In Situ Ellipsometry: Principles and Applications
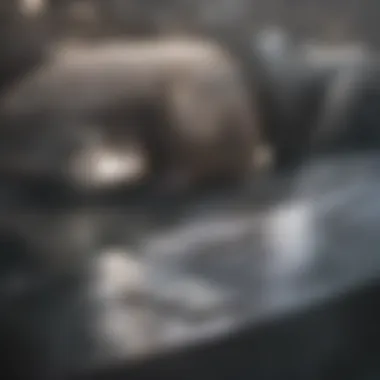
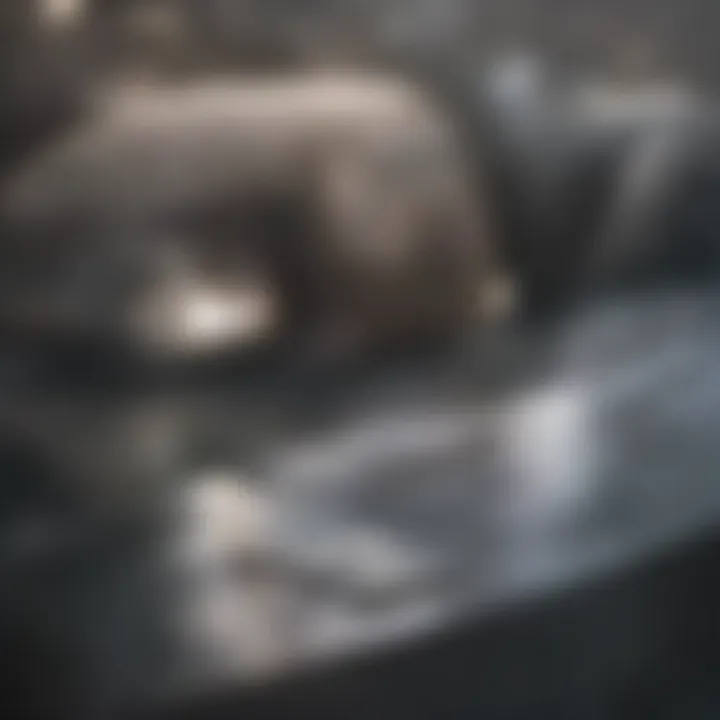
Intro
In situ ellipsometry is an advanced optical technique that plays a significant role in characterizing thin films and surfaces. This method is vital in many scientific fields, including material science, physics, and chemistry. By providing real-time data on film thickness, optical properties, and surface uniformity, it enables researchers to gain insights into material behavior during various processes.
The technique utilizes polarized light to measure the change in polarization as it reflects off a surface. The resulting data can reveal critical information about the materials being studied, such as their refractive index and absorption coefficient.
As research continues to evolve, understanding the principles and applications of in situ ellipsometry becomes increasingly essential for professionals and students alike.
Research Background
Overview of the Scientific Problem Addressed
Understanding thin films is crucial across many disciplines. These films often possess unique properties that differ from their bulk counterparts. This necessitates precise measurement techniques to assess their performance and reliability in applications such as semiconductors, optics, and coatings. Traditional methods, while useful, often lack the resolution or timeliness required to observe changes during processes like deposition or reaction. This is where in situ ellipsometry offers significant advantages.
Historical Context and Previous Studies
The origin of ellipsometry dates back to the early 19th century with the works of François Arago and later, Joseph von Fraunhofer. However, the adaptation of in situ measurements is a more recent development. The advancement in optics and computing has allowed for real-time analysis, pushing the boundaries of what can be achieved with this powerful technique. Previous studies focus on the application of ellipsometry in various fields. For example, in semiconductor fabrication, examining thin oxide layers can help improve device performance.
In the area of biological sciences, researchers use this method to study cell adhesion or protein layers. These investigations underline the versatile nature of in situ ellipsometry and its relevance in providing critical data that informs various scientific disciplines.
Findings and Discussion
Key Results of the Research
The findings show that in situ ellipsometry effectively monitors film growth and transitions. For instance, in the case of polymer films, real-time data have allowed researchers to evaluate how different environmental factors such as temperature and humidity affect film properties. Similarly, in semiconductor industries, continuous monitoring during manufacturing processes helps detect discrepancies early, minimizing waste and enhancing production efficiency.
Interpretation of the Findings
The ability to obtain immediate feedback is invaluable. This technology bridges the gap between laboratory settings and industrial applications. The real-time measurement provided by in situ ellipsometry helps achieve tighter process controls and better-quality materials. As industries strive for higher performance standards, this technique offers a pathway to meet those demands.
