Comprehensive Guide to Cell Culture Techniques
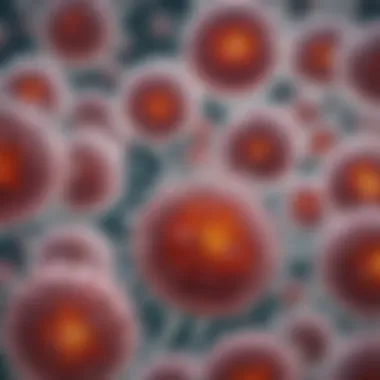
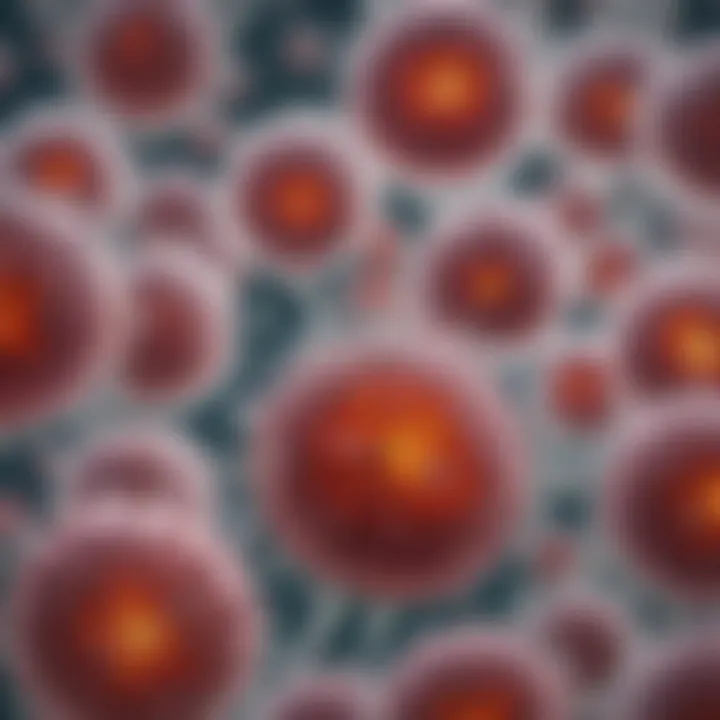
Intro
Cell culture techniques are vital in the realm of biological sciences. They allow researchers to study cells in a controlled environment, facilitating advancements in areas such as pharmacology, genetics, and cancer research. Understanding these methods is critical for anyone involved in biological studies. This article unveils essential techniques, methodologies, and ethical considerations in cell culture.
Culturing cells is more than mere technique; it arises from a variety of scientific contexts aiming to solve complex problems. From exploring cellular behavior to developing vaccines, the applicability of cell culture is vast. Each study employing these methods contributes to our growing knowledge of cell biology, influencing real-world outcomes.
Research Background
Overview of the Scientific Problem Addressed
The manipulation of cells presents significant opportunities for scientific research but also poses numerous challenges. Cultured cells may not perfectly reflect their physiological counterparts, creating discrepancies in experimental results. Issues such as contamination, cellular differentiation, and the inherent limitations of in vitro models must be addressed rigorously.
Historical Context and Previous Studies
Cell culture techniques originated in the early 20th century, with landmark studies paving the way for contemporary methods. One of the first successful cell cultures was achieved by Harrison in 1907, using frog nerve cells. Not long after, techniques evolved to include various mammals, leading to the establishment of standardized practices. Over the decades, research showcased the potential of cell culture in various fields, leading to significant advancements in medical sciences.
Influential studies have incrementally improved protocols, illuminating both the potential and limitations of these techniques. Extensive literature emphasizes the importance of refining methodologies to overcome the challenges researchers encounter today.
Findings and Discussion
Key Results of the Research
Many studies have examined the impact of different variables on cell culture success. For example, factors such as temperature, pH, and nutrient availability can alter cell viability and function. Consistently, data reveal the necessity of monitoring environmental conditions closely to achieve optimal outcomes. Furthermore, advancements in bioreactor designs have enhanced our ability to culture large volumes of cells, significantly supporting industrial applications.
Interpretation of the Findings
The findings indicate that a thorough understanding of cellular needs is paramount for successful culturing. Researchers must adopt a tailored approach, aligning environmental conditions with specific cell types and their applications. Without this nuanced approach, unexpected discrepancies may arise, undermining research validity. This not only stresses the importance of careful planning but also compellingly shows that the journey of cell culture is both intricate and demanding.
"Effective cell culture is as much an art as a science, requiring diligent attention to detail and an adaptive mindset."
Preamble to Cell Culture
Cell culture techniques play a critical role in modern biological research. They provide scientists with the ability to manipulate and study live cells outside their natural environments. Understanding how to properly culture cells allows researchers to investigate cellular behaviors, test hypotheses, and develop new treatments for diseases. The importance of cell culture hinges on its versatility and applicability across various fields including pharmacology, toxicology, and cellular biology.
