sample composition.
This approach is particularly valuable for applications that require rapid identification of compounds, such as in the analysis of drugs or metabolites. The benefits include:
- Simplicity in method development and execution.
- Quick results, making it suitable for high-throughput environments.
- Effective for the detection of known compounds, assuming no interference from other substances.
However, one must consider its limitations as well. Single mass spectrometry may not efficiently distinguish between closely related compounds that have similar mass-to-charge ratios. Therefore, this method often serves as a preliminary step that leads to more complex analyses.
Tandem Mass Spectrometry (MS/MS)
Tandem mass spectrometry, commonly referred to as MS/MS, provides a more intricate approach to mass spectrometric analysis. This technique involves two stages of mass analysis, which allows for significant enhancements in both sensitivity and specificity. After the initial ion generation, selected ions are fragmented in a collision cell, producing daughter ions that are subsequently analyzed for their mass.
The advantages of MS/MS include:
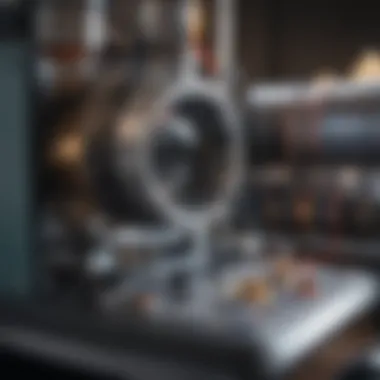
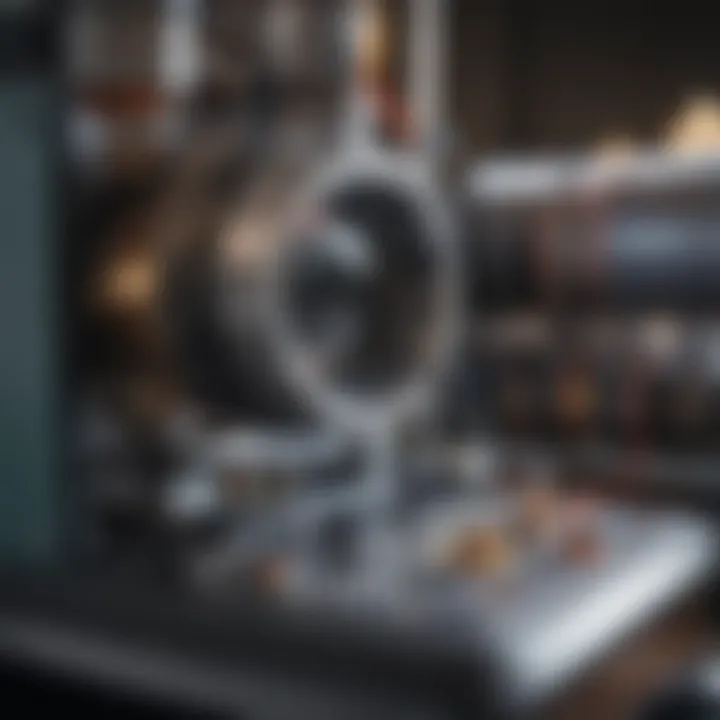
- Improved ability to identify unknown compounds by providing structural information from fragmentation patterns.
- Increased sensitivity, making it particularly suitable for trace analysis in complex biological matrices.
- Capability to analyze isomers that may not be resolved in single mass spectrometry.
Due to these advantages, MS/MS is widely applied in diverse fields such as proteomics, metabolomics, and pharmacokinetics. The method’s complexity, however, requires careful optimization and a deep understanding of the mass spectrometer’s operation.
High-Resolution Mass Spectrometry
High-resolution mass spectrometry (HRMS) is an advanced technique that offers exceptional accuracy in measuring mass-to-charge ratios. This technique utilizes sophisticated instrumentation capable of resolving very closely related masses, making it an indispensable tool in analytical chemistry.
