Exploring MPI Magnetic Technology: Principles and Uses
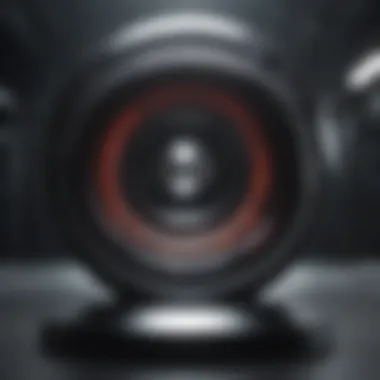
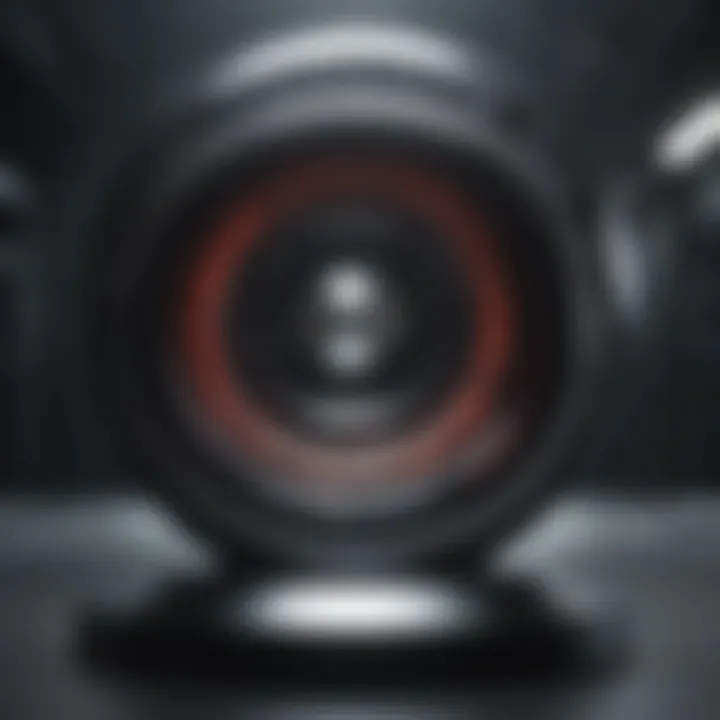
Intro
Magnetic Particle Imaging (MPI) is an emerging imaging modality that utilizes the properties of magnetic nanoparticles to create high-resolution images. As such, it represents a significant advancement in the fields of medical imaging and research. This technique offers several advantages over traditional imaging modalities due to its ability to provide real-time imaging with high spatial resolution and minimal background noise. Understanding the fundamentals of MPI magnetic technology is crucial for researchers, as it opens pathways for innovative applications in various scientific disciplines.
In this article, we will explore the core concepts of MPI, delve into its historical development, and examine its multifaceted applications across different fields. By taking a closer look at the principles, findings, and potential future trends within MPI magnetic technology, we aim to enhance the understanding of this significant topic.
Research Background
Overview of the Scientific Problem Addressed
In the realm of medical imaging, traditional techniques such as MRI and X-rays have limitations, particularly concerning resolution and speed. The challenge lies in balancing image quality with safety and efficiency. MPI magnetic technology seeks to address these shortcomings by offering a novel imaging solution that can operate within the confines of safety while providing real-time data.
Historical Context and Previous Studies
The concept of using magnetic nanoparticles for imaging is not entirely new. Research has progressed significantly over the past few decades. Initial studies focused on understanding the properties of magnetic materials and their potential applications. In 2005, the first groundwork for MPI was laid by researchers who sought a system that could exploit the unique characteristics of superparamagnetic nanoparticles. This paved the way for subsequent research, including technological advancements in hardware and software used in MPI systems.
Intro to MPI Magnetic
Understanding MPI magnetic technology is crucial in both scientific and practical contexts. It revolutionizes how we visualize and interpret magnetic fields through innovative imaging techniques. MPI stands for Magnetic Particle Imaging. This technology marks a significant advancement in how we approach imaging in various fields, notably in biomedical applications.