Cell culture not only enables enhanced study of cell physiology but also facilitates large-scale production of biological products such as vaccines and monoclonal antibodies. Moreover, these techniques are essential for the study of disease progression, drug interaction, and genetic modifications. By mastering cell culture methods, professionals can create in vitro models that simulate in vivo conditions, thus yielding significant insights into human health.
The considerations are vast. One must be aware of the requirements for maintaining sterile conditions, proper nutrient formulations, and optimal environmental conditions, including temperature and CO2 levels. These factors are pivotal in ensuring cell viability and reproducibility of experiments. Successful cell cultures can serve as reliable models for understanding complex biological processes, but failures can lead to significant setbacks.
In summary, the mastery of cell culture techniques is an indispensable skill for researchers and professionals engaging in biological experimentation. It facilitates innovation and improves our capabilities in studying and combating diseases.
Definition of Cell Culture
Cell culture is defined as the process of growing cells in a controlled environment outside their natural habitat. This involves isolating cells from living organisms and placing them in culture vessels that provide necessary nutrients and conditions for growth. The term encompasses various methodologies, including culturing primary cells, established cell lines, and stem cells.
Cultured cells can thrive indefinitely under proper conditions, allowing extensive studies on their behavior and interactions. This controlled environment eliminates many variables present in whole organisms, providing a more straightforward basis for experiments.
Historical Background
The history of cell culture dates back to the early 20th century when the first successful attempts were made to grow cells in vitro. Notable milestones include George Gey's work in the 1950s with HeLa cells, derived from cervical cancer tissue. These cells became the first immortalized cell line, revolutionizing research by providing a continuous source of human cells for a wide array of studies.
Over the decades, advances in technology and methodology have significantly expanded the field. Development of serum-free media and improved aseptic techniques has enhanced the reliability of cell culture. The introduction of bioreactors has further scaled up production, allowing for industrial applications. Today, cell culture is a foundational technique in laboratories worldwide, driving forward research in regenerative medicine, genomics, and beyond.
Types of Cell Cultures
The section on Types of Cell Cultures is essential to understanding the variety of methodologies used in biological research. Each type of cell culture has unique characteristics, advantages, and limitations. Selecting the appropriate cell culture type is critical as it directly affects the outcomes of any research. Furthermore, knowing the distinctions between these cultures allows researchers to make informed decisions based on their specific requirements. This section outlines four primary types of cell cultures: primary cell cultures, secondary cell cultures, cell lines, and stem cell cultures.
Primary Cell Cultures
Primary cell cultures are derived directly from tissues or organs. These cells typically retain their original characteristics and functions better than those derived from cell lines. Primary cultures provide more physiologically relevant information, making them valuable for specific applications like toxicology and pharmacology. The main challenge with primary cultures is their limited lifespan; they can only grow for a certain period due to cellular senescence. Moreover, they may display significant variability between cultures, which complicates experimental reproducibility.
Secondary Cell Cultures
Secondary cell cultures arise from the subculturing of primary cultures. In this type, cells are allowed to grow to confluence before a portion is transferred to a new culture vessel. The advantage of secondary cultures is their extended growth capability, allowing for more extensive studies. However, these cultures may lose some of the original cellular characteristics over time. Researchers need to be aware of potential changes in behavior, morphology, and function as the cells adapt to the new environment.
Cell Lines
Cell lines are established from primary cultures that have been genetically modified to proliferate indefinitely. These lines are essential for varied applications, including drug discovery and fundamental research. Popular examples of cell lines include HeLa, CHO, and 293T. The primary benefit of using established cell lines is their consistency and ease of use. However, the downside is that they may not accurately represent normal physiological conditions, as genetic alterations can lead to changes in gene expression and cellular behavior.
Stem Cell Cultures
Stem cell cultures involve the growth and maintenance of stem cells, which have the unique ability to differentiate into various cell types. These cultures play a significant role in regenerative medicine, developmental biology, and drug discovery. The two main types of stem cells are embryonic stem cells and adult stem cells. While stem cell cultures hold remarkable potential, ethical considerations must be addressed, especially regarding the source of embryonic stem cells. Moreover, maintaining stem cell pluripotency and directing differentiation require specific conditions and treatments to ensure desired outcomes.
"Understanding the complexities of these different cell cultures is pivotal in advancing innovative research across various fields."
In summary, the understanding of the types of cell culturesโprimary, secondary, cell lines, and stem stem cell culturesโis vital. Each type presents unique benefits and consideration that influence experimental design and research applicability. Researchers must carefully assess these differences to select the most suitable cell culture type for their specific aims.
Essential Equipment for Cell Culture
The success of cell culture experiments relies heavily on the right tools and equipment. Understanding the essential equipment for cell culture is vital for any researcher or student delving into biological sciences. Proper equipment ensures optimal growth conditions, minimizes contamination, and allows for reproducible results. Here, we explore key items used in cell culture, their importance, and considerations for their use.
