Understanding the qPCR Process: Step-by-Step Guide
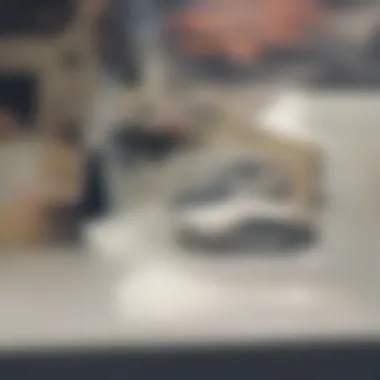
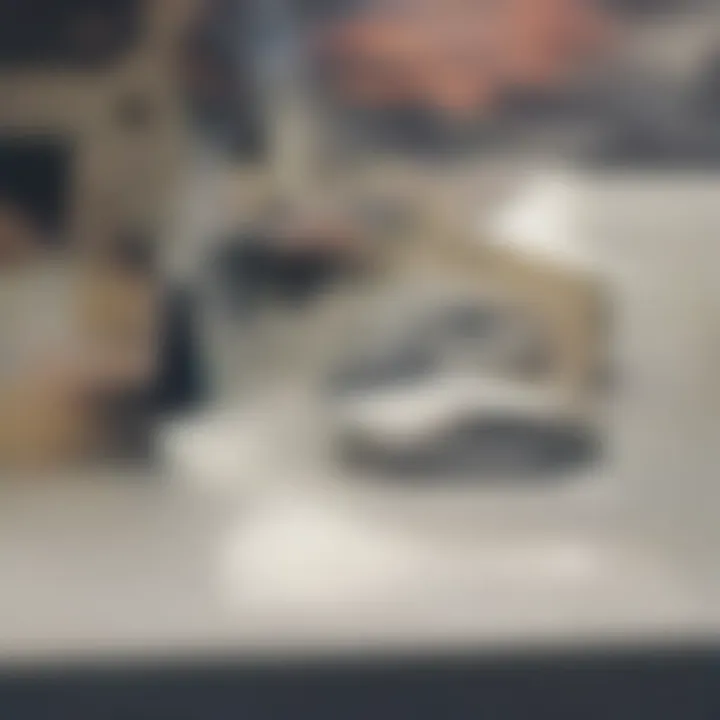
Intro
Quantitative Polymerase Chain Reaction, commonly known as qPCR, plays a crucial role in molecular biology. It allows scientists to quantify nucleic acids with high precision. The increasing reliance on this technique underscores its importance in research and diagnostic applications. This guide will delve into each step involved in qPCR, offering clarity on the entire process. Understanding these steps not only enhances the reliability of results but also optimizes research methodologies.
Research Background
Overview of the scientific problem addressed
The ability to measure gene expression and detect low-abundance nucleic acid sequences is fundamental in many areas of scientific research. Traditional PCR methods do not provide quantitative data, making it challenging to gauge the amount of DNA or RNA present in a sample. qPCR was developed as a solution to this limitation. The method enables researchers to quantify nucleic acids in real-time, making it vital in both basic research and clinical settings.
Historical context and previous studies
The origins of qPCR date back to the 1990s when advancements in fluorescence technology allowed for more accurate monitoring of DNA amplification during the PCR process. Prior studies established the foundation for various qPCR methods. One significant study published in 1996 highlighted the use of hybridization probes in real-time PCR, paving the way for further advancements. Over time, different approaches were introduced, including SYBR Green and TaqMan assays, both of which are popular today.
These developments have made qPCR a standard technique in laboratories worldwide, from academic institutions to clinical diagnostics. The historical progression illuminates how far the technology has come, enhancing research capabilities in numerous fields, such as genetics, oncology, and infectious disease.
Findings and Discussion
Key results of the research
Recent research utilizing qPCR has demonstrated its effectiveness in various applications. Studies show that qPCR is not only reliable for quantifying gene expression but also instrumental in detecting pathogens. The accuracy and sensitivity of this technique allow for the detection of virus RNA in low concentrations, crucial for early diagnosis of diseases like COVID-19.