The critical features of HRMS include:
- Enhanced Mass Accuracy: It can measure the mass of ions to several decimal places, allowing for better formula determination of analytes.
- Isomer Differentiation: High-resolution capabilities enable distinguishing between isomers that would typically be indistinguishable in conventional mass spectrometry.
- Complex Mixture Analysis: HRMS is proficient at analyzing samples with highly complex matrices, such as environmental or biological samples containing numerous compounds.
The trade-offs include higher costs and longer analysis times compared to simpler methods. Despite these challenges, the precision and reliability of HRMS make it a powerful tool in research and quality control across multiple industries.
Applications of Mass Spectrometry
Mass spectrometry plays a crucial role across various sectors due to its precision and versatility. In this section, we explore the numerous applications of mass spectrometry, delving into its significance in distinct fields. Each application highlights the potential of this analytical technique, showcasing its benefits and considerations, helping to delineate its indispensable presence in modern science.
Pharmaceutical Analysis
The pharmaceutical industry heavily relies on mass spectrometry for drug development and quality control. It aids in identifying chemical compounds and monitoring the purity of substances. Mass spectrometry provides rapid and reliable results, which are essential for regulatory submissions. Moreover, it assists in pharmacokinetics by studying how drugs disperse in the body.
Some critical aspects include:
- Drug Metabolism: Understanding how the body breaks down medications.
- Drug Level Monitoring: Ensuring therapeutic levels in patient samples.
- Stability Testing: Evaluating how drugs degrade over time.
Select instruments, like the Waters Xevo and SCIEX Triple Quad, are widely recognized in pharmaceutical analysis, enhancing the efficiency of workflows.
Environmental Monitoring
Mass spectrometry is instrumental in environmental science, providing insights into pollutant levels in air, water, and soil. Its high sensitivity allows detection of trace chemicals, proving necessary for compliance with environmental regulations.
Key utilities include:
- Detection of Contaminants: Identifying harmful substances like heavy metals and pesticides.
- Air Quality Assessment: Analyzing atmospheric pollutants.
- Water Safety: Profiling toxic chemicals in drinking water.
Tools such as the Agilent 7200 and Thermo Scientific Q Exactive can effectively address environmental concerns, making this technique valuable for both researchers and regulatory bodies.
Proteomics and Metabolomics
In biological research, mass spectrometry excels in proteomics and metabolomics, allowing scientists to quantify proteins and metabolites in complex biological samples. This application is crucial for understanding biomolecular interactions and metabolic pathways, which can lead to potential therapeutic targets.
Essentials of this application include:
- Protein Identification: Discovering abundance and modifications.
- Metabolite Profiling: Examining metabolic changes under different conditions.
- Biomarker Discovery: Identifying disease markers for early diagnosis.
Mass spectrometry platforms, such as the Bruker timsTOF and AB Sciex 5600, serve as fundamental tools in this realm, streamlining workflows and enhancing data quality.
Forensic Science
Forensic science finds mass spectrometry crucial for analyzing physical evidence. It helps in drug identification, toxicology reports, and even in the investigation of criminal cases. Mass spectrometry offers rapid, reliable, and reproducible results, aiding law enforcement in solving cases effectively.
In forensic applications, it is utilized to:
- Identify Drugs: Determining substances found at crime scenes.
- Analyze Biological Samples: Testing blood or urine for toxins or drugs.
- Trace Evidence Analysis: Collecting and identifying minute sample traces.
Instruments like the Agilent 5977 and Thermo Scientific TSQ can contribute significantly to forensic investigations, highlighting the intersection between mass spectrometry and legal processes.
In summary, mass spectrometry's diverse applications underline its importance across various scientific domains. By enhancing analytical capabilities, it promotes advancements in research and regulatory compliance, paving the path for innovative solutions.