Culture Vessels
Culture vessels are fundamental for cell culture. They provide a confined space for cells to grow. These vessels come in various types, including Petri dishes, flasks, and multi-well plates. Each vessel has specific uses depending on the type of cells and the type of experiment being conducted.
- Materials: Culture vessels can be made from polystyrene, glass, or treated plastic. The choice of material can influence cell attachment and growth.
- Surface Treatments: Some vessels are coated to enhance adhesion for anchorage-dependent cells. This treatment can significantly improve cell growth and yield.
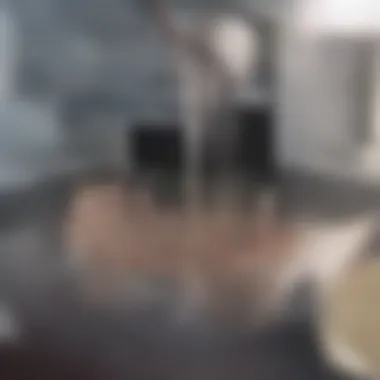
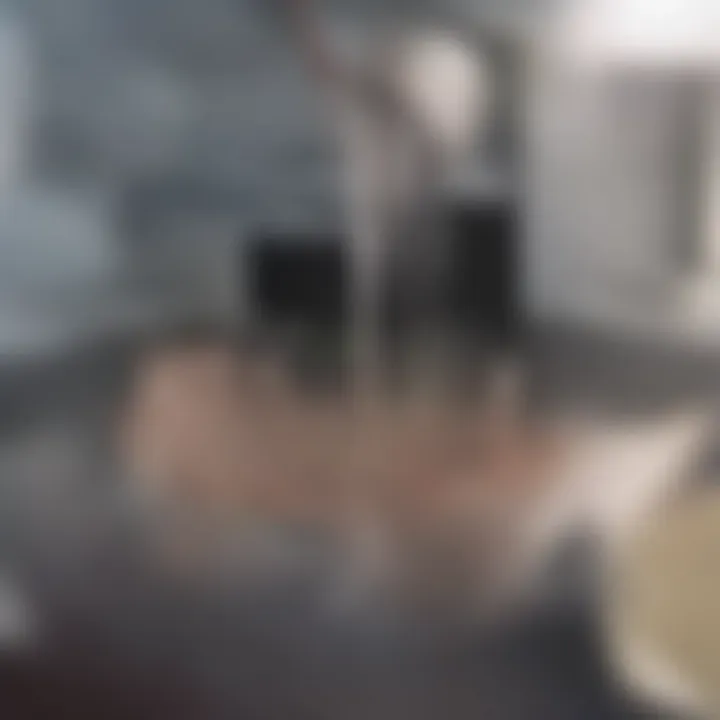
Utilizing the correct culture vessels impacts not only cell morphology but also cell behavior during experiments.
Incubators
Incubators create a controlled environment suitable for cell growth. Maintaining optimal conditions is crucial as cells require specific temperature, humidity, and gas composition.
- Temperature Control: Most mammalian cells thrive at 37ยฐC. Any deviation can lead to impaired growth and viability.
- C02 and O2 Levels: Many incubators allow for adjustments in carbon dioxide levels, which is essential for maintaining pH balance in culture media.
Maintaining a stable environment in incubators is one of the most crucial aspects of successful cell culture.
Bioreactors
Bioreactors are advanced systems designed for large-scale cell culture. They offer a more complex and controlled environment than standard incubators.
- Volume Capacity: Bioreactors can accommodate large volumes, making them suitable for industrial applications such as vaccine production and biopharmaceutical manufacturing.
- Real-time Monitoring: Many modern bioreactors include sensors to monitor pH, temperature, oxygen, and CO2 concentrations in real time, thus allowing immediate adjustments to the culture environment.
Selecting the right bioreactor and understanding its operational principles can enhance productivity while reducing risks of contamination.
Aseptic Tools and Accessories
Aseptic techniques are essential to prevent contamination during cell culture. Using proper tools and accessories plays a critical role in maintaining aseptic conditions.
- Sterile Pipettes and Tips: These ensure that no unwanted microorganisms are introduced into the culture.
- Laminar Flow Hoods: They provide a sterile work area by filtering air, essential for transferring cells and media.
- Protective Equipment: Gloves, lab coats, and masks should be worn to minimize the risk of contamination from the researcher.
An understanding of aseptic techniques and the related equipment can safeguard the integrity of cell cultures and ensure reliable outcomes in experiments.
Common Media for Cell Culture
Cell culture media serve as an essential component for maintaining and growing cells in vitro. The choice of media can greatly influence cell behavior, viability, and experimental outcomes. Each type of media provides a unique composition of nutrients, growth factors, and environmental conditions tailored to specific cell types. Understanding the characteristics and applications of different media is crucial for researchers aiming to optimize their cell culture techniques.