Interpretation of the findings
Understanding the results from qPCR experiments involves careful analysis. The data obtained can indicate the level of nucleic acid present, which helps researchers draw conclusions about biological processes. Further, consistent methodologies ensure reproducibility, which is critical for scientific validation. Thus, the precise execution of qPCR protocols directly affects the quality of research and any subsequent findings.
"Precision in qPCR is not just desirable; it is essential for reliable results that further scientific advancement."
As we continue to explore this technique, a refined understanding of each step will empower researchers and expand the applications of qPCR methodologies.
Prolusion to QPCR
Quantitative Polymerase Chain Reaction (qPCR) stands as a cornerstone technique in modern molecular biology. Its ability to amplify and quantify specific nucleic acid sequences makes it indispensable for various applications in research and diagnostics. The introduction of qPCR has revolutionized the way scientists study gene expression, pathogen detection, and genetic variation. It offers a level of precision and efficiency that traditional methods simply cannot match.
Definition of Quantitative PCR
Quantitative PCR is a technique that allows for the rapid amplification of DNA and RNA sequences while simultaneously enabling quantification. This dual capability differentiates qPCR from traditional PCR. In qPCR, the amplification process is monitored in real-time, providing measurable data throughout the cycling process. This quantitative aspect allows for the determination of initial template quantities based on the results obtained after amplification.
Importance in Molecular Biology
The significance of qPCR in molecular biology cannot be overstated. It serves multiple critical functions:
- Gene Expression Analysis: Researchers use qPCR to assess mRNA levels, shedding light on gene activity and regulation.
- Pathogen Detection: In clinical settings, qPCR helps in the early detection of viruses and bacteria, leading to timely interventions.
- Genetic Variation Studies: qPCR aids in analyzing polymorphisms, greatly contributing to fields like personalized medicine and evolutionary biology.
"The capacity of qPCR to provide realtime data makes it an essential tool for any molecular biologist."
The method enhances experimental reproducibility and offers superior sensitivity compared to other quantification methods. As researchers strive for accuracy, qPCR continues to be the method of choice to illuminate complex biological questions.
Basic Principles of QPCR
Quantitative PCR (qPCR) serves a foundational role in molecular biology. It allows researchers to quantify DNA and RNA, offering insights into gene expression, pathogen detection, and various other applications. Understanding the basic principles underpinning qPCR is essential. This knowledge not only aids in selecting appropriate methodologies but also informs troubleshooting and optimization throughout the qPCR process.
Emphasizing principles such as the mechanism of thermal cycling and the role of fluorescent probes will provide a clearer perspective on how qPCR operates. Grasping these essential elements enhances the reliability of the results generated and supports the broader objectives in research.
Mechanism of Thermal Cycling
Thermal cycling is a core concept in qPCR. This process involves repeated changes in temperature to facilitate the amplification of targeted nucleic acids. The phases include denaturation, annealing, and extension.
- Denaturation: The first step involves heating the reaction mixture to approximately 94-98Β°C. This high temperature disrupts hydrogen bonds between nucleotides, causing the DNA strands to separate.
- Annealing: After denaturation, the temperature is lowered to about 50-65Β°C. This allows primers to bind to complementary sequences on the single-stranded DNA.
- Extension: The temperature is then raised to about 72Β°C, providing an optimal environment for DNA polymerase to synthesize new strands by adding nucleotides to the primed sequences.
This cycling is critical. Each cycle potentially doubles the amount of DNA, creating exponential amplification after several repetitions. Understanding this mechanism aids in optimizing conditions for specific templates, ensuring accurate quantification.
Role of Fluorescent Probes
Fluorescent probes are integral to the qPCR process. They facilitate real-time monitoring of the amplification process, thus allowing quantification. Probes can be broadly categorized into two types: non-specific dyes and sequence-specific probes.
- Non-specific Dyes: Dyes like SYBR Green intercalate into any double-stranded DNA. While these are straightforward to use, they cannot distinguish between specific and non-specific amplification.
