Exploring Heat and Electricity: A Dynamic Relationship
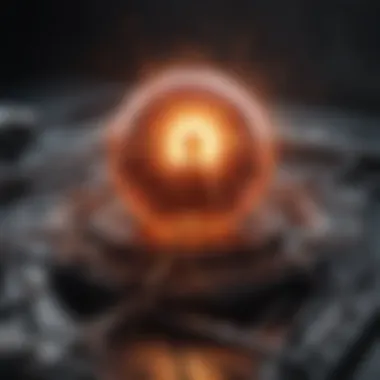
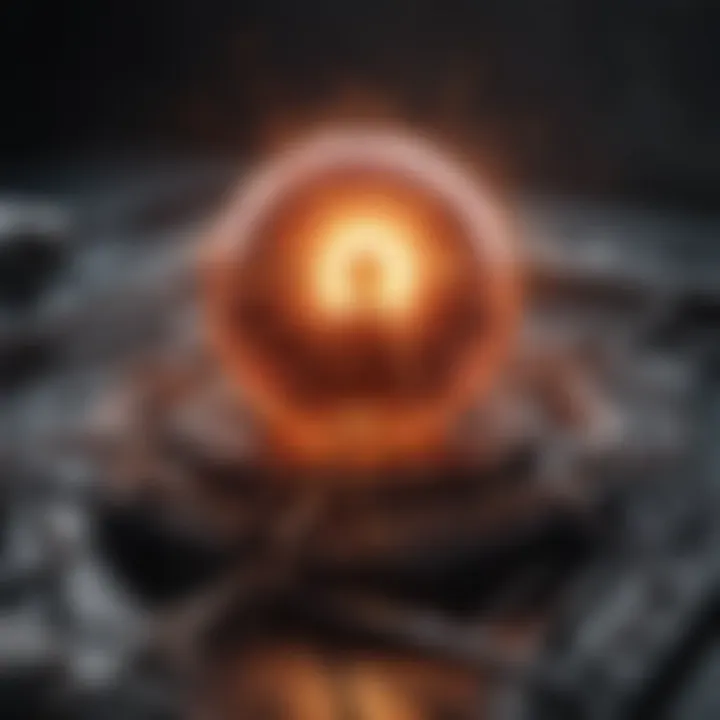
Intro
Heat and electricity are two fundamental forms of energy that play crucial roles in modern science and technology. Their interplay has influenced everything from everyday appliances to large-scale energy systems. Understanding this dynamic relationship not only enriches scientific knowledge but also opens avenues for innovation in energy efficiency and sustainability. This is particularly important in todayβs world, where energy challenges continue to escalate due to climate change and resource depletion. Exploring how heat can be transformed into electrical energy, and vice versa, is vital for developing new solutions and optimizing existing technologies.
Research Background
Overview of the Scientific Problem Addressed
The study of heat and electricity centers around thermodynamics and electromagnetism. These fields examine how energy can be converted and transferred. A key problem in this area is maximizing the efficiency of these energy conversion processes. For example, traditional power plants waste a considerable amount of energy as heat. Therefore, understanding the mechanisms through which these energy forms interact is essential for enhancing energy efficiency and creating more sustainable systems.
Historical Context and Previous Studies
Throughout history, the relationship between heat and electricity has evolved dramatically. Early experiments by scientists such as Benjamin Franklin and Michael Faraday laid the groundwork for understanding electric currents and electromagnetic fields. Later developments, such as the creation of the thermoelectric generator, showcased practical applications of these principles. Significant research has continued in recent decades, particularly around renewable energy technologies that utilize thermoelectric effects. Findings from studies have revealed crucial insights into energy conversion efficiency, providing a foundation for current advancements.
Findings and Discussion
Key Results of the Research
Recent studies highlight several important findings regarding heat and electricity. For instance, the efficacy of thermoelectric materials has seen significant improvements. New materials, such as skutterudites and half-Heusler alloys, display enhanced thermoelectric performance, which can lead to better temperature gradients and higher energy conversion rates.