Basic Media Types
Basic media forms the foundation for many cell culture applications. These media typically contain essential nutrients such as amino acids, vitamins, salts, and glucose. Common examples include Eagle's Minimum Essential Medium (EMEM) and Dulbecco's Modified Eagle Medium (DMEM). Both are versatile, supporting cell lines ranging from fibroblasts to epithelial cells.
These media types are important as they create a proper environment for cell growth and division. However, they may lack specific components required for optimal performance.
- Amino Acids: Essential for protein synthesis. They help in cell growth and function.
- Vitamins: These act as co-factors in enzymatic reactions, aiding metabolism.
- Salts: Maintain osmotic balance and provide essential ions, which are vital for cellular function.
Serum-Based Media
Serum-based media involve adding animal serum, usually fetal bovine serum (FBS), to basic media. Serum provides a rich source of growth factors, hormones, and attachment factors that facilitate cell proliferation and survival. For many cell types, serum is indispensable.
While serum can enhance cell growth, it comes with its considerations. The composition of serum can vary significantly between batches, leading to reproducibility issues. Moreover, the use of animal-derived components raises ethical concerns and potential contamination risks.
Some key benefits of serum-based media:
- Growth Factors: Promote cellular proliferation and maintenance.
- Adhesion Factors: Enhance cell attachment and spreading.
- Nutrients: Provide a diverse nutrient profile that supports various cell lines.
Serum-Free Media
Serum-free media has been developed to overcome some of the limitations of serum-based options. These media are formulated to include all necessary components without relying on animal serum. Products like the Ham's F-10 and RPMI-1640 fit this description.
This type of media holds several advantages:
- Consistency: With no variations, reproducibility improves across experiments.
- Reduced Contamination: Lower risk of introducing pathogens or other unwanted substances.
- Ethical Considerations: Avoids the ethical issues associated with animal serum use.
However, it can be challenging to optimize, as growth factors must be supplemented to meet the specific needs of different cell types.
In summary, selecting the right culture media is essential in cell culture to ensure the desired outcomes in research and application. Each media type presents its own set of advantages and challenges, influencing experimental results.
Cell Culture Techniques
Cell culture techniques are fundamental to biological research. These methods form the backbone of experimental procedures, allowing scientists to grow and manipulate cells outside their natural environment. The importance of these techniques is evident in various applications ranging from drug testing to genetic engineering. Effective cell culture requires precision, skill, and knowledge of best practices. Understanding these techniques enables researchers to maximize cell viability and experimental reproducibility.
Aseptic Techniques
Aseptic techniques are crucial in cell culture to prevent contamination. This practice involves a series of methods designed to maintain a sterile environment during cell handling. Contamination can introduce unwanted microorganisms, thereby compromising experimental results.
To implement aseptic techniques, it is essential to:
- Use sterile equipment and reagents.
- Work near a flame or within a laminar flow hood to create a sterile air barrier.
- Regularly disinfect surfaces and equipment with appropriate solutions, such as 70% ethanol. By adhering to strict aseptic protocols, researchers can ensure the integrity of their cell cultures, thus generating more reliable data.
Cell Passaging
Cell passaging is the process of transferring cells from one culture vessel to another to sustain growth. It is essential for maintaining healthy cell lines. If cells are not passaged properly, they may reach confluence, leading to a decline in growth rates or even cell death.
The key steps involved in cell passaging include:
- Removing the culture medium from the vessel.
- Rinsing the cells with a buffer solution to remove residual serum proteins.
- Detaching the cells using enzymes or mechanical methods, like trypsinization.
- Diluting the cell suspension and placing it into new culture vessels with fresh medium. Understanding the timing and method of passaging is critical in achieving optimal cell growth and preserving cell line characteristics.
Cell Freezing and Thawing
Cell freezing and thawing are techniques used to preserve cell cultures for long-term storage. These methods allow researchers to stop cell metabolism and maintain their genetic integrity for future use.
Freezing involves:
- Preparing cells in a cryoprotectant solution, such as dimethyl sulfoxide (DMSO).
- Gradually cooling the cells at controlled rates before storage in liquid nitrogen.
Thawing requires careful attention to minimize cell stress. The steps are:
- Rapidly warming the frozen cells in a 37ยฐC water bath.
- Diluting the thawed cells to reduce chemical toxicity.
- Transferring them to fresh culture medium for recovery.