- Sequence-Specific Probes: Tools like TaqMan or molecular beacons provide greater specificity. These probes incorporate sequences complementary to the target DNA, providing a fluorescent signal only when bound to the intended target. This specificity reduces background noise and enhances the accuracy of quantification.
The use of these probes allows for precise measurement of nucleic acids in real-time. This capability is vital for assessing gene expression or determining viral load in clinical samples. By understanding how these probes work, researchers can make informed choices regarding their qPCR design.
In summary, the basic principles of qPCR encompass the mechanics of thermal cycling along with the utilization of fluorescent probes. Mastering these principles enhances both the efficiency and effectiveness of molecular quantification.
QPCR Workflow Overview
The QPCR workflow overview serves as a pivotal framework in the understanding and execution of the quantitative polymerase chain reaction. Each step in the workflow is crucial as it directly impacts the integrity and reliability of the outcomes. By delineating the complete process from sample preparation to data analysis, researchers can ensure reproducibility and accuracy in their results. This section aims to highlight essential elements, benefits, and considerations that come into play within the QPCR workflow.
One significant aspect of this workflow is its systematic approach. This method not only simplifies complex procedures but also enhances efficiency. Moreover, strict adherence to the workflow enables researchers to troubleshoot potential issues quickly, thereby saving valuable time in experimental setups. It becomes critical to follow this structured methodology, as deviations can lead to unreliable data or misinterpretations.
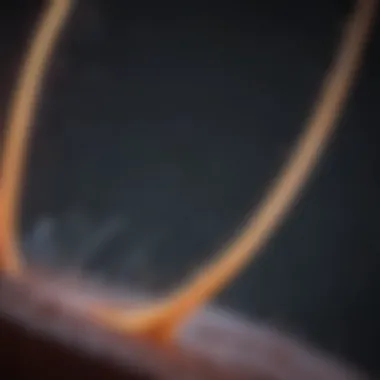
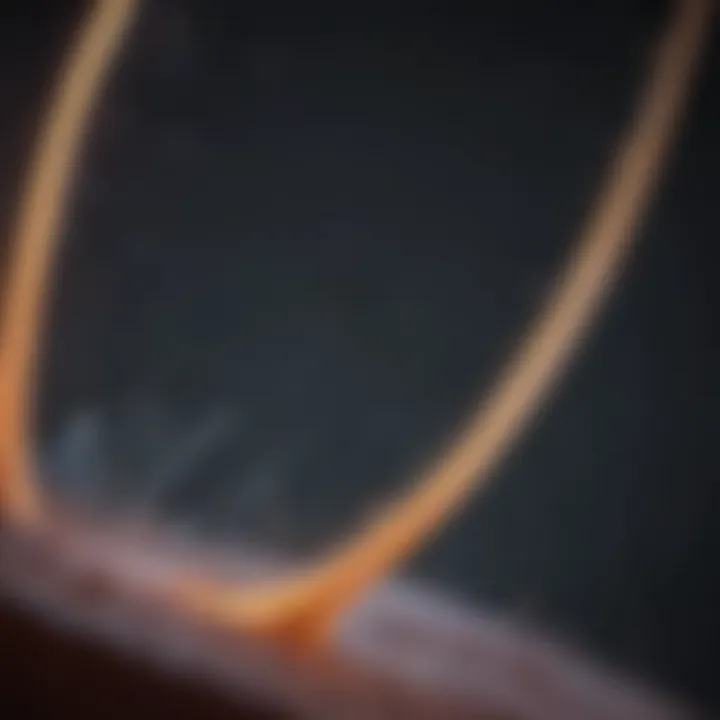
Step-by-Step Process
A detailed step-by-step process outlines each critical phase involved in the QPCR workflow. The following points summarize the essential stages:
- Sample Preparation: This involves isolating nucleic acids, ensuring that quality and quantity are adequate for downstream applications.
- Reagent Preparation: Selecting and preparing the right reagents including master mix, primers, and probes to ensure optimal performance.
- Setting Up the Reaction: Concentrating on proper dilution and plate loading techniques as these can affect the fidelity of amplification.