Moreover, advancements in nanotechnology have allowed scientists to manipulate heat transfer at the nanoscale, resulting in greater precision in electricity generation from thermal sources.
Interpretation of the Findings
The implications of these findings are profound. Enhanced thermoelectric materials not only push the limits of efficiency but also hold promise for renewable energy applications. In a world moving towards sustainability, these innovations could replace or complement traditional energy sources. Furthermore, understanding the principles governing heat and electricity can inspire new approaches to energy capture and usage, ultimately contributing to solutions for contemporary energy challenges.
"Mastering the interplay between heat and electricity is key to improving efficiency and sustainability in energy systems."
This exploration of heat and electricity reveals a synergy that is critical for further advancements in energy technology. As researchers and practitioners continue to investigate these dynamics, the potential for innovation remains vast.
Prologue to Heat and Electricity
The interplay between heat and electricity is central to a variety of scientific disciplines and practical applications. Understanding this relationship is not only essential for academic inquiry but also for technological innovation. Heat and electricity both represent fundamental forms of energy, yet they manifest in unique ways. This article aims to explore their dynamic relationship and the principles governing their interaction.
Heat is a form of energy associated with the movement of atoms and molecules in matter. It can be transformed into electricity through various processes, such as thermoelectric effects and heat engines. Gaining a solid grasp of heat and its behavior allows researchers and engineers to create more efficient systems. On the other hand, electricity, the flow of electric charge, has revolutionized industries and daily life.
Understanding the definitions and implications of both heat and electricity is crucial. This knowledge serves as the foundation for advanced concepts such as thermodynamics and electromagnetic theory. Furthermore, the integration of both forms of energy in real-world applications highlights their relevance in addressing contemporary energy challenges, such as climate change and resource management.
Given the increasing importance of energy efficiency and renewable resources, the relationship between heat and electricity remains a topic of great significance in both research and industry. As advancements in technology continue to evolve, a deeper understanding of these dynamics will be pivotal in shaping a sustainable future.
Definition of Heat
Heat can be defined as the energy transfer between systems or bodies due to temperature differences. It flows from high-temperature regions to low-temperature ones, seeking equilibrium. In essence, heat is linked to the kinetic energy of particles within a substance. This movement can be quantified and expressed in several units, such as joules or calories.
In practical terms, applications of heat are seen in daily processes such as cooking and heating spaces. However, in a scientific context, heat is a fundamental concept in thermodynamics.
- Transfer Methods:
- Conduction: Transfer of heat through direct contact.
- Convection: Movement of heat through fluids (liquids or gases).
- Radiation: Transfer of heat through electromagnetic waves.
Understanding heat's behavior is critical for several reasons. It lays the groundwork for thermal efficiency in energy systems and helps develop technologies aimed at better energy conservation.
Definition of Electricity
Electricity is defined as the set of physical phenomena associated with the presence and motion of electric charge. This charge can flow in circuits, driven by differences in electric potential. Electricity is expressed in units of volts, amperes, and ohms, each playing a crucial role in understanding electric systems.
Electricity has become an indispensable part of modern society. It powers homes, industries, and transportation systems, shaping the way we interact with the world. The generation and distribution of electricity involve numerous technologies, each with its own efficiency and impact strategies.