The importance of this topic lies in its potential to enhance image resolution and provide real-time data visualization. MPI's unique ability to utilize magnetic nanoparticles offers improved sensitivity compared to traditional imaging techniques, such as MRI. This article aims to dissect these concepts and their applications while also highlighting its implications in research and industry. Through a systematic evaluation, readers can appreciate the advancements and challenges MPI magnetic technology presents.
Definition of MPI Magnetic
MPI magnetic refers to a cutting-edge imaging technology that uses magnetic fields to visualize the distribution of magnetic nanoparticles in a given environment. The principle behind MPI is based on the nonlinear response of these nanoparticles to magnetic fields. This technology enables scientists and researchers to generate high-resolution images while ensuring a non-invasive approach in various applications, especially in medicine.
Historical Context
Early Developments
The inception of MPI magnetic technology can be traced back to significant advancements in magnetic nanoparticle research. Early developments focused on understanding how these particles interact with magnetic fields. Initial experiments laid the groundwork for potential applications in imaging, showcasing unique characteristics of how magnetic nanoparticles can be manipulated for imaging purposes.
The key characteristic of these early developments was the innovation in synthesis techniques of magnetic nanoparticles. These techniques allowed for better control over particle size and coating, which are vital for optimizing their imaging performance. The exploration into early developments is essential because it illustrates the starting point for more complex implementations of MPI technology today.
Advantages of these early studies include a clear understanding of magnetic field behavior, which became fundamental in further research. However, they were limited by technological constraints, which restricted their application and efficiency in imaging systems.
Pioneering Research
Pioneering research into MPI magnetic took significant leaps forward as scientists began to grasp the potential of using magnetic fields for imaging at a cellular level. This research explored not only the physical properties of magnetic nanoparticles but also the biological implications of their use. One of the remarkable characteristics of this phase was the shift towards practical applications in medicine, with an increased focus on using MPI for imaging living organisms.
This research phase became notable for its interdisciplinary nature, bridging physics, biology, and engineering. It was a beneficial choice for the field as it provided unique insights into optimizing MPI technology for clinical use. The unique feature here is the adaptability of MPI techniques to integrate with existing imaging technologies, which positions it as a complementary tool in radiology.
While the advantages of this pioneering research include enhanced imaging capabilities and real-time visualization, challenges persisted in terms of regulatory approval and the need for standardization in protocols. Nonetheless, the groundwork laid during these research phases continues to influence current developments in MPI technology.
Fundamental Principles of MPI
Understanding the fundamental principles of Magnetic Particle Imaging (MPI) is crucial for delving into its applications and advantages. This section elucidates how MPI operates, emphasizing the importance of magnetic fields and their interactions with particles. The principles are the backbone of MPI technology, influencing its design, functionality, and utility in various fields like medicine and industry.
Magnetic Fields and Particle Behavior
Magnetic fields play a pivotal role in MPI. They dictate how magnetic nanoparticles interact with each other and with external stimuli. The behavior of these particles under the influence of a magnetic field leads to different imaging characteristics, making it essential for accurate data acquisition.
The strength of the magnetic field affects the resolution and sensitivity of the imaging. Stronger fields can enhance the clarity of images by improving the signal-to-noise ratio. This quality makes MPI a preferred method in many applications, including biomedical imaging where precise details are needed.
Imaging Techniques
Imaging techniques in MPI comprise several methods that help obtain and process image data efficiently. Two key components of this are data acquisition methods and signal processing. Each method contributes distinctively to achieving the desired outcomes in MPI.
Data Acquisition Methods
Data acquisition in MPI is critical as it involves capturing the data necessary to reconstruct an image. One key characteristic of these methods is their ability to gather high-resolution data quickly. This efficiency is particularly beneficial when imaging dynamic biological processes, such as blood flow in real-time.
One unique feature of data acquisition methods in MPI is the use of specialized detectors that can discern subtle differences in magnetic signal. This allows for superior imaging capabilities compared to conventional imaging techniques. However, a disadvantage may arise from the technology's reliance on expensive equipment and sophisticated setups, which can limit accessibility for some researchers or institutions.
