Analytical Ultracentrifugation Service Overview
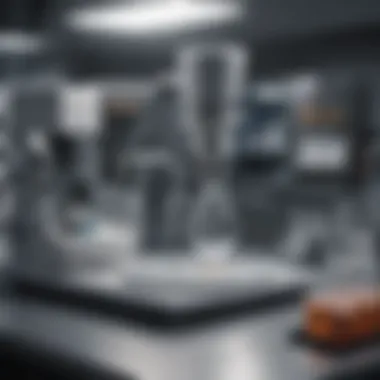
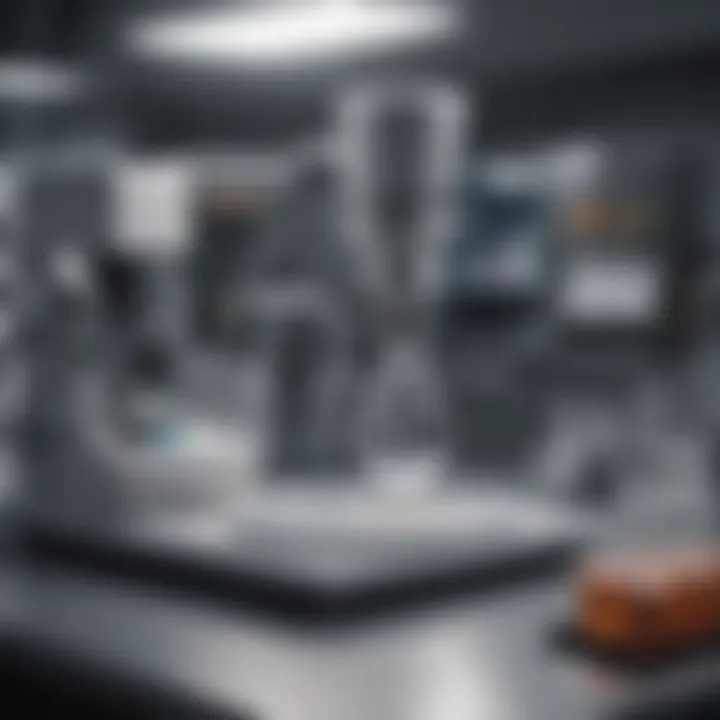
Intro
In the study of macromolecules and nanoparticles, understanding their properties is essential for many fields, including biochemistry, materials science, and pharmaceuticals. Analytical ultracentrifugation (AUC) stands out as a pivotal technique in the characterization process. This method enables researchers to analyze the size, shape, and molecular weight of complex samples with precision. The integration of AUC services is vital for both academic and industrial laboratories aiming for rigorous experimental protocols and accurate results.
Analytical ultracentrifugation provides a means to not only separate but also quantify different components in a mixture. As such, the service has gained traction due to its versatility and effectiveness compared to other analytical tools. Understanding how AUC works, its applications, and its limitations can empower researchers to utilize this technique effectively.
Research Background
Overview of the scientific problem addressed
The characterization of macromolecules, including proteins, nucleic acids, and polysaccharides, presents challenges due to their size and complexity. Conventional methods like gel electrophoresis and chromatography often fall short when it comes to accurately determining molecular weights and interactions in solution. Analytical ultracentrifugation fills this gap by offering detailed insights into the behavior of these substances under centrifugal forces. The technique allows scientists to assess the sedimentation properties of biomolecules, thus revealing important information about their structure and interactions.
Historical context and previous studies
AUC has a storied history, dating back to the early 20th century when the first analytical centrifuges were developed. Over the decades, numerous studies have advanced our understanding of this technique. For example, the work of F. A. Lind and H. E. L. Svedberg in the 1920s laid the foundation for modern analytical ultracentrifugation. They explored sedimentation rates and provided insight into the behavior of colloidal systems, thus expanding the application of ultracentrifugation beyond simple separations.
Research has further advanced with the advent of digital data acquisition and sophisticated software tools that facilitate the analysis of AUC data. Recent studies highlight its application in characterizing protein-protein interactions, assessing nanoparticle stability, and optimizing formulations in drug development. These advancements continue to make AUC a relevant and essential method in today's scientific landscape.
"The evolution of analytical ultracentrifugation has enabled a deeper understanding of molecular interactions that are critical for designing effective biotherapeutics."
By exploring the methodology, benefits, and challenges associated with AUC, we can better appreciate its role in enhancing research quality and outcomes across various scientific disciplines.