- Types of Electricity:
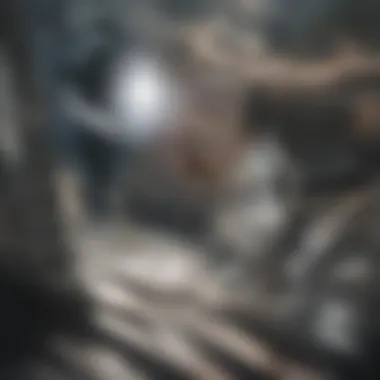
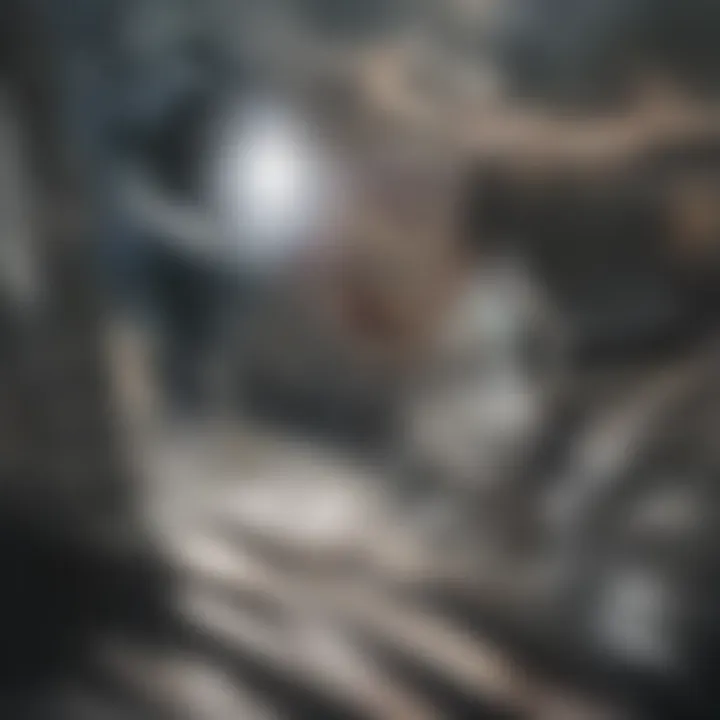
- Static Electricity: Accumulation of charge on the surface of an object.
- Current Electricity: The flow of electric charge through a conductor.
Recognizing the complexities and applications of electricity facilitates new developments in energy technologies. Through smart grid systems and renewable energy integration, the need for reliable electricity sources is increasingly relevant in addressing the world's energy demands.
Theoretical Foundations
Understanding the theoretical foundations of heat and electricity is essential in appreciating their complex interrelationship. These principles provide the framework through which we analyze how energy can be transformed from one form to another. The laws of thermodynamics and electromagnetic theory are foundational, allowing researchers and enthusiasts to predict behaviors of systems that involve heat and electricity. Through these foundations, we can explore not only the mechanics of energy conversion but also the limitations and efficiencies that govern these processes.
Thermodynamics Overview
First Law of Thermodynamics
The First Law of Thermodynamics deals with the principle of the conservation of energy. The focus here is that energy cannot be created or destroyed, only transformed from one form to another. This law is crucial for understanding how heat energy is converted into electrical energy, as well as vice versa. It is a beneficial aspect of this article because it establishes a clear baseline for all energy interactions in thermodynamic systems.
One unique feature of the First Law is its formulation into a mathematical equation, often represented as ( \Delta U = Q - W ), where ( \Delta U ) is the change in internal energy, ( Q ) is the heat added to the system, and ( W ) is the work done by the system. This formal approach not only provides clarity but also allows for quantitative assessments that can guide experimental design. The main advantage of invoking this law in our discussion lies in its fundamental applicability across a wide range of scenarios, from engines to refrigerators.
Second Law of Thermodynamics
The Second Law of Thermodynamics introduces the concept of entropy, asserting that in isolated systems, energy transformations tend to move toward a state of greater disorder. This law contributes significantly to the overall goal of understanding heat and electricity as it dictates the direction and feasibility of energy conversions. It is a vital component of this article due to its implications on efficiency and practical applications.
A key characteristic of this law is its emphasis on irreversibility in natural processes. For example, heat flows naturally from hot to cold bodies and not the other way around, which informs the design of energy systems. The unique feature here is that it offers insights into the limits of work that can be extracted from heat, impacting technologies like heat engines. The implication is clear: while energy can be converted, it is never completely efficient, highlighting an essential limitation in our quest for sustainable energy solutions.