Signal Processing
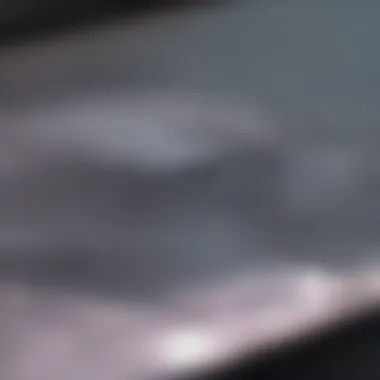
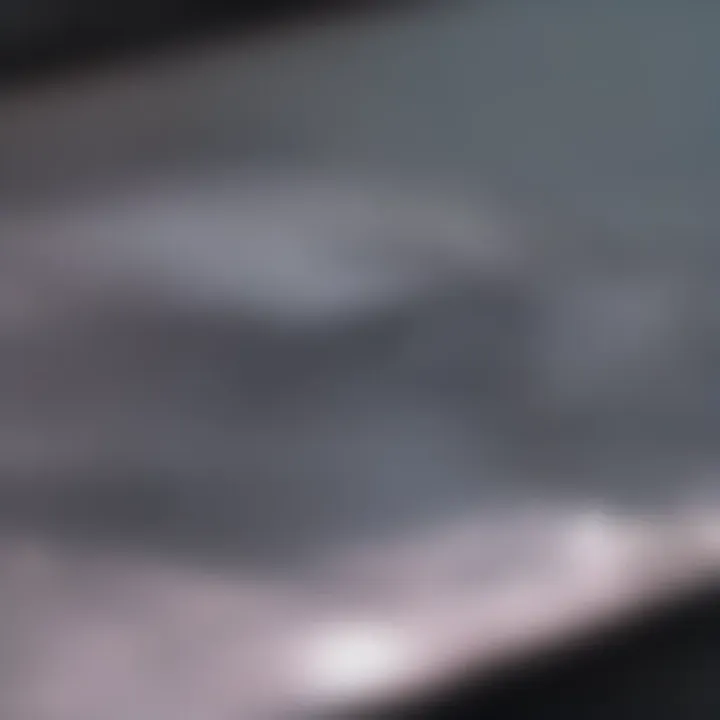
Signal processing in MPI plays a crucial role in interpreting the acquired data. It focuses on transforming raw magnetic signals into clear, interpretable images. A key characteristic of signal processing in MPI is its ability to filter out noise and enhance signal quality, ensuring that the resulting images are of high fidelity.
A unique feature of signal processing techniques lies in their adaptability. They can be customized based on the specific imaging requirements of a study or application. Nonetheless, these processing methods can be complex and may require expertise in advanced algorithms, posing a barrier for new users entering the field.
In summary, the fundamental principles of MPI underpin its effectiveness and versatility. The interplay between magnetic fields and particles, along with sophisticated imaging techniques like data acquisition methods and signal processing, illustrates the intricacies of this technology. Understanding these principles is key to leveraging MPI for future advancements across various disciplines.
Materials Used in MPI Magnetic Technology
Understanding the materials used in MPI magnetic technology is crucial for comprehending its capabilities and applications. The selection of appropriate materials directly influences the performance of MPI systems in imaging and detection tasks. The right materials ensure that the MPI technique can effectively produce high-resolution images and reliable data in a non-invasive manner. Moreover, the ongoing advancements in material science continue to open new avenues for improving MPI applications across various fields.
Magnetic Nanoparticles
Magnetic nanoparticles are at the core of MPI technology. Their unique properties make them essential for various biomedical and industrial applications. The size, shape, and magnetic characteristics of these particles contribute significantly to the sensitivity and spatial resolution potential of MPI systems.
Synthesis Techniques
The synthesis techniques for magnetic nanoparticles play a determining role in the quality and characteristics of the produced materials. Common methods include co-precipitation, solvothermal, and hydrothermal processes. Each technique contributes differently to the final product's attributes. For instance, the co-precipitation method is popular because it is relatively cost-effective and straightforward. It allows for the production of nanoparticles with controlled size and magnetic properties, essential for achieving desired performance in MPI.
One unique feature of the solvothermal synthesis technique is its ability to produce nanoparticles with uniform size and narrow distribution. This uniformity is vital for enhancing the imaging quality in MPI applications. However, this method can also be more complex and require specific temperature and pressure conditions, which may be a disadvantage for some researchers.