In situ ellipsometry not only enhances the understanding of material behavior but also informs the development of new materials and processes, firmly positioning it as a critical tool in modern scientific research.
Prologue to In Situ Ellipsometry
In situ ellipsometry is a sophisticated method that plays a critical role in the characterization of thin films and surfaces. In this section, we will explore the definition, historical context, and overall significance of this technique in research. Understanding in situ ellipsometry is essential for students, researchers, educators, and professionals who aim to delve into material characterization and surface science.
Definition and Overview
In situ ellipsometry utilizes polarized light to assess the optical properties of materials in real time. The technique stems from the principle of measuring changes in polarization as light reflects off a surface. This capability allows researchers to analyze films as they grow or change under various conditions. The information obtained can include film thickness, refractive index, and optical anisotropy. Its non-destructive nature makes it a preferred method in various scientific fields, from physics to biology.
Historical Context
The origins of ellipsometry can be traced back to the early 19th century. However, it was not until the mid-20th century that in situ ellipsometry gained traction. Initial developments centered on static measurements, which were limited in their applications. The shift towards dynamic, in situ techniques arose with advancements in laser technology and computer processing, enabling more accurate and rapid data acquisition. As scientists recognized the benefits of monitoring processes live, the technique found applications in semiconductor manufacturing, optics, and materials science.
Importance in Research
In situ ellipsometry plays a pivotal role in many research areas, offering unique advantages:
- Real-Time Monitoring: Researchers can observe processes as they happen, providing immediate insights into phenomena like film growth and chemical reactions.
- Non-Destructive Testing: The method does not alter the sample, making it suitable for valuable or fragile materials.
- Versatility: It is applicable across diverse fields, from assessing thin films in photovoltaic applications to studying biological systems.
Overall, in situ ellipsometry contributes to a deeper understanding of material interactions and behaviors, pushing the boundaries of scientific research.
Fundamental Principles of Ellipsometry
Understanding the fundamental principles of ellipsometry is critical to grasp how this optical technique operates and its applications in various fields. Ellipsometry is uniquely suited for characterizing thin films, surfaces, and interfaces, providing both qualitative and quantitative data under in situ conditions. The significance of this section cannot be understated; it lays the groundwork for more complex discussions on instrumentation, data analysis, and applications that follow.
Basic Concepts
Ellipsometry hinges on the polarization of light. The method involves measuring the change in polarization as light reflects off a material's surface. This change yields key information about the material's optical properties. By noting the amplitude ratio and phase difference between the reflected light waves, scientists can infer critical parameters such as thickness and refractive index. The core variables are denoted by the terms psi (Ψ) and delta (Δ), which are fundamental to the interpretation of ellipsometric data.
- Psi (Ψ): Represents the amplitude ratio of the light waves.
- Delta (Δ): Represents the phase difference between the reflected light waves.
The relationship between these variables effectively captures changes in the optical properties of the thin films or surfaces under investigation. Understanding how these principles interrelate is essential for effective measurement and data interpretation.
Measurement Techniques
Measurement techniques in ellipsometry vary, each suited for specific applications and materials. Two primary configurations exist: variable angle and fixed angle ellipsometry.
- Variable angle ellipsometry allows the adjustment of the incident light angle. This technique enhances sensitivity to film thickness and composition by changing the angle of incidence.
- Fixed angle ellipsometry maintains a constant angle and is simpler to operate. It is generally used when dealing with homogenous materials or layers.
Equipment also plays a significant role in measurement accuracy. The choice of light source affects sensitivity and wavelength precision. Common light sources include lasers and light-emitting diodes (LEDs). Furthermore, advanced detection systems, such as photomultiplier tubes or CCD cameras, can increase the technique's performance, allowing for rapid data acquisition even in complex environments.