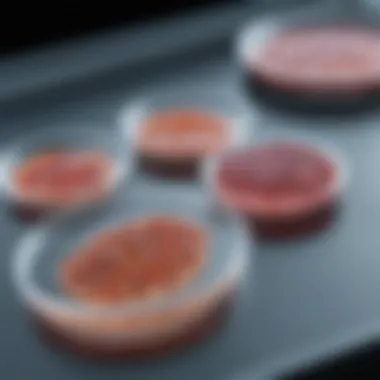
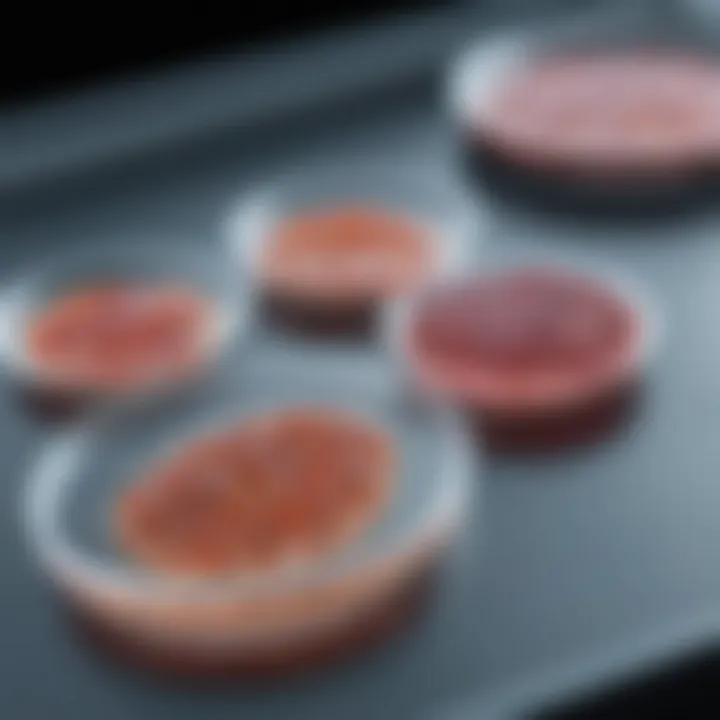
These techniques are vital for maintaining cell stocks, allowing for experiments over extended periods without loss of vital genetic information or cell function.
Proper implementation of cell freezing and thawing greatly affects the lifespan and behavior of cultured cells.
Mastering these cell culture techniques is essential for any researcher in the biological sciences. Each step in the process demands precision, attention to detail, and strict adherence to protocols. The effectiveness of experiments hinges on how well these techniques are applied.
Characterization of Cell Cultures
Characterizing cell cultures is crucial in determining their viability and functionality. This process involves assessing various parameters to ensure that the cells behave as expected and are suitable for specific research applications. Proper characterization helps to guarantee data integrity, reproducibility, and the overall success of experiments. Without adequate characterization, conclusions drawn from studies could be misleading or invalid.
Morphological Assessment
Morphological assessment is a fundamental part of cell culture characterization. This evaluation involves observing cell shape, size, and arrangement under a microscope. Cells often exhibit specific morphologies, which can be indicative of their type and health. For instance, a healthy culture may display uniform cell shapes and sizes, while an unhealthy culture might show significant variation or abnormal features.
Several techniques are used for morphological assessment:
- Light Microscopy: This allows for basic visualization of cell structures. It offers insights into cell density and morphology but lacks the resolution for detailed subcellular structures.
- Phase Contrast Microscopy: Useful for observing live cells without staining, phase contrast microscopy enhances contrast and enables clearer visualization of transparent cells.
- Fluorescence Microscopy: It enables detailed investigations of specific cellular components through the use of fluorescent dyes. This technique helps identify cellular markers and assess changes in morphology in response to treatment.
Overall, morphological assessment provides critical insights for evaluating cell health and culture quality. Without it, assumptions regarding the functionality of cultures may not be reliable.
Functional Assays
Functional assays are another vital aspect of cell culture characterization, complementing morphological assessments. These assays measure the cellsโ physiological activities and responses to various stimuli. They provide essential information relating to cell functionality and can include several approaches:
- Metabolic Activity Assays: These assays evaluate cell viability based on metabolic processes. Common techniques include the MTT assay, which assesses mitochondrial activity, and the resazurin reduction assay for detecting metabolically active cells.
- Proliferation Assays: These assays quantify the increase in cell numbers over time. The incorporation of labeling dyes like BrdU can help to visualize and track cell proliferation.
- Assays for Cell Signaling: These tests assess the functionality of signaling pathways within cells. They help in understanding cellular responses to external influences, which is essential for applications in drug development and toxicology.
Functional assays not only ascertain the health of cell cultures but also offer insight into how cells may behave under experimental conditions.
Troubleshooting in Cell Culture
Troubleshooting in cell culture is an essential aspect of maintaining healthy and viable cell populations. It involves identifying and addressing issues that can compromise the integrity and reproducibility of cultured cells. Troubleshooting is particularly critical as cell culture failures can lead to significant setbacks in research projects, wasted resources, and flawed data. Understanding common issues and their solutions equips researchers to minimize disruptions in their work, ultimately enhancing the efficiency and effectiveness of cellular research.
Contamination Issues
Contamination is one of the most prevalent problems encountered in cell culture. Sources of contamination may include bacteria, fungi, mycoplasma, or even other cell types. These unwanted organisms can disrupt cell growth, alter cellular behavior, and yield unreliable experimental outcomes.
Preventing contamination requires meticulous sterile techniques. Researchers should always work within a laminar flow hood and use proper aseptic protocols during cell handling. Common signs of contamination include turbidity in the culture medium and unexpected changes in cell morphology.