Intro to Analytical Ultracentrifugation
Analytical ultracentrifugation (AUC) is a significant analytical technique that offers precision in the characterization of macromolecules and nanoparticles. This method provides a means to investigate the size, shape, and interactions of various biopolymers and synthetic materials in a solution. Understanding AUC is essential for researchers because it serves as a cornerstone in biochemistry, molecular biology, and material sciences. From studying protein structures to examining drug delivery systems, the applications of AUC are numerous and varied.
Definition and Purpose
Analytical ultracentrifugation is a technique that utilizes high rotational speeds to create a centrifugal field within a solution. This centrifugal force causes the molecules to separate based on their sedimentation coefficients, which are determined by their size, shape, and density. The primary purpose of AUC is to quantify the properties of macromolecules, allowing scientists to obtain vital information about their composition and behavior under various conditions. This capability makes AUC an invaluable tool in both research and industrial settings.
Historical Background
The foundations of analytical ultracentrifugation date back to the early 20th century. The first ultracentrifuge was developed by Theodor Svedberg, a Swedish chemist, who won the Nobel Prize for his work in 1926. Svedberg's pioneering efforts established the principles of sedimentation and led to the development of the analytical ultracentrifuge as a robust instrument for molecular analysis. Over the decades, advancements in technology have refined the method, enabling more accurate measurements and broader applications.
Relevance in Modern Science
In contemporary scientific research, the relevance of analytical ultracentrifugation cannot be overstated. It is a preferred method for studying complex biomolecules such as proteins, nucleic acids, and lipids. Researchers utilize AUC to explore vital aspects of these biomolecules, including their molecular weight, structure, and interactions. Additionally, AUC has gained traction in nanoparticle research, particularly for evaluating drug delivery systems and nanomaterials' stability. The ability to assess these parameters with high resolution underscores AUC's importance in both academic research and industrial innovation.
Principles of Analytical Ultracentrifugation
Understanding the principles of analytical ultracentrifugation is essential for grasping how this technique provides valuable insights into biomolecular properties. At its core, analytical ultracentrifugation employs centrifugal forces to separate particles based on their size, shape, and density. This method is pivotal in a range of scientific fields, including biochemistry and material science. We will now delve deeper into the fundamental mechanisms and influencing factors that affect the outcomes of this analytical approach.
Basic Mechanisms
Analytical ultracentrifugation operates primarily on the principles of sedimentation. When subjected to high rotational speeds, particles within a sample experience forces that drive them towards the bottom of the centrifuge tube. The sedimentation velocity is influenced by the mass and shape of the particles and the viscosity of the surrounding liquid.
During centrifugation, two primary forces act on the particle: the centrifugal force, which pushes the particle outward, and the gravitational force attempting to hold it back. The sedimentation coefficient, a critical parameter, provides insight into the particle's behavior under centrifugal force. It is essential for determining the particle's size and shape accurately.
Additionally, the analysis of the sedimentation profile generated during the experiment allows for the evaluation of particle interactions, density distribution, and concentration gradients, enhancing our understanding of the sample presented.
Key Variables Affecting Results
Several variables influence the results of analytical ultracentrifugation, and understanding these is crucial for obtaining reliable data. Key factors include:
- Rotational Speed: The speed at which the rotor spins can significantly affect sedimentation rates and profiles. Higher speeds generally increase the resolution of differentiation between particles.
- Temperature: Temperature control during the experiment is vital. Variations can lead to changes in viscosity and solubility, potentially impacting sedimentation behavior.
- Buffer Composition: The choice of buffer affects the ionic strength and pH, which are critical in determining the stability and interaction of biomolecules. An optimally chosen buffer can enhance resolution.
- Sample Concentration: The concentration of particles can lead to non-ideal behaviors that complicate interpretations. Overly concentrated samples may exhibit interactions that modify sedimentation rates.
Being aware of these factors helps researchers design more effective experiments and analyze data with greater accuracy. By controlling these variables, one can optimize conditions, thereby yielding more precise and informative results level.
Remember that the intricacies of the sedimentation process require careful consideration of experimental parameters to ensure reliable outcomes.
Instrumentation and Technology
Instrumentation and technology are fundamental pillars in the realm of analytical ultracentrifugation. The efficacy of this technique relies on the precision and reliability of the instruments utilized. High-quality instrumentation allows for accurate measurement and analysis of macromolecules and nanoparticles. The technology employed in ultracentrifugation ensures that data obtained is both reproducible and intelligible, which is crucial for effective scientific communication and research advancements.
Components of an Ultracentrifuge
An ultracentrifuge is composed of several essential components that work together to achieve high-speed separation of samples:
- Rotor: This is the spinning element that holds the sample in place. Rotors can vary greatly, often made from aluminum or titanium to withstand high g-forces.
- Drive System: This powers the rotor, enabling it to reach the required speeds necessary for sedimentation processes. The drive can be either direct drive or belt-driven.