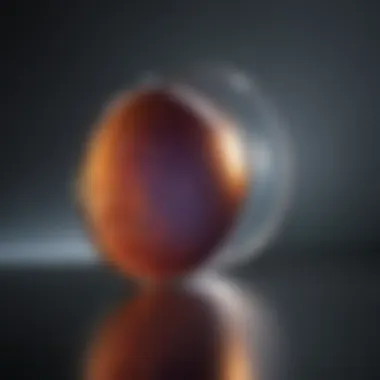
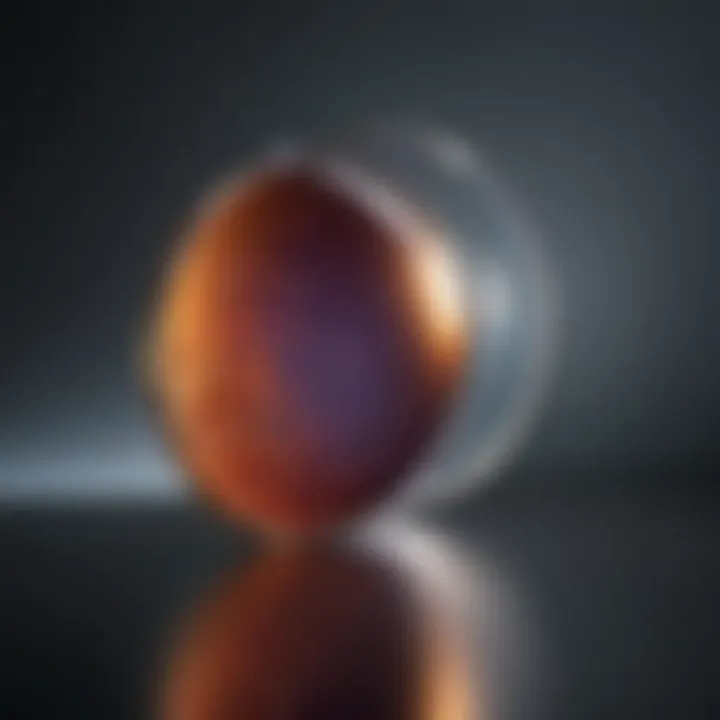
Optical Properties of Materials
The optical properties of materials are paramount in ellipsometry. These properties include refractive index, extinction coefficient, and absorption characteristics. When light interacts with a film, its behavior characteristically changes based on these attributes. Material-specific equations help define these interactions, making it possible to extract quantitative information from ellipsometric measurements.
- Refractive index is an essential parameter that indicates how light propagates through a material compared to its speed in a vacuum.
- Extinction coefficient relates to how much light is absorbed by the material.
Understanding these properties is essential to advancing the application of ellipsometry across various fields, including nanotechnology, materials science, and biological systems analysis.
The core of ellipsometry’s effectiveness lies in its ability to provide precise and sensitive measurements of the optical properties of materials, making it invaluable for monitoring real-time changes in thickness and composition.
Instrumentation for In Situ Ellipsometry
Instrumentation plays a crucial role in the effectiveness of in situ ellipsometry. The specific elements and configurations of these instruments determine their performance and reliability in measuring optical properties of materials in real-time. Understanding the components involved, how to set them up, and the calibration processes is essential for anyone working with this technique. The effectiveness of in situ ellipsometry is directly tied to the quality of the instruments utilized. Therefore, careful consideration of the instrumentation helps to maximize accuracy and minimize uncertainties during experimentation.
Basic Components
The basic components of an in situ ellipsometry setup include the light source, optical elements, detector, and the sample holder.
- Light Source: Typically, these systems use a monochromatic light source, such as a laser. The choice of wavelength is critical to match the material characteristics being studied.
- Optical Elements: Polarizers, beamsplitters, and wave plates are configured to manipulate light before it interacts with the sample. Their arrangement can define the polarization state and intensity significantly, impacting measurement outcomes.
- Detector: A photodetector or CCD camera is employed to gather the reflected light, capturing intensity and phase information. The efficiency of the detector can influence data quality and speed.
- Sample Holder: This component stabilizes and maintains the sample's environment, allowing for consistent measurements subjection to the same conditions during the experiments.
Each of these components needs to be combined thoughtfully to achieve the desired measurement precision, making them indispensable to the ellipsometry process.
Setup Configuration
The arrangement of components defines the setup configuration of the ellipsometry system. A standard configuration includes a beam path that directs the light towards the sample at a specific angle of incidence.
- Angle of Incidence: This angle impacts the sensitivity of the measurements and should be selected based on the material properties.
- Alignment of Components: Proper alignment ensures maximum interaction between the light and the sample. Misalignment can lead to erroneous results, thus rigorous alignment protocols are necessary.
- Sample Environment: The setup should also consider the ambient conditions, such as temperature and pressure. Environmental control mechanisms may need to be integrated to manage these factors effectively.
A well-configured setup facilitates precise measurements, enhancing the overall utility of in situ ellipsometry as a technique for real-time surface analysis.
Calibration Procedures
Calibration is vital to guarantee that in situ ellipsometry measurements are reliable and valid. It involves adjusting the instrument's parameters to correct any systematic errors.
- Standard References: Commonly, known reference materials are used for calibration. Measurements of these known samples provide a baseline to compare against future measurements.
- Adjustment of Parameters: This process may include tweaking the light source intensity or optimizing the detector sensitivity.
- Software Tools: Calibration often involves software tools that apply algorithms to correct for any identified discrepancies, thus ensuring accuracy.