Characterization Methods
Characterization methods are pivotal for verifying the physical and chemical properties of magnetic nanoparticles. Techniques like scanning electron microscopy (SEM) and dynamic light scattering (DLS) are routinely used to assess particle size, morphology, and surface properties. Proper characterization ensures that the nanoparticles will perform adequately in MPI applications.
A key characteristic of these methods is their ability to provide detailed insight into the nanoparticlesβ properties. This level of understanding is essential, as it helps in tailoring the materials to specific MPI requirements. However, these techniques can be time-consuming and may require expensive equipment, limiting accessibility for some researchers or institutions.
Coatings and Surface Modifications
The role of coatings and surface modifications cannot be overlooked in MPI magnetic technology. These modifications enhance the stability and biocompatibility of magnetic nanoparticles for biomedical applications. Adjusting surface properties allows for better targeting and interaction with biological tissues.
Coatings can also reduce aggregation and improve dispersibility in biological environments, which is critical for achieving effective imaging results. Utilizing biocompatible materials for coatings can lead to improved safety in clinical imaging. However, choosing the appropriate coating material requires careful consideration of the intended application to balance performance and stability.
Applications of MPI Magnetic
The applications of MPI magnetic technology encompass a broad spectrum of fields, each demonstrating its unique utility and benefits. As scientific disciplines continue to evolve, so does the significance of MPI. This technology shows great potential not just in biomedical imaging but also in various industrial sectors. Here, we will explore two primary application areas: biomedical imaging and industrial applications, along with their specific aspects, benefits, and considerations.
Biomedical Imaging
In the realm of healthcare, the demand for advanced imaging techniques is perpetually increasing. MPI magnetic technology brings forth innovative solutions that address current limitations found in conventional methods.
Advantages over Conventional Techniques
MRI and CT scans have been the gold standards in medical imaging for years. However, MPI magnetic technology presents several advantages that render it a compelling choice. Notably, its spatial resolution surpasses that of these conventional techniques. The fundamental aspect of MPI is its ability to provide detailed images with high sensitivity and minimal background noise. This precision allows for better differentiation of tissue types, enhancing diagnostic accuracy.
One key characteristic of MPI is its real-time imaging capability. Unlike traditional methods, which often require long acquisition times, MPI can rapidly capture images, providing instant feedback. This feature is particularly beneficial in emergency settings, where every second matters. Another unique feature of MPI is its non-invasive nature. It utilizes magnetic fields rather than ionizing radiation, minimizing risk to patients, which is an essential consideration in clinical practice.
However, while advantageous, MPI is still developing. Its adoption is limited by the availability of specific magnetic nanoparticles and imaging systems. Still, the prospect of transitioning from conventional methods to MPI is highly promising for future medical imaging.
Clinical Case Studies
To further grasp the impact of MPI in biomedical imaging, examining clinical case studies is indispensable. These studies not only illustrate the practical applications of MPI but also highlight its efficacy.
A significant feature of clinical case studies in this context is the focus on real-world scenarios where MPI outperformed other imaging modalities. Results from these studies have shown enhanced tumor detection rates and improved visualization of vascular structures. Such results advocate for the integration of MPI into standard imaging practices.
Moreover, these studies often report on multi-modal imaging approaches, where MPI is combined with other techniques like PET or ultrasound. This collaborative methodology enhances diagnostic capabilities, making it a popular choice among researchers and clinicians alike.
Despite the promising results, challenges exist, especially regarding standardization and regulatory approval of MPI devices. More comprehensive studies are necessary to fully assess the long-term benefits and limitations of MPI in clinical settings.
Industrial Applications
The industrial sector is another significant domain where MPI magnetic technology shines. Various applications exist, particularly concerning quality control and non-destructive testing.
Quality Control in Manufacturing
In manufacturing processes, MPI technology plays a critical role in quality assurance. This application is essential to ensure products meet safety and performance standards. The unique aspect of MPI in quality control is its capability to detect even minute defects within products without compromising their integrity.