- Sample Cells or Tubes: These hold the samples and must be carefully chosen based on the intended analysis to ensure accurate results.
- Temperature Control Systems: Maintaining the correct temperature during centrifugation is vital, as temperature fluctuations can affect sample behavior and data reliability.
- Detection System: This can involve optical systems for absorbance or interference measurement and is crucial for monitoring the sedimentation and analyzing the resulting data.
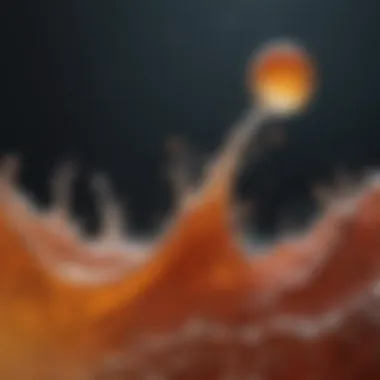
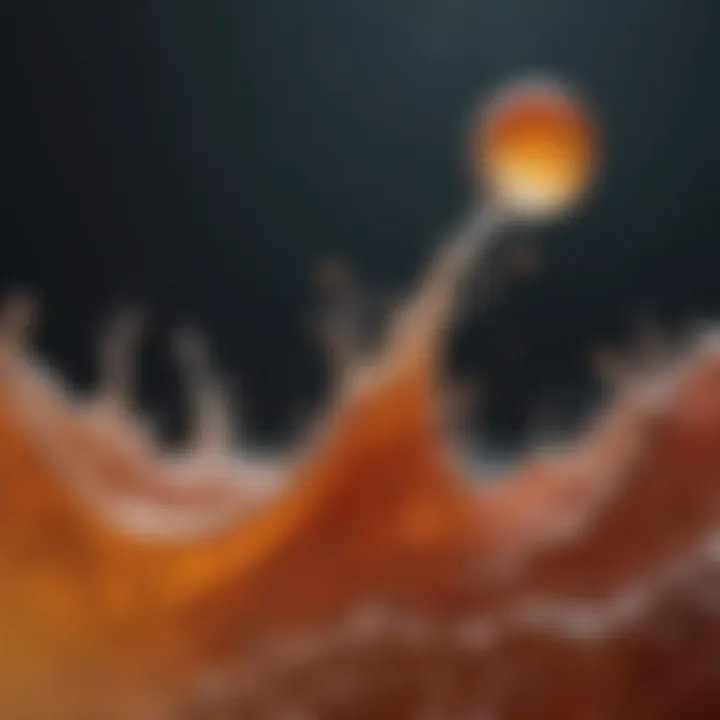
Understanding the specific roles of these components helps researchers optimize their use of ultracentrifugation techniques.
Types of Ultracentrifuges
Different types of ultracentrifuges serve specific purposes, catering to diverse research needs. Generally, they can be classified into two main categories:
- Analytical Ultracentrifuges: These are equipped with advanced detection systems, allowing for real-time data acquisition while separating components in a sample. They provide critical insights into molecular weight, distribution, and interactions among biopolymers.
- Preparative Ultracentrifuges: These focus on separating components for collection rather than detailed measurements. Preparative systems are typically larger and designed for high-throughput applications, such as the isolation of proteins or nucleic acids for further study.
Specialized Ultracentrifuges
In addition to the main types, several specialized versions exist to address specific analytical challenges:
- Swinging-Bucket Rotors: These allow sample tubes to pivot, enhancing sedimentation efficiency for larger particles.
- Fixed-Angle Rotors: These maintain a constant angle to the axis of rotation, which is favorable for sedimenting a wide range of sample sizes efficiently.
Advancements in Analytical Technology
The field of analytical ultracentrifugation is continually evolving, driven by technological advancements. Key areas of progress include:
- Enhanced Detection Techniques: Modern ultracentrifuges now feature more sensitive detection methods, such as multi-wavelength detection for detailed analysis of complex samples, improving data accuracy and interpretability.
- Data Analysis Software: Improved computational tools assist researchers in interpreting the complex data generated from ultracentrifuge experiments, enhancing the understanding of molecular interactions and behaviors.
- Automation: Some ultracentrifuge systems are becoming increasingly automated, minimizing human error and standardizing processes, resulting in more reliable outcomes and an increase in throughput.
Incorporating these advancements into research practices not only improves accuracy but also enhances the practical application of analytical ultracentrifugation in various scientific fields.
Preparation for Analytical Ultracentrifugation
Preparation for analytical ultracentrifugation is a critical phase. Proper preparation can significantly influence the accuracy and reliability of the results. Each of the aspects discussed in this section plays a key role in ensuring successful experiments. Understanding these factors can not only enhance data quality but also reduce potential errors that may arise during experimentation.