Electromagnetic Theory
Maxwell's Equations
Maxwell's Equations are fundamental to understanding electric and magnetic fields and how they interact with each other. They are a set of four equations that describe how electric charges produce electric fields, how currents produce magnetic fields, and how changing magnetic fields produce electric currents. These equations are crucial to our understanding of electromagnetic phenomena and thus are a natural fit for this article.
The key characteristic of Maxwell's Equations is their unification of electricity, magnetism, and optics. This sets them apart as they encompass a wide range of physical phenomena beyond just electric and thermal considerations. The ability to describe a vast array of electromagnetic behavior provides significant advantages in fields that depend on both heat and electricity.
Electromagnetic Waves
Electromagnetic Waves are generated by oscillating electric and magnetic fields, which can propagate through space. The understanding of these waves is crucial for modern communication technologies and the conversion of energy forms. In the context of this article, their role is to bridge the gap between electrical and thermal energy, as their generation often involves processes that involve both.
A vital characteristic of electromagnetic waves is their ability to carry energy through space, which can then be harvested in various ways. This unique property means that they play a role in numerous applications, including solar energy collection systems, where sunlight is converted into electrical energy. The advantages here include efficient energy transfer and the potential for scaling in renewable energy technologies.
The exploration of heat and electricity through the lens of theoretical foundations offers a pathway to understanding the complexities of energy interactions in our modern world.
Conversion Processes
The conversion processes section elucidates the intricate mechanisms through which heat and electricity interrelate. Understanding these processes is essential, as they serve as the foundation for numerous applications in technology and energy efficiency. By investigating how heat can be transformed into electrical energy and vice versa, we can harness their potential more effectively. This insight is relevant in various industries, including energy production, heating, and cooling systems. Moreover, advancements in conversion technologies are pivotal in addressing current energy challenges, emphasizing the necessity of innovation in this field.
Thermoelectric Effect
The thermoelectric effect represents a unique phenomenon where a temperature gradient can generate an electric voltage, thus illustrating the interplay between heat and electricity. Its significance lies in its ability to convert waste heat into usable electrical energy. This aspect is crucial as society moves toward energy conservation and sustainability. The thermoelectric effect comprises two primary sub-effects: the Seebeck effect and the Peltier effect.
Seebeck Effect
The Seebeck effect is characterized by the generation of an electromotive force (EMF) when there is a temperature difference between two different conductive materials. This phenomenon is vital as it provides a basis for thermoelectric generators, which can convert waste heat into electricity. One significant characteristic of the Seebeck effect is its ability to operate in diverse environments, allowing for energy harvesting in various applications ranging from industrial processes to automotive technologies.
Its major benefit is the reduction of energy waste by utilizing heat that would otherwise be lost. However, the efficiency of the Seebeck effect in converting thermal energy into electrical energy is often limited, which poses a challenge for its widespread use in some designs.
Peltier Effect
The Peltier effect differs from the Seebeck effect; it entails the heating or cooling at the junction of two different conductors when an electric current is applied. This aspect is particularly beneficial in applications requiring precise temperature control, such as in electronic devices and refrigeration systems. The characteristic aspect of the Peltier effect is its capability to create significant temperature differences in compact formats, allowing for versatile cooling solutions.
Nevertheless, like the Seebeck effect, the Peltier effect faces challenges with efficiency. It tends to consume considerable electrical power relative to the cooling output it provides, leading to ongoing research aimed at enhancing its performance in practical applications.