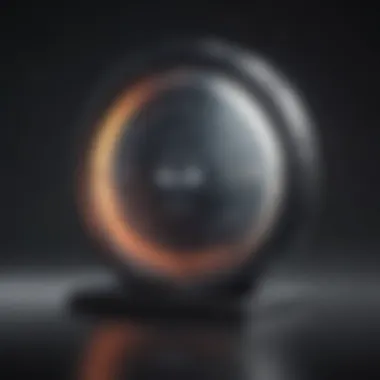
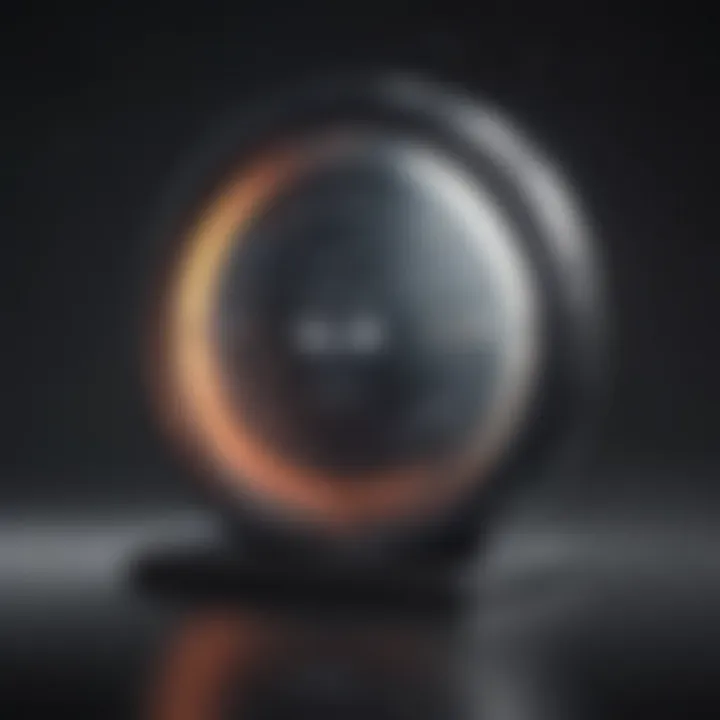
Furthermore, the real-time imaging characteristic of MPI enables manufacturers to monitor production lines continuously. This monitoring ensures that any issues can be addressed immediately, reducing waste and cost. Manufacturing settings that adapt MPI can enjoy increased throughput, as fewer resources are spent on inspections.
However, the deployment of MPI for quality control is not without its challenges. The initial investment in MPI technology and the requirement for trained personnel can deter some organizations. Still, the long-term benefits of increased efficiency and product reliability often outweigh these initial hurdles.
Non-Destructive Testing
Non-destructive testing (NDT) is integral to ensuring the safety and reliability of structures and materials. MPI magnetic technology provides a unique approach to NDT, enabling inspectors to assess materials without causing harm or alteration.
One key characteristic of MPI in NDT applications is its sensitivity to internal defects, such as cracks and voids. The ability to visualize these issues prior to failure is invaluable across numerous industries, including aerospace, automotive, and construction. As a result, MPI becomes a beneficial choice for ensuring operational safety and enhancing maintenance protocols.
The unique feature of MPI technology in this application is its capacity for rapid and accurate inspections. Traditional NDT methods can be time-consuming and labor-intensive, whereas MPI provides faster results. This efficiency is particularly crucial in high-demand sectors where downtime can be costly.
Nonetheless, just like with quality control, the integration of MPI into standard NDT practices requires overcoming specific barriers. Ensuring trained personnel and designing suitable operational protocols are critical to the successful implementation of MPI in this context.
MPI magnetic technology offers unique benefits across various applications, enhancing imaging capabilities and quality control, but it is essential to consider limitations and integration challenges.
Advantages of MPI Magnetic Technology
The benefits of MPI magnetic technology are significant and multifaceted. This section explores the various advantages it offers in comparison to traditional imaging methods. The impact of these advantages is crucial for researchers and professionals working in fields related to imaging and diagnostics.