Sample Requirements
The nature of the sample is fundamental in analytical ultracentrifugation. First, each sample must be homogenous and free from contaminants. Impurities can scatter light or cause unexpected sedimentation behaviors. Thus, it is crucial to purify samples adequately.
Additionally, concentration is vital. Generally, samples should be at a concentration that allows for adequate detection but not so concentrated that it causes aggregation. For instance, many proteins are analyzed at concentrations ranging from 0.1 mg/mL to several mg/mL. This concentration ensures that the appropriate sedimentation velocity can be measured without interference. However, the optimal concentration can vary based on the type of analysis.
Finally, researchers should consider the volume of the sample. Typically, a minimum of 100 µL is required for analytical ultracentrifuges. Ensuring correct volume is necessary for effective operation and to allow for adequate data representation.
Buffer Conditions
Buffer conditions can profoundly affect the sedimentation behavior of samples. Hence, it is essential to select the right buffer that maintains the structural integrity of the macromolecules. First and foremost, the pH of the buffer should be relevant to the molecule of interest. Alterations in pH can lead to denaturation or aggregation.
Buffer ionic strength also needs consideration. High ionic strength can shield charges on proteins, affecting their sedimentation rates. Conversely, low ionic strength may result in increased electrostatic repulsion. Therefore, a balance must be struck that reflects physiological conditions or experimental requirements.
Temperature plays another important role in buffer conditions. Most experiments are conducted at a controlled temperature, usually between 4°C and 25°C. Maintaining this temperature prevents heat-induced denaturation, ensuring that the results are representative of the original sample biochemistry.
Handling and Storage
Proper handling and storage of samples before ultracentrifugation are critical for preserving their properties. For example, samples should be stored at appropriate temperatures. Many biomolecules are sensitive to degradation, which can occur when left at room temperature. Therefore, refrigerated storage is recommended for most protein or nucleic acid samples.
Minimizing exposure to air is another precaution. Oxygen can cause oxidation, altering the properties of the sample. Use of an inert atmosphere or sealing containers is advisable, especially for sensitive materials.
Besides storage, handling techniques must minimize shear forces. This is particularly necessary when pipetting samples. Careful pipetting reduces the risk of foaming and mechanical degradation.
Methodology of Analytical Ultracentrifugation
The methodology of analytical ultracentrifugation is a fundamental component of understanding this powerful technique. It involves a series of systematic procedures that ensure accurate results, making it a vital part of the analytical ultracentrifugation service. This section will delve into experimental design, data collection techniques, and analysis methods. Each of these components is critical for optimizing the outcomes of ultracentrifugation experiments.
Experimental Design
Experimental design in analytical ultracentrifugation begins with defining the objectives of the study. It is crucial to clearly outline what is to be measured, such as molecular weight, shape, or interaction dynamics. Key elements of this stage include:
- Selection of Samples: Choosing appropriate samples is essential. The sample should be pure and representative of the target substance.
- Concentration Considerations: Optimal sample concentration is vital for effective sedimentation and accurate results. Overly concentrated samples can lead to artifacts and misleading data.
- Validation of Buffer: The buffer solutions must be compatible with the analytical objectives. This affects the solubility and stability of the macromolecules.
This thoughtful approach will set the groundwork for reliable experimentation.
Data Collection Techniques
Data collection in analytical ultracentrifugation is done through two primary techniques: sedimentation velocity and sedimentation equilibrium. Each one offers unique insights into the sample under study.
- Sedimentation Velocity measures the rate at which particles move under centrifugal force. Sensors in the ultracentrifuge detect changes in absorbance or interference patterns, generating distribution data over time.
- Sedimentation Equilibrium reaches a balance where sedimentation and diffusion are equal. This provides a steady-state view that is effective for determining molecular-weight distributions and interactions.
The choice of technique depends on the specific aim of the study, which influences the type of data collected and analyzed.