Emerging Trends and Innovations
Emerging trends and innovations in mass spectrometry hold significant value in refining its application across various scientific fields. As research demands grow more complex, the evolution of mass spectrometry continues to address these challenges. The integration of new techniques, the miniaturization of equipment, and advancements in ionization methods contribute greatly to enhancing the effectiveness and versatility of this analytical tool. Understanding these innovations helps researchers adapt to the changing landscape of science.
Integration with Chromatography
LC-MS
Liquid Chromatography-Mass Spectrometry (LC-MS) emerges as a crucial method in the realm of analytical chemistry. This combination offers an effective way to separate, identify, and quantify substances. The key characteristic of LC-MS is its ability to handle complex mixtures with high sensitivity and specificity. It is especially useful in pharmaceutical analysis, where precise measurement of compounds is essential.
One unique feature of LC-MS is its dual functionality. The liquid chromatography component concentrates the analytes before mass spectrometry analysis. This enhances detection limits and facilitates the analysis of compounds present in low concentrations, a common scenario in drug development. In this article, its benefits include high throughput and capability to analyze a wide range of molecules. Nevertheless, the main disadvantage is the cost of equipment and the requirement for skilled personnel, which can limit its accessibility to some laboratories.
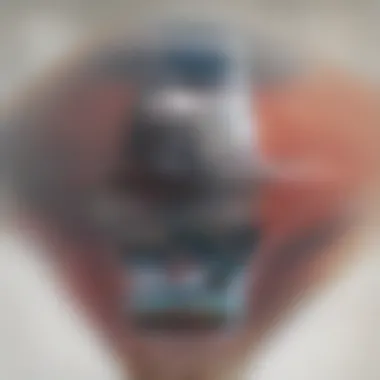
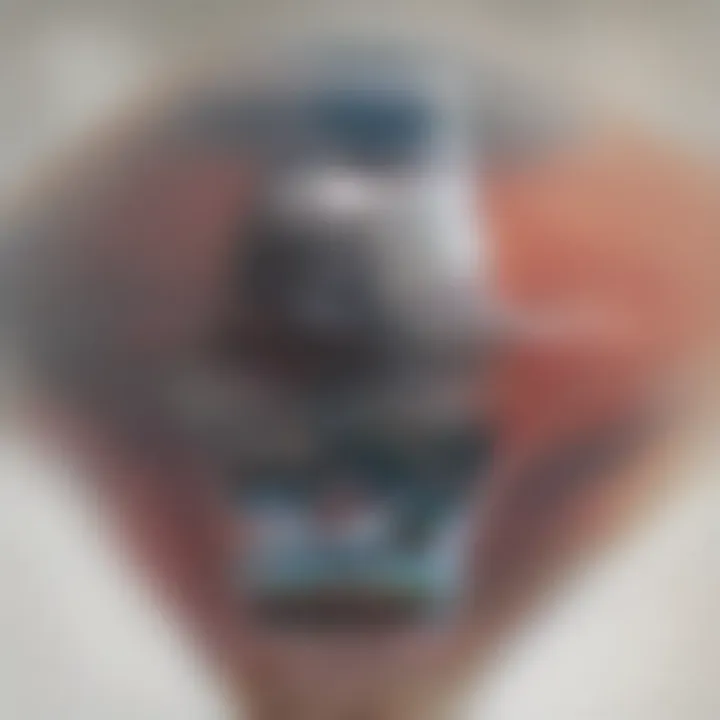
GC-MS
Gas Chromatography-Mass Spectrometry (GC-MS) serves as another vital method in mass spectrometry applications. This technique is particularly suited for volatile and semi-volatile substances, playing a significant role in environmental monitoring and forensic science. The key characteristic of GC-MS is its high resolution, allowing for the detection and quantification of trace elements in complex matrices.
A distinct aspect of GC-MS lies in its sample preparation process, as samples must be vaporized before reaching the mass spectrometer. This prepares samples effectively for analysis. The advantages of GC-MS include its sensitivity and ability to analyze large volumes of samples. However, its limitation includes the necessity of volatile substances, making it less effective for non-volatile components. Recognizing these factors is crucial in selecting the appropriate analytical method for specific research needs.