Effective calibration fosters trust in the data gathered, allowing researchers to draw meaningful conclusions from the results.
In situ ellipsometry relies deeply on instrumentation accuracy, which directly impacts the reliability of derived findings.
Careful attention to these aspects of instrument setup and calibration ensures that in situ ellipsometry remains a robust tool for advancing our understanding of material properties.
Experimental Methods
The section on Experimental Methods is pivotal in understanding how in situ ellipsometry operates within practical environments. The meticulous approach to experimental methods helps ensure the accuracy and reliability of the measurements that are critical to exploiting the full potential of this technique. This chapter resonates with researchers, as it outlines the necessary protocols that precede, accompany, and follow ellipsometric measurements. The core topics covered highlight specific methodologies that facilitate effective data gathering in real-world settings and the various factors influencing experimental outcomes.
Sample Preparation Techniques
Sample preparation is crucial for obtaining accurate and reproducible results in in situ ellipsometry. The quality of the samples directly influences the measurement data. This process includes the cleaning, coating, and arranging of materials before the actual measurement takes place. Proper cleaning protocols are pivotal to remove contaminants that might affect the optical properties of the surface. Using solvents such as ethanol or acetone often proves effective in achieving clean, smooth surfaces. It is important also to ensure that the samples are uniformly prepared to minimize variability.
One common technique involves spin coating, which creates thin and uniform layers of materials on substrates. This method can create layers of precise thickness, essential for evaluating optical properties accurately. Careful attention should also be given to environmental factors such as humidity and temperature, which can alter sample characteristics and should be controlled during preparation. In summary, stringent sample preparation techniques directly contribute to the successful application of in situ ellipsometry by maximizing the reliability of the measurements.
Real-Time Measurements
The capability to perform real-time measurements is a defining feature of in situ ellipsometry. Such an approach allows for immediate observation of changes in the material’s optical properties under various stimuli, such as temperature, pressure, or chemical environment. This immediacy offers several benefits; it enables researchers to monitor processes as they happen, providing insights into dynamic systems like thin film growth or chemical reactions occurring at surfaces.
Real-time measurement systems must incorporate sophisticated data acquisition tools that can handle fluctuations and provide immediate analyses of reflected light polarization states. This includes using fast detectors and appropriate algorithms capable of processing large data sets efficiently. Consequently, practitioners can capture time-resolved data, allowing for a deeper understanding of processes over time. Adopting real-time measurement strategies serves to enhance the applicability of in situ ellipsometry in various research domains, such as materials science and nanotechnology.
Data Acquisition Strategies
Data acquisition is a fundamental aspect of in situ ellipsometry, as it determines how effectively the measurement information is collected and interpreted. Several strategies may enhance data quality and ensure comprehensive analysis.
Selecting appropriate measuring intervals is vital. This selection should align with the expected dynamics of the phenomena being studied. Fast acquisition rates are generally preferred to capture short-lived events, while longer intervals may suffice for slower processes. Additionally, employing multiple wavelengths can glean more information from a single sample, enabling a broader profile of optical characteristics. A systematic approach to gathering data, including the setting of baseline measurements and careful consideration of noise, will also significantly enhance the quality of outcomes.
Using dedicated software tools can streamline data handling and analysis. Many modern systems can exploit machine learning techniques to identify patterns and assist in interpreting complex datasets. The seamless integration of software and hardware in the data acquisition chain facilitates thorough analysis, promotes reproducibility, and ultimately improves the reliability of findings. Overall, a well-planned data acquisition strategy lays the groundwork for the meaningful application of in situ ellipsometry across various scientific fields.
Data Analysis in In Situ Ellipsometry
Data analysis plays a crucial role in in situ ellipsometry. As an optical technique, ellipsometry provides a wealth of information about film thickness, refractive index, and other optical properties in real time. However, to transform these raw measurements into meaningful results, effective data analysis is necessary. This section will explore the key components of data analysis in in situ ellipsometry, focusing on mathematical models, software tools, and the interpretation of results.