To address contamination, it is essential to take immediate action:
- Isolation: Separate contaminated cultures to prevent spreading.
- Identification: Utilize specific tests, such as plating on selective media or PCR, to identify the type of contaminant.
- Elimination: In some cases, antibiotics may be used, but this should be approached with caution, as it can induce resistance and complicate results.
- Reestablishment: Restart cultures from uncontaminated samples or use cryopreserved stocks if available.
"The key to a successful cell culture is maintaining sterility throughout the process."
Cell Viability Problems
Cell viability problems can manifest as low growth rates or high cell death, which directly affect experimental outcomes. Several factors may contribute to reduced cell viability, including inappropriate media composition, suboptimal culture conditions, or physical damage during pipetting and passaging.
To tackle cell viability challenges, several steps can be taken:
- Media Assessment: Regularly review the formulation of the culture media. Ensure that it contains necessary nutrients, growth factors, and appropriate pH.
- Temperature and COโ Levels: Maintain accurate temperature and COโ levels in incubators to sustain optimal growth conditions.
- Cell Density: Avoid overcrowded cultures, as higher cell density can lead to nutrient depletion and increased waste accumulation.
- Regular Monitoring: Perform cell viability assays, such as Trypan Blue exclusion or MTT assays, to monitor cellular health over time.
By staying vigilant about these concerns and implementing practical solutions, researchers can significantly improve cell viability outcomes and maintain the integrity of their cultures.
Applications of Cell Culture
The applications of cell culture are vast and pivotal in several fields of biological research. Understanding these applications is crucial for students, researchers, educators, and professionals who delve into cellular biology. Cell culture enables researchers to study cellular processes in a controlled environment, which significantly contributes to advancements in medical research and biotechnology. This section explores three key areas where cell culture plays an essential role: drug development, toxicology studies, and vaccine production.
Drug Development
Cell culture serves as a fundamental tool in the drug development process. Researchers use cultured cells to screen new compounds for therapeutic properties. The benefits of utilizing cell cultures in this context include:
- Cost-effectiveness: It is less expensive to cultivate cells rather than use whole organisms.
- Speed: Experiments can generally be performed faster in vitro.
- Controlled conditions: Researchers can manipulate growth factors and environmental conditions to study specific cell responses to drugs.
The process often begins with high-throughput screening of compounds against cell lines, allowing the identification of lead candidates. For example, the development of cancer treatments frequently involves assessing the cytotoxic effects of various agents on tumor cell lines. Furthermore, once promising candidates are identified, cell cultures can also assist in preclinical testing, providing insights into the pharmacokinetics and toxicity before advancing to animal studies or human trials.
Toxicology Studies
In toxicological research, cell culturing provides a significant advantage for assessing the safety of chemicals, drugs, and environmental agents. By exposing cell cultures to various substances, researchers can evaluate:
- Cellular responses to potential toxins.
- Mechanisms of toxicity at the molecular level.
- The concentration thresholds that would lead to adverse effects.
The ability to observe how cells react in a controlled setting facilitates the swift identification of harmful agents while minimizing the ethical concerns associated with animal testing. Results gleaned from these studies can inform regulations and guidelines to ensure public safety regarding new products.
"Cell culture techniques enable scientists to conduct experiments with precise control over variables, thereby yielding more reliable data on toxic effects."
Vaccine Production
Another critical application of cell culture lies in vaccine production. The development of vaccines requires cultivating cells that can support the growth of virus strains or bacteria. Live-attenuated and inactivated vaccines often rely on cell cultures to produce large quantities of pathogens, which are then harvested for vaccine formulation.
Utilizing cell cultures in this process has several implications:
- Increased yield: Cell cultures can provide higher quantities of viral antigens compared to traditional methods.
- Scalability: Cultured cells allow for rapid scaling of vaccine production to meet demand.
- Improved safety: Cell culture techniques minimize the risk of contamination with animal pathogens, making the process safer.
In recent years, advances such as the use of mammalian cell lines have enhanced the efficacy and safety profiles of vaccines. Researchers also continue to explore novel approaches like recombinant DNA technology, which leverages cell culture for vaccine development, emphasizing the important role of cell culture in public health.