Analysis of Results
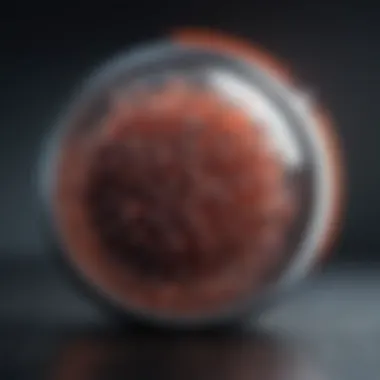
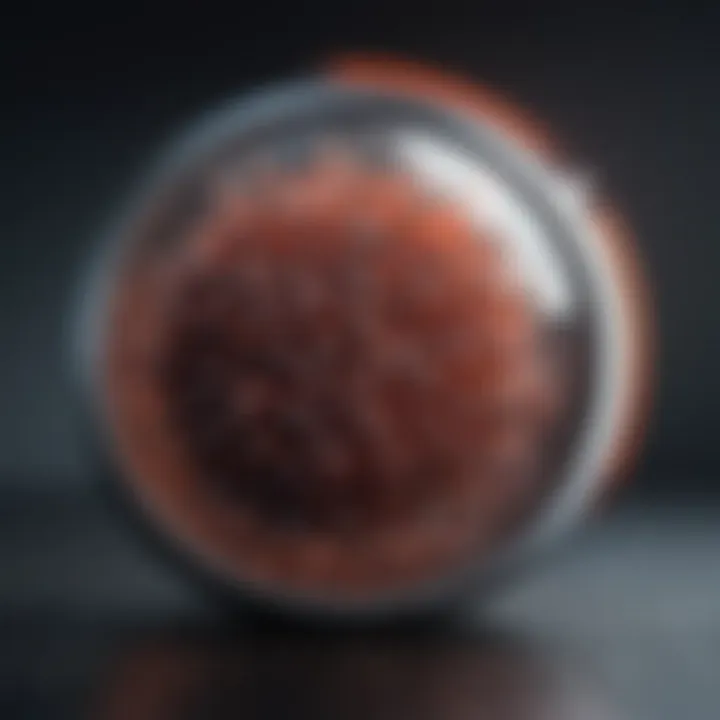
Interpreting the results from analytical ultracentrifugation is a sophisticated endeavor. Analyzing sedimentation data requires expertise in both theoretical models and computational techniques. Important steps in this phase include:
- Model Selection: A proper model must be chosen to represent the experimental conditions. This can range from simple ideal scenarios to more complex interactions.
- Software Utilization: Computational tools are essential for data fitting and analysis. Programs like SEDFIT and SEDPHAT are popular options for extracting meaningful parameters from the collected data.
- Statistical Evaluation: Results should undergo statistical scrutiny to validate findings and assess reliability. This is often overlooked but is detrimental for precise conclusions.
Applications of Analytical Ultracentrifugation
Analytical ultracentrifugation (AUC) is a technique essential for various fields, such as biochemistry, nanotechnology, and pharmaceutical research. This section delves into the significant applications of AUC, revealing how it contributes to substantiating scientific insights and advancing technology. It encompasses biomolecular characterization, nanoparticle analysis, and protein interaction studies, each highlighting the nuanced capabilities of AUC in addressing complex research problems.
Biomolecular Characterization
Biomolecular characterization through AUC allows for the precise determination of molecular weight, shape, and sedimentation coefficients of macromolecules. The technique makes it possible to analyze biopolymers like proteins, nucleic acids, and polysaccharides, offering a view into their composition and behavior in solution. Understanding these properties is crucial for researchers aiming to develop new biomaterials or drugs.
AUC provides insights into heterogeneous samples, identifying subtle variations in molecular populations that other methods may overlook. This precision benefits not only fundamental research but also practical applications like drug formulation and quality control in biotechnology.
Nanoparticle Analysis
Nanoparticles present unique challenges due to their size and behavior at the nanoscale. AUC effectively characterizes nanoparticles by measuring their size distribution, buoyant density, and aggregation state under various conditions. This is particularly valuable in fields like materials science and medicine where the behavior of nanoparticles influences their efficacy in applications like targeted drug delivery.
Moreover, the ability of AUC to analyze samples in solution makes it advantageous for real-time studies. Researchers can track changes in nanoparticle characteristics over time, providing a robust understanding of stability and interactions within a biological environment.
Protein Interaction Studies
Understanding protein interactions is fundamental in many biological processes. AUC facilitates the study of these interactions by measuring sedimentation behavior in mixed solutions. This enables the identification of binding affinities and stoichiometries without the need for labeling molecules.
Through AUC, researchers can explore complex associations, such as those occurring in signaling pathways or enzymatic reactions. The results obtained yield insights into molecular mechanisms and can guide the design of inhibitors or activators targeting specific interactions.
"The analytical ultracentrifugation technique plays a pivotal role in advancing our knowledge about molecular interactions in biochemistry and nanotechnology."
In summary, the applications of analytical ultracentrifugation extend across various scientific domains, enhancing our understanding of macromolecules and their functions. Researchers benefit from the detailed data provided by AUC, which translates into real-world applications and scientific innovations.
Advantages of Analytical Ultracentrifugation Service
Analytical ultracentrifugation provides several key advantages that make it a preferred analytical method in many scientific fields. These advantages are primarily related to the resolution, versatility, and non-destructive nature of the analysis it offers. Understanding these benefits can help researchers and professionals appreciate the capabilities of this technique and how it fits into their work.