Heat Engines
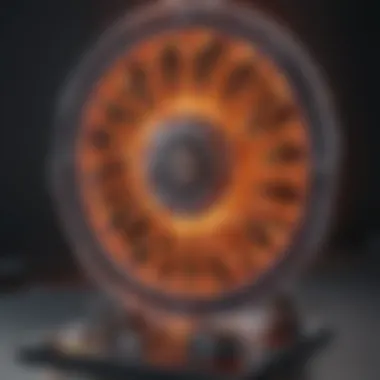
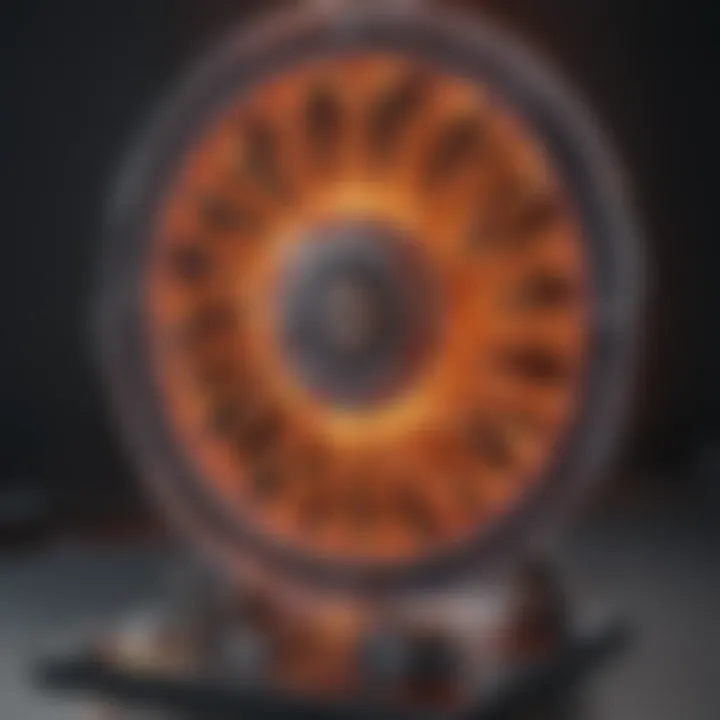
Heat engines play a crucial role by converting thermal energy into mechanical or electrical energy, bridging the realms of heat and electricity. They come in various types, each suited for specific applications. The understanding of heat engines is vital for innovation in technology and energy generation.
Types of Heat Engines
Types of heat engines include internal combustion engines, steam engines, and gas turbines. Internal combustion engines are prevalent in vehicles, converting fuel into motion through controlled explosions. Steam engines, traditionally used in locomotives, employ steam produced from boiling water, converting heat into mechanical work. On the other hand, gas turbines are used extensively in power plants, generating electricity efficiently from combustion gases.
Every type of heat engine has its unique features, providing different advantages like power density or operational flexibility. However, they also come with disadvantages, such as emissions for some models, necessitating continuous improvements in their designs.
Efficiency Considerations
Efficiency considerations are integral to the assessment and design of heat engines. The ability of a heat engine to convert heat energy into usable work directly influences its performance and viability. The thermal efficiency of an engine is typically expressed as the ratio of work output to heat input.
Improving efficiency is vital as it increases energy output while minimizing fuel consumption and emissions. A high-efficiency engine is not just cost-effective but also contributes to sustainable practices. However, achieving high efficiency in real-world applications is complex and requires innovative engineering solutions.
Continual innovation and research in conversion processes will drive future advancements in energy technology, addressing critical challenges related to efficiency and sustainability.
Practical Applications
The practical applications of heat and electricity are crucial for understanding how these forms of energy interact in real-world scenarios. The ways in which they are harnessed can significantly impact efficiency, sustainability, and innovation. By examining specific applications such as thermal power plants and renewable energy systems, we can glean insights into effective energy management strategies and their implications for future developments.
Thermal Power Plants
Operation Principles
Thermal power plants convert heat energy into electrical energy using various fuels such as coal, natural gas, or nuclear fission. The operation principles hinge on the use of a heat engine, where thermal energy is transformed to mechanical energy, which then generates electricity. The key characteristic of thermal power plants is their ability to produce large amounts of power consistently. This reliability makes them a popular choice in the energy sector.