Spatial Resolution and Sensitivity
Spatial resolution refers to the ability of an imaging system to distinguish between two closely spaced points. In the context of MPI technology, this is one of its standout features. MPI can achieve exceptionally high spatial resolution, which is vital in applications like biomedical imaging. The capability to discern small structures can greatly enhance diagnostic accuracy.
Additionally, sensitivity is another key element where MPI excels. This technology allows for the detection of low concentrations of magnetic nanoparticles. The high sensitivity levels can result in earlier detection of diseases, leading to better patient outcomes. In clinical settings, such advantages are paramount as they can influence treatment decisions.
Key points regarding spatial resolution and sensitivity include:
- Enhanced visualization of small anatomical structures.
- Improved detection rates for early-stage diseases.
- Potential for less invasive procedures due to accurate targeting.
In summary, the precision in both spatial resolution and sensitivity makes MPI magnetic technology a powerful tool in imaging.
Safety and Non-Invasiveness
Another significant advantage of MPI is its safety and non-invasiveness. Traditional imaging techniques often involve exposure to ionizing radiation or contrast agents that can have adverse effects. In contrast, MPI utilizes magnetic nanoparticles that are considered safe for human use. This characteristic minimizes risks associated with imaging procedures, making MPI especially appealing in sensitive populations, such as children and elderly patients.
The non-invasive nature of MPI plays a pivotal role in encouraging its adoption in clinical settings. Non-invasive procedures typically result in quicker recovery times and lower patient anxiety levels. This can enhance the overall experience of patients undergoing diagnostic processes.
Aspects to note about safety and non-invasiveness include:
- Reduced risk of adverse reactions from contrast agents.
- Lower long-term health risks associated with repeated imaging.
- Improved patient acceptance of imaging procedures.
The safety and non-invasiveness of MPI technology positions it as a frontrunner in modern imaging solutions.
Challenges in MPI Magnetic
The exploration of MPI magnetic technology reveals essential challenges that can hinder its widespread adoption and efficiency. Addressing these challenges is critical for researchers and practitioners looking to implement MPI in both biomedical and industrial settings. By understanding the technical limitations and market acceptance, stakeholders can devise strategies to overcome obstacles and improve the technology's implementation.
Technical Limitations
Technical limitations are among the most significant barriers to the progress of MPI magnetic technology. These limitations can be categorized into hardware constraints and software challenges, each playing a vital role in the overall effectiveness of the technology.
Hardware Constraints
Hardware constraints involve issues related to the physical components of MPI systems. Currently, the main limitation stems from the requirements of high-quality magnetic field production. An inherent challenge is the need for sophisticated coil designs to generate the magnetic fields necessary for effective imaging. Additionally, high-resolution detectors are expensive and complex.
This specificity fosters a key characteristic of hardware in MPI systems: customization. Custom-built hardware can optimize image quality, but this often results in a higher cost and longer development times.
"The cost of high-tech hardware in MPI can be a significant barrier for smaller institutions, limiting access to cutting-edge imaging technology."
Unique features of these constraints include the integration of various components that must work seamlessly together. While tailored solutions can enhance performance, they also introduce challenges in maintenance and repair, which can further complicate operations.
Software Challenges
Software challenges in MPI magnetic technology are significant as they pertain to data acquisition, processing, and analysis. The complexity of signals produced during imaging demands robust software solutions for real-time data interpretation. Many existing systems struggle to adapt effectively to different types of imaging scenarios and user needs.