High Resolution and Sensitivity
One of the most significant advantages of analytical ultracentrifugation is its high resolution and sensitivity. The method allows for precise measurement of sedimentation rates, which are critical for characterizing macromolecules. This high resolution is achieved due to the powerful centrifugal forces created during the centrifugation process. The sensitivity of this technique enables the detection of even low concentrations of biomolecules. This quality is especially relevant when dealing with heterogeneous samples where components may only exist in trace amounts. The ability to clearly differentiate between similar species adds great value to experimental data and often leads to more conclusive insights.
Versatility Across Disciplines
Analytical ultracentrifugation is not limited to just one area of study; it is a versatile tool applicable in various scientific disciplines. Biochemistry, molecular biology, and materials science are only some examples where this methodology shines. Researchers can use it to study proteins, nucleic acids, nanoparticles, and more. This flexibility means that it can be adapted for various experimental designs, whether for generating fundamental data or for specific industrial applications. When thinking about cross-disciplinary collaborations, using a method as versatile as this can significantly enhance the scope of research and innovation.
Non-Destructive Analysis
Another notable benefit of analytical ultracentrifugation is its non-destructive nature. Many analytical techniques compromise the sample in some way, affecting subsequent experimentation or analysis. However, in analytical ultracentrifugation, samples can often be retrieved after analysis, allowing for additional tests and validations. This reduces waste, saving both time and materials while providing the opportunity for further exploration. The non-destructive aspect also enables multiple analyses in varying conditions, which is crucial when characterizing the behavior of complex systems under different environments.
"By employing analytical ultracentrifugation, researchers not only maximize the potential use of their samples but also enhance the reliability of their findings."
In summary, the advantages of analytical ultracentrifugation service—its high resolution and sensitivity, versatility across disciplines, and non-destructive nature—make it an essential tool for researchers. Understanding these benefits positions scientists to leverage this technique effectively in their investigations.
Limitations and Challenges
Understanding the limitations and challenges of analytical ultracentrifugation is essential for researchers and practitioners. This section addresses critical components that are involved in data interpretation, operational constraints, and cost considerations. Recognizing these challenges allows scientists to make informed decisions and optimize their use of this powerful technique.
Complexity of Data Interpretation
The interpretation of data from analytical ultracentrifugation can be intricate and requires a robust understanding of the principles behind the technique. Factors such as sedimentation coefficients, molecular weight, and hydrodynamic properties play significant roles in the results obtained. Misinterpretation can lead to incorrect conclusions, ultimately affecting research outcomes.
Additionally, the software used for data analysis can present a steep learning curve. Many researchers may find themselves overwhelmed by the variety of models and algorithms available. Each has different assumptions and prerequisites, which can lead to errors if not properly understood. It's crucial to invest time in training and familiarization with the analytical tools to mitigate these complexities.
Operational Constraints
Operational constraints of analytical ultracentrifugation can pose significant challenges to laboratories. First, the need for specialized equipment limits accessibility. Not every lab can afford or maintain a high-speed ultracentrifuge. This restriction often necessitates outsourcing samples to specialized service providers, which may lead to additional delays in research timelines.
Moreover, specific sample preparation techniques must be strictly adhered to. Inconsistent sample qualities or improper loading can skew the results, rendering the entire analysis ineffective. Therefore, achieving accurate and reliable results often requires close attention to detail. Operational efficiency is also necessary. Researchers must ensure that they have trained personnel who can operate the machinery and interpret data correctly.
Cost Considerations
Cost considerations are another critical aspect when engaging in analytical ultracentrifugation. The initial investment in purchasing an ultracentrifuge can be substantial. Beyond the purchase, ongoing operational costs including maintenance, consumables, and potential service fees can add up.