One unique feature of these plants is the concept of steam generation. Water is heated to create steam, which drives turbines connected to generators. This process has several advantages, including scalability and established technology. However, it also brings disadvantages, such as greenhouse gas emissions and high water usage, which are concerns in discussions of environmental sustainability.
Environmental Impact
The environmental impact of thermal power plants is significant and often debated. The specific aspect of emissions from burning fossil fuels cannot be overlooked. These plants are major sources of carbon dioxide and other pollutants, which contribute to climate change and local air quality issues. The key characteristic here is the balance between energy output and environmental cost. This impact makes thermal power plants a controversial yet necessary component in the current energy landscape.
While they provide essential energy, one unique feature is the increasing adoption of carbon capture and storage technologies. These technologies aim to mitigate emissions and enhance sustainability. However, these solutions can sometimes lead to higher operational costs, complicating the economic viability of thermal power plants in the long term.
Renewable Energy Systems
Solar Thermal Energy
Solar thermal energy harnesses sunlight to produce heat that can be converted into electricity or used directly for heating applications. This form of energy is especially relevant as it aligns with global efforts to shift towards more sustainable energy sources. The specific aspect of solar thermal systems is their ability to provide a renewable and inexhaustible source of energy. The key characteristic of solar thermal energy is its efficiency in converting sunlight into usable heat, which makes it a beneficial choice for both residential and industrial applications.
A unique feature is the use of concentrated solar power technology. This involves using mirrors to focus sunlight, thereby generating steam to drive turbines, resulting in electricity generation. Although solar thermal systems offer great potential to reduce reliance on fossil fuels, they also have some disadvantages, such as high setup costs and dependence on sunlight availability.
Geothermal Energy
Geothermal energy refers to the heat sourced from the Earthβs interior. It is a steady and reliable energy source that can be utilized for electricity production and for direct heating. The specific aspect of geothermal energy is its low emissions profile compared to traditional fossil fuel sources. This makes it an attractive option in discussions about sustainable energy. The key characteristic of geothermal systems is their ability to provide a constant supply of energy, making them a reliable choice in regions where geothermal resources are available.
A unique feature of geothermal systems is their minimal land footprint compared to solar or wind energy systems, which can lead to less environmental disruption. However, one of the disadvantages includes the limited geographical areas where geothermal energy is viable, which may affect its widespread adoption in some regions. Overall, geothermal energy presents both challenges and opportunities for the energy sector moving forward.
Recent Advancements
Recent advancements in the field of heat and electricity highlight significant strides in technology and research. These improvements can enhance energy efficiency, reduce environmental impact, and provide practical applications for various industries. By focusing on innovative materials and systems, researchers aim to develop more effective methods of energy conversion and management.
One major area of progress is the development of innovative thermoelectric materials. These materials can directly convert heat into electrical energy through thermoelectric effects. Their importance lies in their potential for enhancing the performance of devices that rely on heat transfer and generation. Improved thermoelectric materials promise not only increased efficiency but also the ability to harness waste heat from industrial processes, making energy systems more sustainable.
Another crucial advancement is the emergence of smart energy management systems. These systems leverage advances in technology to optimize the distribution and consumption of energy. By using real-time data and machine learning algorithms, they can analyze energy usage patterns and adjust operations for maximum efficiency. This not only lowers energy bills but also contributes to minimizing the carbon footprint of residential and commercial buildings.
Smart energy management systems exemplify how integrated technologies can lead to substantial energy savings.
The importance of recent advancements in heat and electricity cannot be overstated. They represent a blend of innovation and practical application that is vital for addressing current and future energy challenges. As industries strive for sustainability, these developments will play a pivotal role in achieving cleaner and more efficient energy solutions.