Mathematical Models
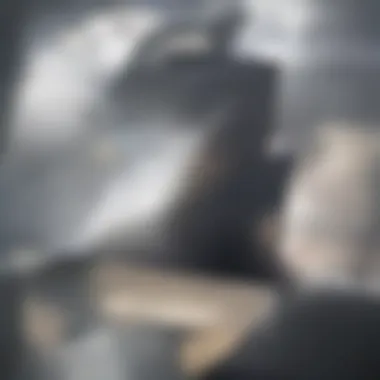
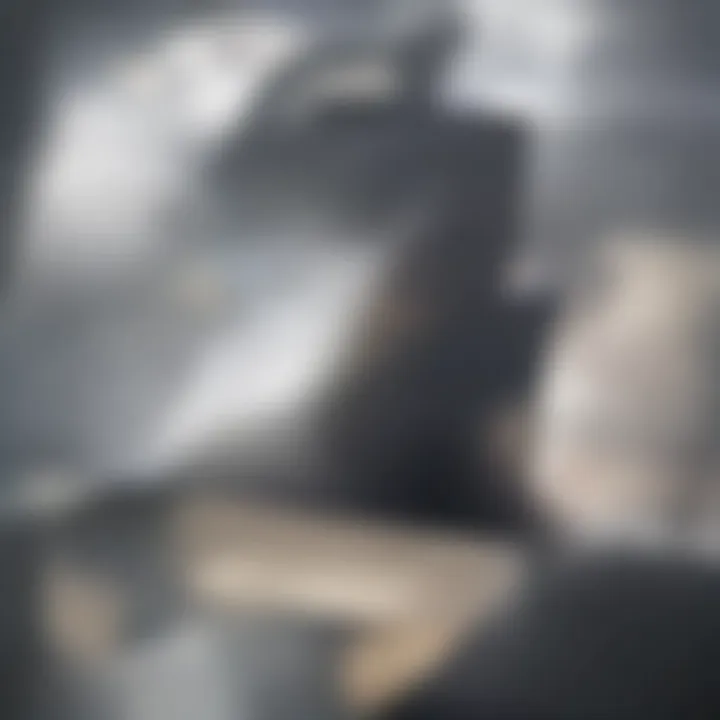
Mathematical modeling forms the foundation of data analysis in ellipsometry. These models describe how light interacts with materials, serving to translate the optical measurements into physical properties. A common approach is to use the Fresnel equations, which relate the amplitude and phase of light waves reflected from a material surface.
Various modeling strategies exist depending on the complexity of the sample. For instance, the basic model applies to single-layer films, while more advanced models consider multilayer structures or anisotropic materials. The choice of model impacts the accuracy of the results.
Some frequently used mathematical models include:
- Cauchy model: It describes the refractive index as a function of wavelength, which is useful for transparent films.
- Lorentz-Drude model: Suitable for metallic films, it accounts for both dielectric and conductive behaviors of materials.
- Tauc-Lorentz model: Applied for amorphous semiconductors, it connects absorption and refractive index.
Accurate modeling requires knowledge of the film's properties and thorough fitting procedures. A common practice is to utilize regression analysis to minimize differences between observed and theoretical values.
Software Tools
The reliance on sophisticated software tools is significant in the data analysis of ellipsometry. These tools streamline both the modeling process and the interpretation of results. Notable software packages include:
- WVASE: This tool is widely used for modeling thin films and allows diverse data input.
- Ellipsometry Analysis Tool (EAT): It focuses on real-time data processing and can handle complex models.
- Mathcad: While not specific to ellipsometry, it provides an environment for mathematical computations and can integrate models.
Additionally, many modern ellipsometers come with integrated software that facilitates real-time analysis. This integration enables researchers to visualize data as it is collected, enhancing the efficiency of experiments.
Interpretation of Results
Interpreting results from in situ ellipsometry requires critical thinking and a clear understanding of both the underlying physics and the limitations of the measurement technique. Researchers must analyze how the optical constants derived from data correlate with the physical properties of the materials studied.
Key considerations in result interpretation include:
- Data Quality: Understanding the noise level and potential artifacts in the data is critical. Noise can arise from various sources like environmental vibrations or fluctuations in light source intensity.
- Model Fit vs. Reality: A good fit in the model does not always mean the model accurately represents the sample. Researchers should validate the results using complementary characterization techniques, such as X-ray reflectivity or atomic force microscopy.
- Contextual Information: The broader context of the study is essential. Results derived from in situ ellipsometry might differ based on sample history or environmental factors, thus necessitating a comprehensive examination of the experimental setup.
In summary, the analysis of data in in situ ellipsometry is an intricate process involving mathematical modeling, software applications, and thoughtful interpretation. Each of these elements contributes to deriving useful and valid conclusions, facilitating advancements in research across multiple domains.
"Understanding data analysis in in situ ellipsometry is key to unlocking its full potential across scientific disciplines."
By mastering these techniques, researchers can enhance their ability to characterize materials efficiently, leading to innovative applications and deeper insights into material properties.
Applications of In Situ Ellipsometry
In situ ellipsometry presents a significant advancement in optical measurement techniques, particularly applicable in various fields of science and engineering. The real-time measurement capabilities facilitate dynamic analysis of thin films and surfaces, allowing for immediate feedback on experimental conditions and parameters. This enhances precision in research, leading to more reliable data and outcomes.