- Running the Thermal Cycler: This stage encompasses programming thermal cycler settings accurately for the amplification process.
- Data Collection and Analysis: Monitoring the reaction and analyzing the collected data to obtain meaningful results through Ct values.
Incorporating these steps provides a clear pathway for researchers to follow, ensuring that each quantification experiment is executed with precision.
Key Components of QPCR
Understanding the key components of QPCR is vital for anyone involved in this technology. Each component plays an integral role in the success of the QPCR reaction. These components include:
- Thermal Cycler: The machine responsible for the cycling steps that allow for denaturation, annealing, and extension during the amplification.
- Master Mix: A premixed solution containing DNA polymerase, nucleotides, buffers, and salts, crucial for facilitating the reaction.
- Primers: Short DNA fragments designed to initiate the replication of the target nucleic acid, their design impacts specificity and efficiency.
- Fluorescent Probes: These are used to monitor the reaction in real-time by emitting fluorescence signals that correlate with the amount of DNA being amplified.
Effective optimization of these components can significantly enhance quantitative accuracy and overall quality of the experiment.
By focusing on the outlined workflow and understanding these components, researchers can enhance the reliability of their qPCR results and expand their applications in diagnostics and research.
Sample Preparation for QPCR
Sample preparation is a critical stage in the quantitative polymerase chain reaction (qPCR) process. The quality and integrity of nucleic acids are determinative factors in obtaining reliable and reproducible results. Inadequate sample preparation can lead to misleading data, which may compromise the validity of the entire experiment. Therefore, rigorous protocols must be established and followed.
Isolating nucleic acids, which will be discussed next, is pivotal in ensuring that the samples are suitable for qPCR. This entails extracting DNA or RNA from biological samples such as cells, tissues, or fluids. Improper extraction can introduce contaminants or degrade the nucleic acids, influencing the performance of the qPCR.
Moreover, evaluating sample quality shines a light on the status of the extracted nucleic acids. This assessment often involves measuring the concentration and purity of the samples. Poor-quality samples can result in inconsistent or erroneous qPCR outcomes. Thus, employing standardized methods for both isolation and quality evaluation is vital for successful qPCR experiments.
"Robust sample preparation sets the foundation for reliable qPCR results."
The benefits of meticulous sample preparation for qPCR include:
- Improved data reliability
- Enhanced reproducibility of results
- Minimization of contamination risks
In essence, thorough and systematic sample preparation cannot be overstated. It serves as the bedrock upon which all subsequent qPCR steps stand, influencing everything from thermal cycling to data analysis.
Reagent Preparation
Reagent preparation is a critical phase in the qPCR process. The success of the experiment heavily relies on the quality and suitability of the reagents being used. Properly prepared reagents can greatly influence the efficiency, sensitivity, and specificity of the qPCR reaction. A comprehensive understanding of this stage can help in minimizing errors and enhancing reproducibility.
Selecting the Right Master Mix
Choosing an appropriate master mix is essential for successful qPCR experiments. Master mixes typically contain all necessary components, including DNA polymerase, buffer, dNTPs, and often, a stabilizer. Here are some considerations to keep in mind:
- Specificity: Select a master mix that is designed for the type of target nucleic acid you are working with. Some mixes are optimized for certain assays, which can improve specificity.
- Sensitivity: High-quality master mixes often provide higher sensitivity, allowing for the detection of low abundance targets. Look for mixes that have been validated for the specific application you intend to use.
- Compatibility: Ensure that the master mix is compatible with the fluorescent probes and primers you selected. Incompatibility can lead to suboptimal results.
By taking the time to select a suitable master mix, researchers can enhance their chances of obtaining reliable and meaningful data.
Adding Primers and Probes
The addition of primers and probes is a pivotal step in setting up the qPCR reaction. Primers are short DNA sequences that bind to the target nucleic acid, while probes provide a means to measure the amplification process through fluorescence. Here are crucial aspects to consider:
- Primer Design: Invest time in designing high-quality primers. They should be specific to the target sequence and have optimal melting temperatures. Aim for minimal secondary structure, as this can hinder amplification.