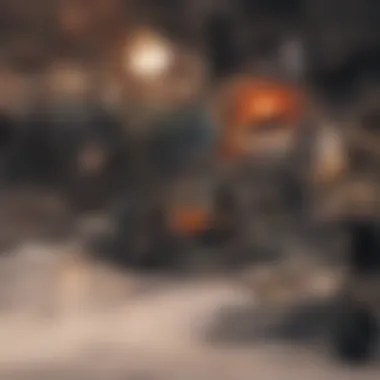
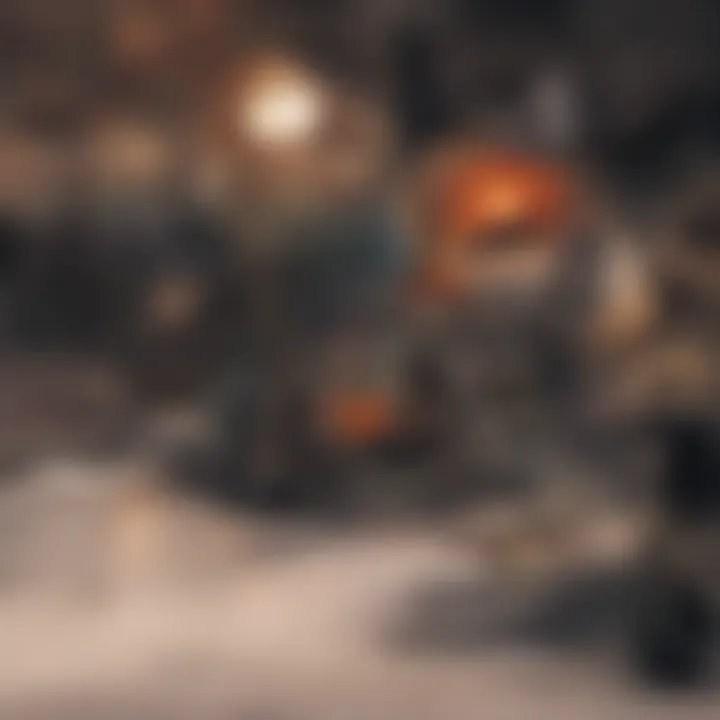
Innovative Thermoelectric Materials
Innovative thermoelectric materials are at the forefront of research and development in energy conversion. These materials exhibit unique properties that enable them to convert temperature differences directly into electric voltage. The efficiency of these materials is measured by their dimensionless figure of merit, denoted as ZT. A higher ZT value indicates better performance and greater efficiency.
Recent research has focused on developing advanced materials such as bismuth telluride and half-Heusler compounds. These materials show promise due to their favorable thermoelectric properties and the ability to operate over a range of temperatures. Researchers are also exploring nanostructuring techniques to enhance the thermoelectric performance by reducing thermal conductivity while maintaining electrical conductivity.
In the context of practical applications, innovative thermoelectric materials can be utilized in various technologies:
- Waste Heat Recovery: Capturing excess heat from industrial processes to generate electricity.
- Cooling Devices: Thermoelectric modules can provide cooling for electronic components without moving parts.
- Portable Power Generation: Small-scale devices can convert heat from campfires or engines into usable power.
These materials open new avenues for sustainable energy. The continued research in this area holds the potential for breakthroughs that can profoundly impact energy systems and reduce reliance on fossil fuels.
Smart Energy Management Systems
Smart energy management systems represent a critical evolution in how energy is monitored and utilized. These systems use connected devices and advanced analytics to improve the efficiency of energy use in buildings and industrial settings. One key aspect of these systems is their ability to provide real-time monitoring of energy consumption.
The integration of the Internet of Things (IoT) technology allows sensors to collect data on energy use patterns and environmental conditions. This information, when analyzed, can reveal opportunities for energy savings. For instance, smart thermostats can automatically adjust heating and cooling based on occupancy and preferences, maintaining comfort while reducing energy costs.
Benefits of smart energy management systems include:
- Enhanced Energy Efficiency: Optimized energy use leads to lower utility costs.
- Data-Driven Decision Making: Access to detailed energy data empowers users to make informed decisions regarding consumption.
- Environmental Impact Reduction: By minimizing energy waste, these systems contribute to lower greenhouse gas emissions.