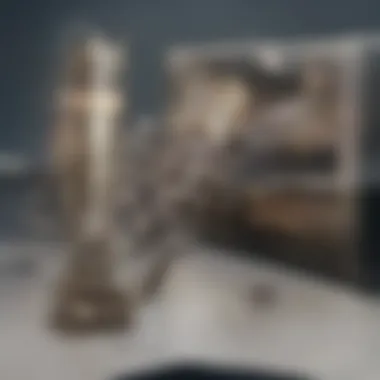
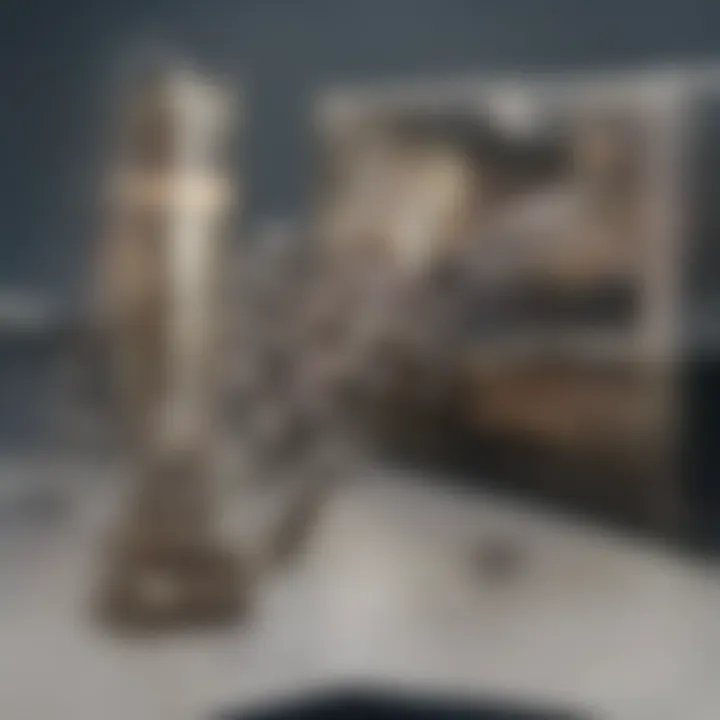
A distinct feature of software in this context is the reliance on advanced algorithms for signal reconstruction. While these algorithms can drastically improve image clarity and spatial resolution, they also require constant updates and modifications to keep up with evolving technologies.
The dynamic nature of software development in MPI systems creates a beneficial yet challenging landscape. Software must remain versatile to cater to various applications, yet also powerful enough to manage high volumes of data efficiently. As a result, there is a significant need for skilled professionals who can navigate these complexities.
Market Acceptance
Market acceptance of MPI technology involves the willingness of institutions and industries to adopt it as part of their imaging repertoire. Several factors contribute to market hesitancy, including cost considerations, awareness of benefits over existing technologies, and the overall maturity of MPI technology.
Additionally, regulatory hurdles and the need for clinical validation can deter potential users. For MPI technology to see broader acceptance, stakeholders must demonstrate clear advantages compared to conventional imaging methods.
In summary, addressing the identified challenges in MPI magnetic technology is vital for fostering its acceptance and practical application. As researchers continue solving technical limitations and promoting its benefits, the potential for MPI in various fields will likely expand.
Future Directions in MPI Magnetic Research
The future of MPI magnetic research is pivotal for advancing imaging techniques and applications in various disciplines. As technology evolves, exploring innovative directions ensures that MPI maintains its relevance and enhances its effectiveness. Understanding these future routes helps researchers and practitioners assess the potential of MPI technology. This section will explore innovative approaches and the potential for market expansions as critical components of MPI's advancement.
Innovative Approaches
Emerging Technologies
Emerging technologies play a significant role in the progression of MPI magnetic systems. These technologies often bring new methodologies, instruments, and techniques that can drastically improve existing practices. One key characteristic of these technologies is their potential for improved sensitivity and resolution. This can lead to clearer images and more accurate diagnostics.
These innovations typically involve the development of upgraded hardware, new algorithm solutions, or refined imaging strategies. For example, advancements in magnetic nanoparticle design can enhance the stability and efficacy of MPI. The unique feature of these emerging technologies is their ability to reduce imaging times while maintaining or increasing the quality of data captured.
However, adopting these technologies can be challenging. Costs related to development and implementation can be high, and technical expertise is often required to fully utilize these advanced systems. Nonetheless, the long-term benefits in diagnostics and imaging are convincing arguments for their exploration.
Cross-Disciplinary Collaborations
Cross-disciplinary collaborations are a critical aspect of advancing MPI magnetic research. This entails partnerships between fields such as physics, engineering, and biomedical sciences. The key characteristic of such collaborations is the diversification of expertise. By pooling knowledge from different disciplines, researchers can approach MPI from various angles, which leads to more robust and innovative solutions.
An example of cross-disciplinary work could involve material scientists and medical researchers collaborating to develop new types of magnetic nanoparticles specifically for biomedical imaging. The unique feature of these collaborations is the ability to combine different skill sets, fostering creativity and innovation.