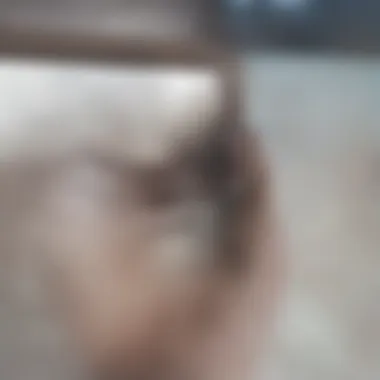
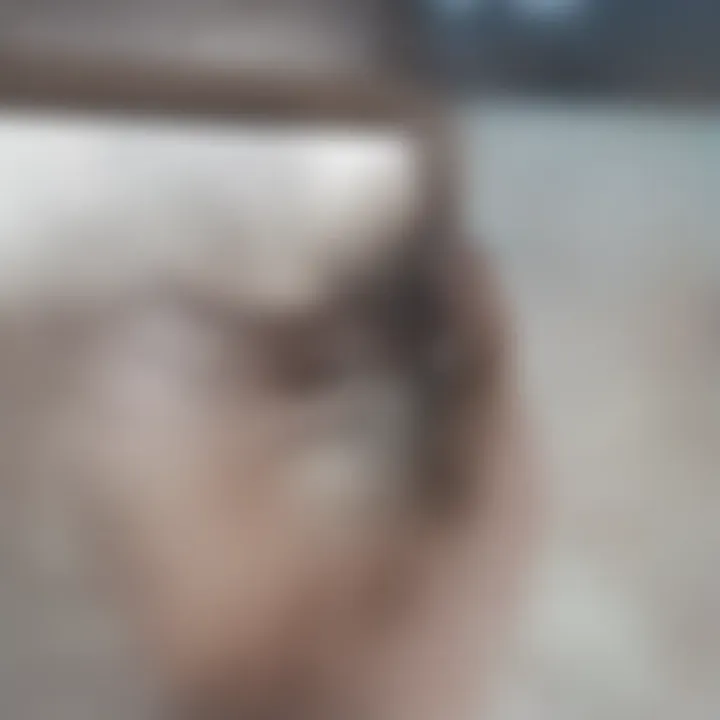
Ethical Considerations in Cell Culture
Ethical considerations in cell culture are vital for conducting responsible research. This topic influences not only the choice of methodologies but also the integrity of scientific inquiry itself. As researchers probe the complexities of biological systems, it is essential to maintain an understanding of ethical practices. This ensures the minimization of harm and promotes respect for life, however small or simple it may be. Moreover, it fosters public trust in science, paving the way for continued support and funding for research initiatives.
Use of Animal-Derived Cells
The use of animal-derived cells in cell culture is a controversial area. While these cells provide invaluable insights into biological processes, ethical dilemmas arise. The sourcing of these cells needs careful consideration. Researchers must abide by the principles of the 3RsโReplace, Reduce, and Refine. This involves seeking alternatives to animal-derived cells whenever possible, using fewer animals, and refining techniques to minimize suffering.
Many laboratories implement strict protocols to ensure compliance. This includes obtaining approval from relevant ethical committees before commencing research. Institutions often emphasize transparency in behalf of their research using animal-derived cells, promoting public engagement and understanding.
"Ethical oversight is an integral part of responsible research, ensuring that the rights and welfare of all living beings are upheld."
Regulatory Frameworks
Regulatory frameworks are crucial for guiding the ethical use of cell culture techniques. These frameworks provide guidelines that govern how researchers handle animal-derived cells and ensure that their research adheres to legal and ethical standards. Various national and international organizations oversee these regulations, including the National Institutes of Health (NIH) and the U.S. Department of Agriculture (USDA).
In many countries, adherence to these regulations is mandatory. Institutions must establish an Institutional Animal Care and Use Committee (IACUC) to review and approve all research proposals involving animal use. These committees ensure that researchers adhere to ethical standards and evaluate every aspect of proposed studies.
- Key components of regulatory frameworks include:
- Ethical review processes
- Requirements for animal welfare
- Reporting obligations in case of adverse outcomes
Maintaining compliance with regulations not only protects animals but also bolsters the validity and credibility of research findings. This demonstrates a commitment to ethical principles, enhancing the reputation of the scientific community at large. Adherence to these frameworks, therefore, plays a crucial role in fostering an environment of ethical responsibility in the pursuit of knowledge.
Future Trends in Cell Culture
The landscape of cell culture is rapidly evolving, driven by advancements in technology and the growing understanding of cellular behavior. The future of cell culture is not just vital for scientific research but also for clinical applications. As we explore future trends, we will address important elements, the benefits they offer, and the considerations that accompany these advancements in this field.
3D and 4D Cell Cultures
The shift from traditional two-dimensional (2D) cultures to three-dimensional (3D) and even four-dimensional (4D) cultures marks a significant escalation in the accuracy of biological modeling.
3D cell cultures better mimic the natural environment of cells within tissues. This configuration promotes more realistic cell behavior, allowing for enhanced cell interactions, differentiation, and response to drugs. The benefits are clear: researchers can gain deeper insights into cellular functions, leading to better predictions of clinical responses in vivo. Some key advantages of 3D cell cultures include the following:
- Increased cell viability and functionality
- Improved drug testing outcomes
- Enhanced tissue engineering capabilities
4D cell cultures introduce time as an additional dimension. This approach enables researchers to observe changes in cellular structure and behavior over time, capturing dynamic processes that are essential for understanding development and disease progression. The implications for personalized medicine, regenerative therapies, and cancer research are significant.
Personalized Medicine
Personalized medicine represents a significant leap in healthcare, aligning treatments more closely with individual patient profiles. In the context of cell culture, personalized approaches entail creating patient-specific cell lines, derived directly from a patient's cells.