Challenges and Future Directions
The interplay between heat and electricity presents numerous challenges and opportunities for advancement in technology and sustainability. In this section, we will explore the obstacles faced in efficiency improvements and sustainability considerations, with an aim to outline future directions in research and practical applications.
Efficiency Improvements
Enhancing the efficiency of systems that convert heat to electricity and vice versa is critical. Heat loss is a primary issue that affects overall performance. Current technologies, including thermoelectric materials and heat engines, often operate at suboptimal efficiencies. Therefore, exploring new materials and improving existing processes is vital.
Recent research has focused on advanced thermoelectric materials, which can offer better performance. Materials such as bismuth telluride are traditionally used, but newer compositions like tin selenide show promise for higher efficiency. Moreover, it is essential to develop processes that minimize heat dissipation during conversion. This includes innovations in thermal insulation and active cooling techniques.
"The pursuit of efficiency in energy conversion is not just a technical challenge; it is a necessity for sustainable energy systems."
In addition, adopting smart energy management systems can greatly improve efficiency. These systems use algorithms to optimize energy flows, thus allowing for better use of available heat and electricity. By implementing real-time data analysis, users can reduce waste and maximize output.
The integration of these technologies requires significant investment and research. Collaboration among institutions, industries, and governments is necessary to create a framework that supports such innovations.
Sustainability Considerations
Sustainability is a crucial element when considering the future directions of heat and electricity dynamics. Climate change and finite resources demand that energy systems not only become more efficient but also adopt sustainable practices. This involves transitioning to renewable energy sources, such as solar, wind, and geothermal, which can provide heat and electricity without adversely impacting the environment.
Choosing sustainable materials for thermoelectric applications is part of this conversation. Many traditional materials have environmental drawbacks, such as rare earth metals that require extensive mining. Researching and developing alternative materials is essential. Materials that are abundant, recyclable, and have lower environmental impacts should be prioritized.
Furthermore, lifecycle assessments of energy systems must consider long-term impacts. These analyses can inform decisions about which technologies to adopt or improve. For example, exploring the full environmental cost of thermoelectric generators from production to disposal is vital in ensuring truly sustainable solutions.
Encouraging policies and enriching public knowledge about energy consumption also play pivotal roles in promoting sustainability. Governments can facilitate the transition by implementing regulations that encourage renewable energy use, funding research for cleaner technologies, and providing incentives for industries that adopt efficient systems.
End
The conclusion of this article emphasizes the intricate relationship between heat and electricity. This connection is vital for not only understanding fundamental physical processes but also for practical applications across various industries. The dynamic interplay between these two forms of energy governs advancements in technology and energy efficiency, while having significant implications for sustainability.
Summary of Key Points
This article discussed several essential themes:
- Theoretical Foundations: Detailed concepts such as thermodynamics and electromagnetic theory support our understanding of heat and electricity.
- Conversion Processes: The thermoelectric effect and heat engines illustrate how energy can be transformed from one form to another.
- Practical Applications: Real-world systems like thermal power plants and renewable energy sources demonstrate the significance of this interplay in energy production.
- Recent Advancements: Innovations in thermoelectric materials and smart energy management systems highlight ongoing developments in the realization of efficient energy systems.
- Challenges and Future Directions: Important issues surrounding efficiency improvements and sustainability considerations are crucial for future progress.
Implications for Future Research
The exploration of heat and electricity reveals significant pathways for future research. Scholars and practitioners should focus on:
- Innovative Technologies: Developing new materials and methods to improve thermoelectric efficiency can lead to groundbreaking applications in energy conversion.
- Sustainable Practices: Research on integrating renewable energy sources with existing systems can enhance sustainability efforts ground across multiple sectors.
- Interdisciplinary Approaches: Collaboration across fields such as physics, engineering, and environmental science can result in more comprehensive solutions to energy challenges.
The relationship between heat and electricity holds untapped potential and warrants continued investigation to foster innovations that can address current and future energy needs.