Miniaturization and Portability
The trend towards miniaturization and portability is reshaping mass spectrometry devices. Miniaturized devices allow for easier handling, reduced costs, and improved accessibility in fieldwork scenarios. These portable mass spectrometers can operate in real-time, enabling immediate data collection and analysis in locations where traditional lab setups are not feasible. The benefits of miniaturization include enhanced mobility, allowing researchers to deploy instruments directly within various environments, from clinical settings to environmental fields.
However, challenges such as limited analytical performance and sensitivity compared to larger counterparts still remain. Researchers continue to explore ways to overcome these limitations, furthering the trend of compact and efficient instrumentation.
Advancements in Ionization Methods
The development of novel ionization methods plays a pertinent role in the advancement of mass spectrometry. Techniques like Desorption Electrospray Ionization (DESI) and Ambient Ionization offer fresh approaches to sample handling. The key benefit is the ability to analyze samples in their native environments, thus preserving chemical composition and structure.
These advancements provide significant improvements over traditional methods, which often require sample preparation that can alter results. Consequently, the unique capabilities of these new ionization methods enhance the overall accuracy and reliability of data acquisition in mass spectrometry applications. Researchers are increasingly utilizing these techniques, validating their use across a variety of disciplines.
Emerging trends in mass spectrometry signify a shift towards more accessible, efficient, and versatile analytical techniques. By staying informed about these developments, researchers can harness the potential of mass spectrometry to address modern challenges in various fields.
Challenges in Mass Spectrometry
Mass spectrometry, while a sophisticated and powerful analytical technique, faces various challenges that can impact its effectiveness. Understanding these challenges is essential for researchers and practitioners in the field. These obstacles often stem from the complexity of the sample preparation process, the influence of matrix effects, and the intricacies involved in data interpretation. Addressing these issues not only enhances the accuracy of the mass spectrometry results but also broadens its applications across different scientific domains.
Sample Preparation Complexity
Sample preparation stands as one of the most intricate steps in mass spectrometry. The complexity arises from the need to ensure that samples are adequately prepared before analysis. The myriad of substances in biological samples, for example, can necessitate extensive purification or concentration processes. This can include techniques such as solid-phase extraction or liquid-liquid extraction to isolate the target compounds.
The difficulty with sample preparation is often magnified when dealing with complex matrices, where the presence of co-eluting compounds can interfere with the analysis. Variability in sample preparation protocols can lead to inconsistencies in results. Therefore, developing a standardized preparation method is crucial to enhance reproducibility and reliability in analytical results.
Matrix Effects
Matrix effects refer to the influence of co-existing compounds in a sample on the analysis of the target analyte. Such effects can alter ionization efficiency, leading to inaccurate quantification of the analytes. For instance, in biological samples, the presence of proteins, salts, and other constituents can suppress or enhance the ionization of the target molecules. This leads to signal variations that are not reflective of the actual concentration of the target analyte.
Control measures, such as using internal standards, can help mitigate matrix effects. However, full elimination of these issues is often unfeasible. Awareness and understanding of matrix effects are vital in ensuring that mass spectrometry results are both valid and representative.
Data Interpretation Challenges
Interpreting mass spectrometry data poses another considerable challenge. The vast amount of data generated can be overwhelming. Analyzing the spectra requires a thorough understanding of the peaks, their corresponding masses, and the chemical identity of the analytes. This is particularly challenging when dealing with unknown compounds, where deconvoluting the data becomes more complicated.
Furthermore, the integration of mass spectrometry with other analytical techniques can enhance data complexity. The various software tools available can assist in data processing, but selecting the correct parameters for peak identification often requires expert knowledge. As a result, training and expertise play an essential role in achieving accurate data interpretation.
"Addressing these challenges is key to maximizing the effectiveness of mass spectrometry in research and applications."