- Probe Selection: Probes should be chosen based on the qPCR platform being used. Different types of probes like TaqMan or SYBR Green serve different purposes and have particular characteristics. Make sure to understand their differences before choosing one.
- Concentration: The concentration of primers and probes in the reaction mix is critical. Too much or too little can adversely affect the efficiency of the amplification. Typically, primer concentrations range from 100 to 400 nM, but this can vary depending on the specific protocol.
Incorporating the right primers and probes is vital for achieving accurate and reproducible results in qPCR. This ensures that the desired targets are amplified properly and detected accurately.
Setting Up the QPCR Reaction
Setting up a quantitative polymerase chain reaction (qPCR) is a vital component of the overall process. This stage is pivotal because it directly influences the accuracy and reliability of the results obtained from the qPCR experiment. Proper setup ensures that each reaction is optimized to amplify nucleic acids efficiently while minimizing the potential for errors or variability.
Dilution of Samples
The dilution of samples is essential to create a workable concentration range for the qPCR reaction. It is important to adjust the concentration of the nucleic acids to fall within the optimal linear range for quantification. If the input concentration is too high, this might lead to signal saturation in the detection phase. This saturation distorts the data, making it difficult to ascertain accurate results. On the other hand, if the concentration is too low, the sensitivity of the assay may be compromised.
Typically, sample dilutions may need to be prepared in multiples, such as 1:10, 1:100, or even more depending on the starting material. It is crucial to use high-purity reagents and sterile techniques during this step to prevent contamination, which could compromise the integrity of the results. Accurate pipetting is paramount, as any deviation can lead to significant errors in quantification. In addition, the dilution factors must be carefully recorded to facilitate precise calculations in subsequent data analysis.
Plate Loading Techniques
The process of loading plate wells with prepared qPCR mixtures also plays a significant role in the success of the experiment. Adherence to proper loading techniques enhances reproducibility and reliability of the results. When loading samples, it is advisable to follow a consistent pattern. For example, loading samples in a diagonal or sequential manner can help to avoid cross-contamination between wells.
Additionally, consistent volume loading into each well is critical. Volume discrepancies can introduce variability and affect the amplification outcomes. Using multichannel pipettes can increase efficiency while maintaining accuracy, particularly when dealing with larger sample sizes.
Regarding the qPCR plate, it is often beneficial to include negative and positive controls. Negative controls help identify potential contamination sources, while positive controls ensure that the amplification process is functioning correctly.
Overall, the effectiveness of the qPCR reaction significantly depends on how well the samples are diluted and subsequently loaded into the plates. Taking the time to perfect these steps increases the likelihood of obtaining valid, meaningful data in your qPCR analysis.
Running the QPCR
Running the qPCR is a crucial step that often determines the outcome of the entire process. Proper execution can yield reliable data that contributes to a better understanding of nucleic acid quantification. At this stage, the meticulous calibration of the thermal cycler and real-time monitoring of the amplification process are paramount. These components influence the precision of results and highlight the importance of appropriately settings, as a slight error could lead to misleading interpretations.