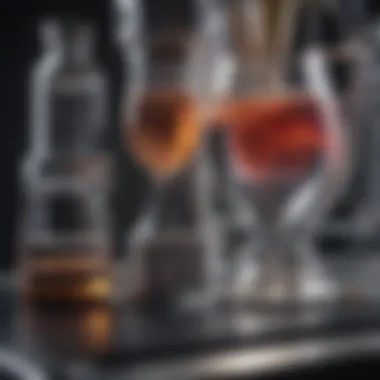
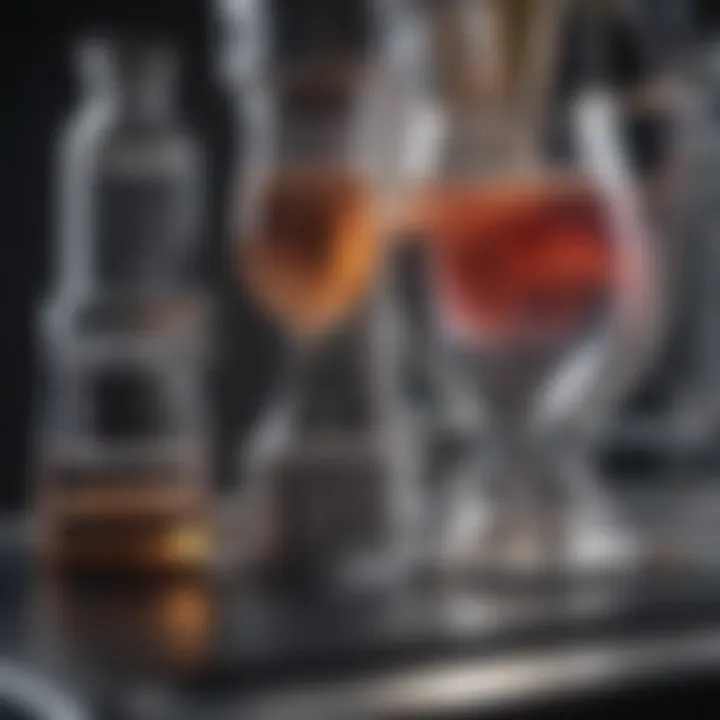
Outsourcing to specialized analytical ultracentrifugation services can also be expensive. While these services can alleviate the burden of operation, the cost per sample can be prohibitive for routine analysis. It's important for organizations to weigh the costs against the benefits, taking into account the need for precision in their experiments.
In summary, the limitations and challenges associated with analytical ultracentrifugation highlight the need for careful planning and consideration. By understanding the complexity of data interpretation, operational constraints, and related costs, researchers can better navigate this analytical technique and enhance their scientific endeavors.
Case Studies in Analytical Ultracentrifugation
Case studies in analytical ultracentrifugation (AUC) serve as vital resources that illustrate the real-world application and impact of this technique. These studies provide compelling examples of how AUC can characterize macromolecules and nanoparticles in various scientific fields. Importantly, they demonstrate not only the versatility of this analytical method but also its effectiveness in addressing complex biological and chemical questions.
Benefits of Case Studies
Case studies offer insights into actual scenarios where AUC has contributed to scientific advancement. They can uncover best practices for sample preparation, data collection, and analysis. Often, the insights gained from such case studies help streamline processes and reduce the chances of error in experimental procedures. Furthermore, these narratives can foster a deeper understanding of the limitations and challenges inherent in the technique, guiding future research endeavors.
Successful Applications in Biotechnology
In biotechnology, analytical ultracentrifugation has proven essential for characterizing proteins, nucleic acids, and other macromolecules. One notable application is in the determination of protein size and shape. For example, researchers can use sedimentation velocity experiments to assess the purity and molecular weight of recombinant proteins. This is particularly important for biopharmaceuticals, where even slight variations in molecular characteristics can influence therapeutic efficacy.
Moreover, AUC is instrumental in understanding protein-protein interactions. A recent study demonstrated that AUC was utilized to reveal the binding kinetics between an antibody and its target antigen. Such insights are crucial for optimizing therapeutic formulas and ensuring desired biological responses in clinical settings. The capability to conduct non-destructive analysis means that samples remain intact for further testing, a significant advantage in biotechnological research.
Insights from Pharmaceutical Development
Pharmaceutical development has also benefitted from the analytical capabilities of AUC. The technique aids in the characterization of drug formulations, especially those involving biological molecules. For instance, a prominent application of AUC is the evaluation of liposomal formulations. Researchers can determine the size distribution and density of liposomes, which directly affect their therapeutic properties and efficacy.
Additionally, AUC plays a critical role in the formulation stability studies of drugs. By observing the sedimentation behavior of drug formulations over time, scientists can gather data on potential aggregation and degradation. For example, a recent AUC study detailed how a specific protein drug maintained its stability over extended periods under various conditions, providing valuable information for storage and handling procedures.
The data derived from AUC studies empower researchers to make informed decisions during the early stages of drug development, greatly enhancing the probability of successful outcomes.
Culmination
The narratives from successful applications in biotechnology and pharmaceutical development underscore the significance of analytical ultracentrifugation. Each case study not only showcases the versatility of the method but also emphasizes the importance of ongoing research and innovation in this field. As scientists continue to explore its potential, AUC will likely remain a cornerstone technique for macromolecular analysis and characterization.