However, coordination between disciplines can be difficult. Differences in terminology, objectives, and methodologies can lead to misunderstandings. In spite of these challenges, the mutual benefits of shared knowledge often outweigh the potential downsides, leading to remarkable advancements in MPI technology.
Potential Market Expansions
New Applications in Healthcare
New applications in healthcare represent a substantial opportunity for MPI magnetic technology. As the demand for non-invasive and precise imaging grows, the healthcare sector increasingly looks for advanced imaging solutions. The key characteristic of these applications is their ability to provide detailed insights without harming patients.
Potential unique applications include using MPI in early cancer detection or monitoring the effectiveness of treatments. The advantages of these applications stem from MPI's high sensitivity and spatial resolution, which can lead to better patient outcomes and more informed clinical decisions.
However, new applications must undergo stringent testing and validation before widespread adoption. The regulatory landscape in healthcare can create barriers, slowing down the introduction of new technologies. Nonetheless, the potential benefits make this a critical area for future research and development.
Integration with Other Imaging Techniques
Integration with other imaging techniques is another important aspect of MPI's potential market expansion. This involves combining MPI with established methods like MRI or CT scans to boost overall diagnostic capabilities. The main characteristic of this approach is synergistic enhancement, where the strengths of one imaging modality complement those of another.
For instance, MPI could offer real-time imaging capabilities that traditional methods lack, while MRI provides excellent soft tissue contrast. The unique feature of this integration is its potential to create comprehensive imaging systems that can lead to improved diagnostic accuracy.
One disadvantage may be the complexity of combining different technologies. Systems may require extensive re-engineering to allow for seamless operation. Integration also requires significant cross-disciplinary effort. Despite these challenges, integrating MPI with existing methods could pave the way for significant advancements in medical imaging.
Ending
The conclusion serves as a vital component of this article as it encapsulates key takeaways and introspects on the broader implications of MPI magnetic technology. Summarizing insights from earlier sections reinforces the importance of understanding both the capabilities and limitations of this innovative imaging method. It encourages readers, particularly students, researchers, educators, and professionals, to reflect on the contents and consider how MPI magnetic can influence their respective fields.
In summation, the MPI magnetic technology presents unique advantages for biomedical imaging and industrial applications, offering enhanced spatial resolution and non-invasive procedures. As noted, the challenges perhaps create barriers to entry in broader markets, but the commitment to innovative approaches and cross-disciplinary collaborations could yield significant advancements. Thus, the conclusion not only synthesizes the information presented but also highlights considerations necessary for future exploration and appreciation of MPI magnetic.
Summary of Insights
This section succinctly highlights vital points discussed throughout the article. The journey begins with the definition and historical context of MPI magnetic, laying the foundation for understanding its fundamental principles and applications. Key insights include:
- Understanding the operational mechanisms that underpin magnetic particle imaging.
- The role of magnetic nanoparticles, which are crucial for improved imaging quality and applications in various domains.
- Innovations in imaging techniques enhancing data acquisition and signal processing, helping to overcome previous limitations.
- Notable advantages such as safety, non-invasiveness, and remarkable sensitivity in imaging.
- Challenges faced by researchers and practitioners, including technical constraints and issues with market acceptance.
- Future directions that can potentially reshape MPI, especially in healthcare settings and collaboration with other imaging techniques.
"The journey through MPI magnetic technology highlights both its capabilities and the considerations essential for its advancement."
Final Thoughts on MPI Magnetic
As we finalize this exploration, it is clear that MPI magnetic technology stands at a pivotal juncture. The ongoing research and development in this field must focus on addressing existing challenges, while leveraging its many advantages to forge new paths in both medical and industrial arenas.
The interdisciplinary nature of MPI magnetic creates a platform ripe for collaboration, inspiring researchers to integrate findings from various fields, leading to novel solutions for complex problems. As the community continues to investigate emerging technologies, the potential for MPI magnetic to transform conventional practices is tangible. It is crucial for stakeholders to remain informed and engaged, ensuring that advancements not only benefit academia but also translate effectively into real-world applications.