This custom approach allows for tailored drug testing and treatment regimens, reflecting the unique genetic and phenotypic characteristics of the individual. Key considerations in personalized medicine with regard to cell culture include:
- The ethical implications of using human cells
- The need for stringent regulatory frameworks to ensure safety and efficacy
- The technological resources required for efficient cell manipulation and genetic engineering
As personalized medicine continues to gain traction, the synergy between advanced cell culture techniques and genomics will likely lead to breakthroughs that enhance patient care.
"The ongoing integration of advanced cell culture techniques into personalized medicine signifies a paradigm shift in how treatments are developed and implemented, moving toward more effective and individualized healthcare solutions."
In sum, the future trends in cell culture promise not just advancements in laboratory research but also practical applications that could redefine medical treatments. As researchers and practitioners in the field embrace these changes, they must also navigate the accompanying challenges, including ethical dilemmas and regulatory needs that arise with innovation.
Epilogue
The conclusion of this article encapsulates significant insights on cell culture techniques, highlighting their indispensable role in biological research. It synthesizes the shared knowledge throughout various sections while emphasizing critical considerations that enable researchers and practitioners to excel in their endeavors. By understanding the multifaceted aspects of cell culture from equipment and methods to ethical implications, one can appreciate the profound impact these techniques have on scientific progress.
Each of the different methodologies discussed serves not only practical functions but also contributes to a broader understanding of cellular behavior in diverse environments. Furthermore, awareness of the ethical frameworks and future trends leads practitioners to not only focus on technical execution but also on responsible and progressive application.
"The potential for discovery in cell culture is vast, yet it is crucial to approach it with diligence and integrity."
Adherence to established best practices ensures the reliability of research outcomes. This ultimately reinforces the credibility of findings and fosters a culture of excellence in scientific inquiry.
Summary of Key Points
The sections explored fundamental concepts and applications of cell culture, cementing foundational knowledge essential for researchers:
- Definition and Historical Context: Understanding the evolution of cell culture.
- Types of Cultures: Differentiating primary from secondary cultures and recognizing cell lines and stem cell cultures.
- Essential Equipment: A survey of the instruments necessary for effective cultures, including incubators and bioreactors.
- Media Types: The significance of choosing the appropriate media for optimal cell growth.
- Techniques: Detailed insights into aseptic methods, cell passaging, and cryopreservation.
- Characterization and Applications: Evaluating cell cultures for drug development, toxicological assessment, and vaccine production.
- Troubleshooting: Strategies for managing common issues like contamination and viability problems.
- Ethical Considerations: Navigating ethical challenges associated with utilizing animal-derived cells.
- Future Trends: Exploring advancements like 3D and personalized medicine applications.
Recommendations for Best Practices
To maximize the efficiency and effectiveness of cell culture work, adherence to best practices is paramount:
- Maintain Aseptic Conditions: Prioritize sterility in all procedures to minimize contamination risks.
- Choose Appropriate Media: Align media selection with cell type requirements and ensure proper supplementing, if necessary.
- Monitor Cell Health: Regularly assess morphology and growth rates to determine optimal passaging schedules.
- Document Procedures Rigorously: Maintain detailed records of culture conditions, passage history, and any anomalies.
- Implement Quality Control: Regularly test for mycoplasma and other contaminants to ensure cell line integrity.
- Be Ethical: Follow ethical guidelines and regulatory frameworks to promote responsible research practices.
By integrating these best practices, researchers can enhance the reliability and reproducibility of their results, thereby contributing to the advancement of biological sciences.
Primary Literature
Primary literature constitutes original research articles that present new findings in the field of cell culture. These documents are vital; they report on experiments, methodologies, and results, directly contributing to the body of knowledge in cell culture.
For students and professionals alike, accessing primary literature is essential for staying current with advances in methodologies and techniques. Journals such as Cell or Tissue Culture Methods are well-regarded sources of primary research. Reading these articles critically helps in understanding the experimental design, results obtained, and the interpretations made by the authors.
Engaging with primary literature fosters critical thinking skills, encouraging readers to analyze methodologies and outcomes critically. By evaluating these sources, researchers can identify gaps in knowledge that their work might address. The challenge often lies in navigating through the vast number of articles, which requires effective strategies for assessing relevance and quality.
Review Articles
Review articles synthesize existing literature, offering comprehensive overviews of specific topics within cell culture. They serve as invaluable resources for newcomers and experienced researchers alike. By aggregating findings from multiple studies, review articles contextualize information and reflect on the state of the field.
Such articles summarize advancements and benchmark methodologies against one another, enabling researchers to gain insights without having to delve into numerous individual studies. Well-written reviews highlight trends and differences in cell culture techniques, facilitating informed decision-making during experimentation.
Furthermore, review articles often discuss future directions in research. They provide foresight into emerging techniques, potential applications, and unresolved questions in the field. This makes them particularly valuable for guiding research agendas and funding proposals.