In summary, the challenges associated with mass spectrometry, particularly in sample preparation complexity, matrix effects, and data interpretation, must not be overlooked. As advancements continue to unfold in mass spectrometry, an understanding of these challenges remains paramount. Researchers must continuously evolve their strategies to overcome these obstacles for improved analytical accuracy.
Future Directions in Mass Spectrometry
The future of mass spectrometry promises to advance in several significant areas. Understanding these potential directions is crucial for researchers and professionals who rely on mass spectrometry for their work. The evolving nature of technology and research initiatives will drive innovations, impacting fields such as biotechnology, pharmaceuticals, and environmental science.
Next-Generation Technologies
Next-generation technologies are set to redefine how mass spectrometry operates. Improvements in instrumentation and software will enhance sensitivity and resolution. For instance, innovations in ionization techniques may allow for the analysis of extremely low concentrations of substances. These advancements can lead to more effective detection of biomarkers in clinical settings, aiding in disease diagnosis and monitoring.
Moreover, the integration of machine learning algorithms will streamline data analysis. As data complexity increases, automated interpretation can provide quicker, more reliable results. Researchers can expect mass spectrometry to incorporate high-throughput systems, allowing simultaneous analysis of multiple samples with minimal user intervention.
Applications in Personalized Medicine
In the context of personalized medicine, mass spectrometry could play a vital role in tailoring treatments to individual patients. By identifying unique metabolic profiles, clinicians can understand how different patients respond to drugs. This specificity can improve the effectiveness of treatments while minimizing adverse effects.
Additionally, mass spectrometry can assist in pharmacogenomics. By analyzing genetic variations that affect drug metabolism, healthcare providers can predict which medications will work best for a particular patient. This approach requires detailed understanding of metabolic pathways, which mass spectrometry can elucidate through precise chemical analysis. As personalized medicine continues to grow, the demand for mass spectrometry will likely increase, making its advancements even more significant.
Collaborative Research Initiatives
Collaboration among researchers is essential for pushing mass spectrometry into new frontiers. Interdisciplinary teams that combine expertise from chemistry, biology, medicine, and engineering can foster innovation. Joint research initiatives can expedite the development of novel applications and integration of new technologies, ultimately enhancing the reliability of mass spectrometry in various fields.
For example, partnerships between academic institutions and industry can lead to more comprehensive studies. Sharing resources and knowledge enables faster progress in understanding complex biological systems. Collaborative efforts may also pave the way for standardization of protocols, ensuring consistency in mass spectrometry results across laboratories. Such initiatives will help in establishing mass spectrometry as a cornerstone technique in both research and clinical diagnostics.
The End
Summary of Key Points
- Mass spectrometry involves key processes such as ionization, mass analysis, and ion detection.
- Different ionization techniques, like Electrospray Ionization and MALDI, cater to various sample types.
- The choice of mass analyzer can significantly affect the resolution and accuracy of measurements, with options like Quadrupole and Orbitrap available.
- Applications span across pharmaceutical analysis, environmental monitoring, proteomics, and forensic science, demonstrating its versatility.
- Emerging trends, such as miniaturization, greatly enhance usability and accessibility in diverse settings.
- Challenges in sample preparation and data interpretation persist, yet innovations continue to address these issues.
- The future of mass spectrometry holds immense promise, especially in personalized medicine and collaborative research efforts.
The Importance of Mass Spectrometry in Modern Science
Mass spectrometry is an indispensable tool in today's scientific landscape. Its ability to provide detailed molecular insights makes it crucial for various fields, including biochemistry and pharmacology. As new technologies emerge, mass spectrometry expands its reach, offering solutions to complex analytical problems. This relevance is evident not only in research environments but also in practical applications like drug development and environmental safety assessments.
Mass spectrometry not only enhances our understanding of molecular structures but also drives forward the capabilities of modern analytics. Its contributions to scientific advancement are profound, ultimately improving human health and safety. Therefore, continued exploration of mass spectrometry will ensure it remains pivotal in innovative research and real-world applications.