Thin Film Characterization
Thin films are integral in a multitude of applications, particularly in electronics and coatings. In situ ellipsometry enables continuous monitoring of the film growth and properties, thus providing insights into the thickness, refractive index, and optical constants of the films as they develop.
- Real-time Feedback: Unlike other measurement techniques, in situ ellipsometry can provide immediate data on how the optical characteristics change during the deposition process. This is crucial for optimizing conditions in thin film fabrication.
- Non-Destructive Measurement: The method involves minimal alteration to the sample, a key advantage when analyzing sensitive materials.
- Versatility Across Materials: The approach is suitable for various materials including metals, semiconductors, and polymers, making it a broadly applicable technique.
Some typical applications include monitoring the growth of semiconductor layers in photovoltaic cells or analyzing dielectric films in optics. Both scenarios benefit from precise control over the material properties during the fabrication process.
Biological Systems Analysis
In situ ellipsometry is also finding its place in the domain of biological sciences. It allows researchers to investigate living cells and biofilms without extensive preparation or destruction of samples. This capability supports advanced studies in fields such as biosensing and biomaterials research.
- Surface Interaction Observations: The technique can discern interactions at the molecular level, important for understanding how drugs interact with cell membranes.
- Monitoring Biological Processes: Real-time measurements enable the study of growth dynamics and morphological changes in live cells or tissue samples.
- Applications in Drug Delivery: Tracking how drug molecules penetrate biological films can provide valuable data for pharmaceutical development.
This type of analysis opens new avenues for research, especially in developing therapeutic strategies and understanding cellular behavior in various environments.
Nanotechnology
The growing field of nanotechnology benefits greatly from in situ ellipsometry. The ability to analyze materials at extremely small scales allows scientists to tailor properties with high precision. In nanotechnology applications, the technique helps reveal key parameters critical for device performance.
- Characterization of Nanostructures: In situ ellipsometry is pivotal in assessing the optical properties of nanoparticles, quantum dots, and nanocomposites.
- Development of Nanoscale Coatings: This technique is effective in monitoring the formation and characterization of nanoscale coatings, thus enhancing their functionality and stability for diverse uses.
- Integrating with Other Techniques: The versatility of combining in situ ellipsometry with other methods, such as atomic force microscopy, enhances the understanding of nanoscale phenomena.
In summary, the applications of in situ ellipsometry extend across diverse disciplines including materials science, biology, and nanotechnology. This breadth illustrates its capacity to improve understanding and foster innovation in research.
Recent Advancements in Technology
Recent technological advancements in in situ ellipsometry are pivotal as they enhance the precision and applicability of this optical method. This section delves into the significance of these developments, particularly exploring automation, integration with other techniques, and emerging trends. These advancements not only improve measurement accuracy but also expand the range of applications, making in situ ellipsometry a crucial tool in various research fields.
Automation and High-Throughput Techniques
Automation in in situ ellipsometry refers to the implementation of automated systems for conducting measurements and data collection. This innovation allows researchers to increase throughput and reduce human error during experimentation. High-throughput techniques enable simultaneous analysis of multiple samples, thus accelerating research timelines. Here are some benefits:
- Increased Efficiency: Automated systems can perform repetitive tasks without fatigue, leading to more consistent results.
- Data Integrity: Automation minimizes the risk of human error, ensuring reliability in data collection.
- Cost-Effectiveness: By improving throughput, fewer resources are needed to obtain substantial data over shorter periods.
Automated ellipsometers are often integrated with sophisticated software for real-time data analysis. These improvements allow for quicker adjustment of parameters during ongoing experiments, thus facilitating greater control over the experimental conditions.