Future Perspectives
The future perspectives of analytical ultracentrifugation are essential for the ongoing development and application of this technique in various scientific fields. Understanding emerging trends, technological advancements, and accessibility can significantly influence the effectiveness of ultracentrifugation service in research and industry. With increasing complexity in biomolecular studies and the need for precise characterization, innovations in analytical ultracentrifugation are vital.
Emerging Trends in Technology
Recent advancements in technology enhance the capabilities of analytical ultracentrifugation. One noteworthy trend is the integration of automation. Automated systems allow for higher throughput and ensure reproducibility of results which is important in high-stakes research environments. Additionally, developments in detection methods, such as the incorporation of multi-wavelength detection, improve the ability to analyze complex samples by providing real-time data.
Another emerging trend is miniaturization. Smaller, more compact devices provide researchers with the capability to conduct analyses in limited spaces while consuming fewer resources. This trend is particularly advantageous for labs that face constraints due to budget or equipment space. Furthermore, software updates for data analysis streamline interpretations and enhance user experience, making it more approachable for those new in this field.
Potential for Increased Accessibility of Services
Increased accessibility of analytical ultracentrifugation services is a critical consideration moving forward. The rise of collaboration between academic and commercial laboratories has made this technology more available. Previously, only a handful of institutions had the necessary resources to conduct high-quality analytical ultracentrifugation. However, the sharing of equipment and expertise between facilities can democratize access.
The expansion of training programs and workshops also plays a significant role in improving accessibility. Educating the next generation of scientists enhances the skill set required to utilize this sophisticated technique. Furthermore, remote services and cloud-based data analysis platforms can support researchers regardless of their location. Such advancements mean that teams in various countries can analyze data collectively and contribute to integrative studies efficiently.
"By increasing accessibility to analytical ultracentrifugation, we open doors for innovative research and application that could not be realized otherwise."
The combination of these emerging trends and increased accessibility will likely shape the future of analytical ultracentrifugation. They will facilitate groundbreaking research in fields such as biotechnology, pharmaceuticals, and nanotechnology, emphasizing the necessity of ongoing investment and development in these areas.
End
The conclusion of this article encapsulates the significance of analytical ultracentrifugation in scientific research. This technique is not just a peripheral tool; rather, it forms a critical backbone for investigations into biomolecular and nanoparticle characteristics. Understanding its complexities and practical applications can elevate the research quality, enabling accurate interpretations and reliable experimental results.
Summary of Key Points
In synthesizing the information from previous sections, several key points emerge:
- Definition and Relevance: Analytical ultracentrifugation is a versatile technique essential for characterizing macromolecules and nanoparticles across various scientific disciplines.
- Methodological Insights: Attention to sample preparation, buffer conditions, and meticulous experimental design greatly influence the outcome of the analysis.
- Technological Advancements: Recent developments in instrumentation enhance the resolution and sensitivity of measurements, broadening the scope of applications.
- Challenges: Despite its advantages, issues related to data interpretation complexity and operational costs remain significant hurdles in its application.
- Future Trends: Emerging technologies and increased service accessibility present opportunities for wider adoption and increased emphasis on research that leverages this analytical method.
Final Thoughts on Analytical Ultracentrifugation
As we contemplate the future of analytical ultracentrifugation, its role is undeniably vital in modern research. The continuous evolution of technology and the increasing recognition of its potential across multiple fields signify a promising trajectory. For researchers and practitioners, leveraging this technique can provide profound insights that drive innovation. Moreover, understanding its limitations can help in making informed choices about when to employ this method effectively.
Importance of Citing Sources
Citing sources not only enhances the academic integrity of the work but also allows interested readers to explore related topics more thoroughly. In the field of analytical ultracentrifugation, where methodologies and technologies evolve rapidly, referencing current literature ensures that the information remains relevant and accurate. By directing readers towards influential studies or reviews, one can facilitate deeper engagement with the topic.
Specific Elements to Include
In the references section, it is essential to consider a variety of source types:
- Peer-reviewed journal articles that provide empirical data,
- Books that elaborate on the principles and practices related to ultracentrifugation,
- Review papers which summarize key developments in the field,
- Conference papers and technical reports that might showcase recent advancements or applications.
Benefits of Strong Referencing
- Validation of Claims: Supports the validity of experimental results and discussions.
- Guidance for Future Research: Offers avenues for further exploration and study.
- Encourages Collaboration: Helps researchers connect with cited authors or related academics.
- Promotes Academic Integrity: Reduces the chances of plagiarism and fosters respect for intellectual property.
By meticulously gathering citations from credible sources, authors can ensure that their references section stands as a testament to their dedication to high-quality research and dedication to assisting their readers. Such diligence aids in enhancing the overall understanding of analytical ultracentrifugation, aligning with the goals of scholars, practitioners, and students alike.