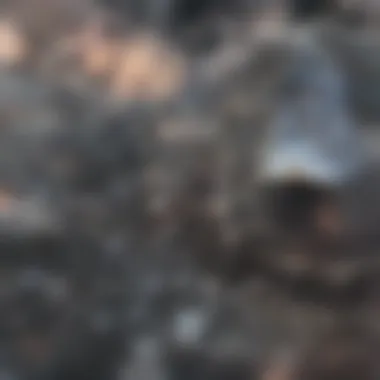
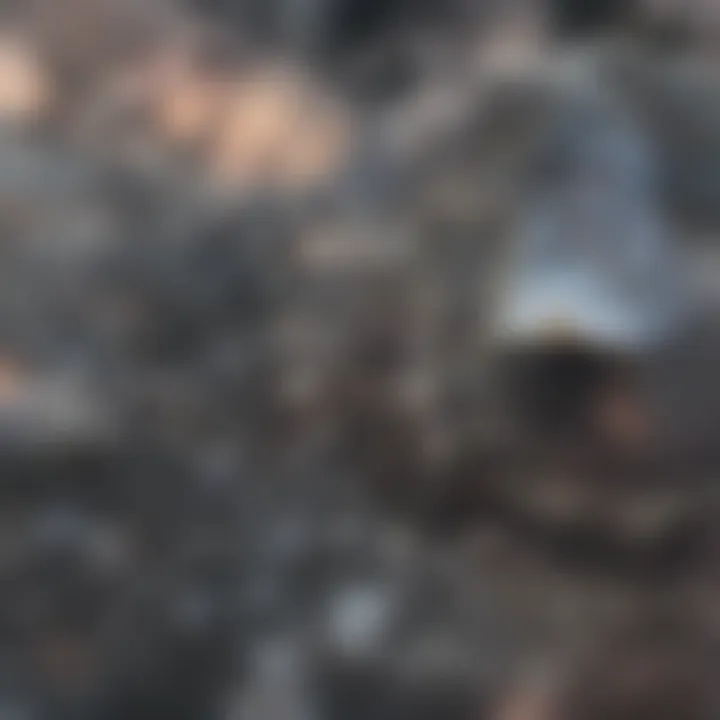
Thermal Cycler Settings
Thermal cycler settings are critical in defining the parameters of the qPCR process. Each cycle involves a series of temperature changes crucial for denaturation, annealing, and extension. Setting these temperatures correctly is vital. Typically, the denaturation step occurs at around 95Β°C, where double-stranded DNA separates. Next, the annealing temperature is set, usually between 50Β°C to 65Β°C, depending on the primers used. Finally, the extension phase happens at approximately 72Β°C where the DNA polymerase synthesizes new DNA strands.
It is also important to determine the number of cycles. Too few cycles may not amplify the target sufficiently, while too many cycles can lead to non-specific amplification, thus generating false-positive results. This fine balance is essential for achieving reproducible and accurate data.
Monitoring Reaction Progress
Monitoring the reaction progress during qPCR is integral to ensuring the validity of the results. Real-time qPCR employs fluorescent dyes or probes to measure the amount of PCR product as it forms. The increase in fluorescence correlates with the quantity of the target nucleic acid, allowing for quantitative assessments.
Researchers often rely on software integrated within the thermal cycler to visualize this data. It's important to assess the threshold cycle (Ct) values, as they denote the point where the fluorescence surpasses the background signal. Proper analysis of these values can provide critical insight into gene expression levels.
By closely observing both the settings and the progress of the qPCR reaction, researchers can minimize potential issues, leading to accurate and informative conclusions in their studies.
"Precision in qPCR is not just about correct settings; it is also about real-time evaluation of the reaction."
Understanding and applying the right settings and monitoring techniques is essential for anyone involved in molecular biology research. The importance of this step cannot be overstated, as it lays the groundwork for accurate data interpretation.
Data Analysis in QPCR
Data analysis in quantitative polymerase chain reaction (qPCR) is a crucial phase that directly affects the interpretation of experimental results. The analysis process involves quantifying the nucleic acids amplified during the PCR, which is vital for various applications in molecular biology. Analyzing qPCR data accurately ensures that researchers draw valid conclusions from their experiments. It helps in identifying gene expression levels, assessing the quality of samples, and even in clinical diagnostics.
Several elements are critical in this analysis, including the interpretation of threshold cycle (Ct) values and the determination of gene expression levels. By understanding these components, researchers can validate their results and enhance the reliability of their findings.
Understanding Threshold Cycle (Ct) Values
Threshold Cycle, commonly referred to as Ct, is a key concept in qPCR data analysis. The Ct value is defined as the cycle number at which the fluorescence signal from the PCR reaction crosses a defined threshold level. It reflects the amount of nucleic acid present in the sample at the beginning of the qPCR process.
- Lower Ct values indicate a higher initial quantity of target nucleic acid, implying that the genetic material was abundant in the sample.