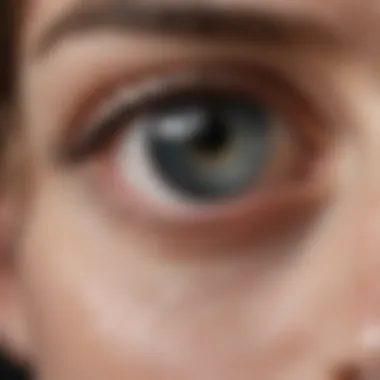
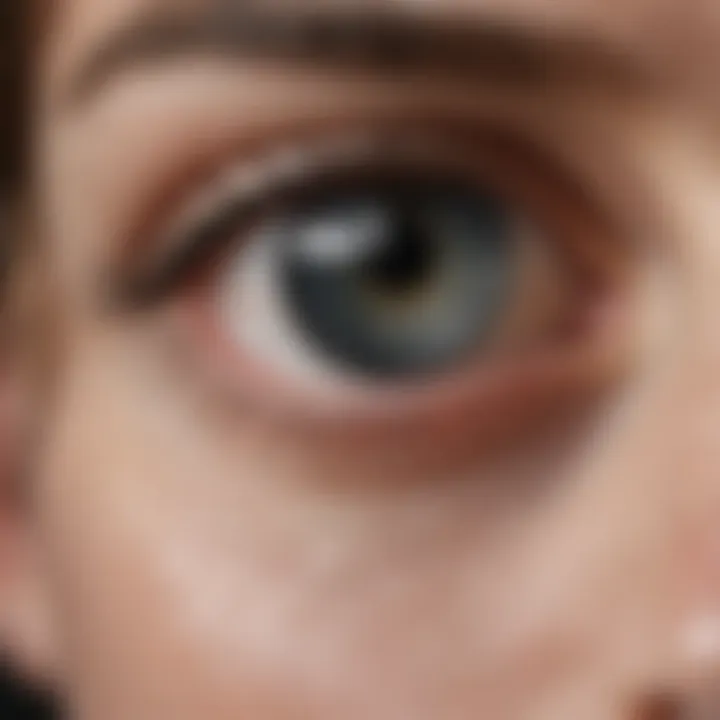
Integration with Other Techniques
Integrating in situ ellipsometry with complementary techniques offers a more comprehensive approach to material characterization. This combination enhances the quality of data obtained and broadens the scope of analysis. For instance:
- Coupling with Spectroscopy: When combined with techniques like infrared or Raman spectroscopy, in situ ellipsometry can provide detailed insights into molecular interactions and chemical bonding.
- Hybrid Systems: Systems that incorporate ellipsometry with atomic force microscopy or electron microscopy allow for simultaneous topographical and optical measurements.
- Data Fusion: Merging data from various techniques provides multifaceted information, improving the understanding of the material’s physical and chemical properties.
Such integrated setups are essential when studying complex materials or systems, such as thin films in nanotechnology or biological samples, where multifarious factors must be considered.
Emerging Trends
The landscape of in situ ellipsometry is continually evolving, with several emerging trends shaping its future. Keeping abreast of these trends is crucial for researchers looking to harness the full potential of this technique. Some notable trends include:
- Machine Learning Applications: The incorporation of machine learning algorithms into data analysis is enhancing predictive capabilities, thus allowing for more informed decisions regarding material properties.
- Miniaturization: The trend towards smaller, more portable ellipsometers is enabling studies in more diverse environments, such as field research or clinical settings.
- Real-Time Monitoring: Increased demand for real-time monitoring applications continues to drive developments in software and hardware that facilitate immediate data analysis.
"The advancements in technology related to in situ ellipsometry represent a convergence of optics, materials science, and data analytics, underscoring its relevance in modern research."
In summary, the recent advancements in technology play a crucial role in enhancing the capabilities of in situ ellipsometry. Through automation, integration, and emerging technological trends, this field continues to grow and adapt to the needs of diverse scientific research.
Challenges in In Situ Ellipsometry
In situ ellipsometry, while a valuable tool in material characterization, presents several challenges that researchers and practitioners must navigate. Understanding these challenges is critical for optimizing experimental outcomes and ensuring accurate data interpretation. This section delves into technical limitations and operational difficulties that affect the application of in situ ellipsometry.
Technical Limitations
One primary technical limitation in in situ ellipsometry involves the sensitivity of measurements to environmental factors. Variations in temperature, humidity, and ambient light can lead to significant changes in ellipsometric signals. Such variations can introduce unwanted noise and errors into the data, thus complicating the reliability of measurements. To mitigate these influences, rigorous control of experimental conditions is essential.
Moreover, the optical configuration and calibration of the ellipsometer can impact the precision of measurements. Misalignment of optical components or inadequate calibration can produce skewed results. Regular calibration procedures are necessary to maintain system accuracy. Additionally, the choice of compatible materials for samples can further influence data quality. In cases where the optical properties of materials are not well-defined or understood, characterizing the sample becomes much more challenging.
"In situ ellipsometry is most effective when all technical parameters are optimized and environmental factors thoroughly controlled."
Operational Difficulties
Operational difficulties can arise during the day-to-day execution of in situ ellipsometry experiments. One major concern involves the complexity of real-time data analysis. Collecting ellipsometric data requires sophisticated software capable of processing and interpreting the information swiftly. For practitioners lacking experience with such software, the learning curve can be steep. This can lead to misinterpretations or delays in obtaining meaningful results.
Another operational challenge lies in sample preparation. Preparing samples in a manner conducive to accurate ellipsometric measurement can require significant effort and expertise. For instance, achieving uniformity across thin films is vital; variations can lead to inconsistencies in data. Moreover, the need for real-time monitoring often compels researchers to work quickly, potentially compromising sample integrity if not handled carefully.
Finally, integrating in situ ellipsometry into existing laboratory workflows may present logistical issues. It requires specialized equipment and trained personnel. The adequacy of the physical space and resources can also hinder its implementation in some research environments. Addressing these operational difficulties demands comprehensive training and a commitment to adopting best practices in ellipsometric techniques.
Future Directions
The exploration of future directions in in situ ellipsometry is essential not only for advancing the technique itself but also for broadening its applicability across various domains of scientific research. Understanding where this field is headed allows researchers and professionals to anticipate developments that may enhance their work and open new avenues for inquiry. The following sections delve into potential innovations and the expansion of applications related to in situ ellipsometry.
Research Innovations
In situ ellipsometry is poised for several innovative advancements. A significant area of interest includes the integration of artificial intelligence and machine learning into data analysis processes. These technologies can streamline the interpretation of complex ellipsometric data, enabling quicker and more accurate conclusions. By harnessing AI algorithms, researchers can identify patterns that are not easily discernible through traditional methods, thereby enhancing the efficiency of their investigations.
Moreover, improvements in sensor technology are on the forefront. More sensitive and miniaturized sensors can provide higher resolution measurements in real time. This is particularly beneficial in fields where precision is critical, such as semiconductor manufacturing and nanotechnology. Additionally, the trend toward automating experimental setups suggests a future where in situ ellipsometry can be conducted with less human intervention, leading to increased reproducibility in experiments.
Finally, there is a growing interest in the development of multi-wavelength ellipsometry. By measuring multiple wavelengths simultaneously, researchers can obtain more comprehensive data sets that provide deeper insights into material properties and behaviors, substantially improving the characterization of complex systems.
Expanding Applications
The potential applications of in situ ellipsometry will likely expand significantly in the coming years. One area ripe for exploration is the field of renewable energy. In situ ellipsometry can be utilized to monitor the deposition and quality of thin films used in solar cells, ensuring optimal performance and longevity of photovoltaic devices.
Another promising domain is in the biotechnology sector. The capacity of in situ ellipsometry to analyze biological systems in real time opens new avenues for studying cellular processes, drug interactions, and biomolecular behaviors. Such insights can greatly enhance the development of targeted therapies and personalized medicine.
Furthermore, in situ ellipsometry is expected to find new applications in the nanotechnology sphere. As researchers work with nanomaterials, precise measurement techniques are crucial. The ability to monitor thin film growth at the nanometer scale offers substantial benefits to the development of nanostructured materials and devices, including sensors, catalysts, and drug delivery systems.
Epilogue
The conclusion of this article serves as a crucial synthesis of the principal ideas discussed throughout. It highlights not just the significance of in situ ellipsometry but also emphasizes the intricate relationship between its principles, applications, and technological advancements.
Summary of Findings
In this article, we have explored the essential elements of in situ ellipsometry, detailing its fundamental principles and the technical instrumentation necessary for its application. Key findings include:
- Real-time Measurements: In situ ellipsometry allows for immediate monitoring of thin film behaviors, providing insights into material properties that are critical in several fields such as nanotechnology and biological systems.
- Diverse Applications: The technique has been employed across various disciplines, emphasizing its versatility. From semiconductor research to biomaterials, the range of applications shows its broad relevance.
- Data Analysis Techniques: The discussion covered mathematical models and software tools that aid in the interpretation of data, underscoring the complexity and sophistication of analyses that can be conducted using this technique.
This synthesis not only reinforces the importance of understanding in situ ellipsometry in the context of optical measurement but also paves the way for future research opportunities.
Implications for Future Research
In situ ellipsometry holds substantial promise for future scientific inquiry. Several implications arise from the findings discussed:
- Enhancing Measurement Precision: Continuous improvements in measurement techniques could lead to more accurate characterizations of materials, which is vital for the development of new technological applications.
- Integrating Multimodal Approaches: The potential integration of in situ ellipsometry with other analytical techniques could enhance data richness and provide more comprehensive insights into complex systems.
- Exploring Novel Applications: As the technology evolves, new fields of study may emerge. This could expand applications into environmental science or advanced manufacturing, where material behavior under various conditions requires close monitoring.
Understanding these implications will not only drive innovations but also enable researchers to push the boundaries of what is possible with in situ ellipsometry. This underscores the importance of continued exploration in this area, ensuring its vital role in future scientific discoveries.