- Higher Ct values suggest that less initial target nucleic acid was present at the start of the reaction.
Accurate determination of Ct values is essential. It allows for the quantification of the target nucleic acids, facilitating comparative analysis across different samples.
Understanding Ct values is fundamental for reliable data interpretation, impacting conclusions drawn about gene expression and sample concentrations.
Determining Gene Expression Levels
Determining gene expression levels using qPCR data involves comparing Ct values of the target genes to those of reference genes, often referred to as housekeeping genes. This comparative approach allows researchers to normalize the data, accounting for any variations in sample quantity and quality.
The steps in determining gene expression levels typically involve:
- Selection of Reference Genes: A proper reference gene is crucial for normalization. It should be stably expressed across all samples to provide consistent results.
- Calculation of ΞCt: This calculation involves subtracting the Ct value of the reference gene from the Ct value of the target gene for each sample. This step provides a comparative measure of gene expression.
- Further Analysis: The relative expression levels can be calculated using methods like the comparative Ct method (2βΞΞCt). This approach facilitates the assessment of gene expression changes across different conditions.
Common Challenges and Solutions
The execution of quantitative polymerase chain reaction (qPCR) involves various challenges that can hinder the accuracy and reliability of results. Recognizing these challenges is crucial for enhancing the quality of experiments in molecular biology. By identifying potential pitfalls and implementing suitable solutions, researchers can improve the robustness of their qPCR analyses. This section will delve into common issues faced during qPCR and suggest effective strategies to address them.
Issues in Sample Contamination
Sample contamination remains a pervasive challenge in qPCR. It can lead to erroneous quantification and misinterpretation of results. Contaminants may originate from multiple sources including laboratory surfaces, reagents, or even the samples themselves. For instance, minute amounts of DNA from previous experiments or reagents can become introduced into the new sample, resulting in false-positive readings.
To alleviate contamination risks, here are several steps to follow:
- Use of Dedicated Equipment: Assign specific pipettes and tubes for qPCR usage, minimizing the chance of cross-sample contamination.
- Good Laboratory Practices: Regularly clean workspaces and tools with appropriate disinfectants. Maintain a organized work area with a clear separation between pre- and post-amplification procedures.
- Incorporate Negative Controls: Always include negative controls in qPCR experiments. This helps identify contamination at any stage of the process.
"The aim should be to create a contamination-free environment, as even a small amount of foreign nucleic acid can jeopardize experimental outcomes."
Taking these proactive measures can significantly reduce the likelihood of contamination and improve overall precision in qPCR results.
Dealing with Inconsistent Results
Inconsistent results are another frequent issue encountered in qPCR experiments. Variability may arise due to improper sample preparation, variations in reagents, or discrepancies in thermal cycling conditions. Such inconsistencies can lead to low reproducibility, making it difficult to compare different experiments or draw valid conclusions.
To tackle inconsistent results, consider the following approaches:
- Standardize Protocols: Use a unified protocol for sample preparation and reaction setup. Having a consistent approach can drastically reduce variability.
- Optimize Reagent Concentrations: Carefully adjust the concentrations of primers and probes to establish optimal conditions for each specific assay.
- Monitor Thermal Cycling: Regularly calibrate thermal cyclers and ensure that they operate under recommended settings. Cycling conditions that are too aggressive can yield suboptimal results.
Applications of QPCR
Quantitative Polymerase Chain Reaction (qPCR) has become an indispensable tool in modern molecular biology. Its applications span various domains, demonstrating its versatility and significance. Understanding how qPCR is applied can help scientists harness its full potential, thus improving research outcomes and diagnostic capabilities. Here, we explore some key applications of qPCR, focusing on its roles in clinical diagnostics and research.
Clinical Diagnostics
In the field of clinical diagnostics, qPCR plays a crucial role by providing rapid and accurate detection of pathogens. This technique allows laboratories to identify infectious agents with high sensitivity. For instance, qPCR can detect viral RNA or DNA in patient samples, enabling swift diagnosis of diseases like COVID-19 or HIV. Such timely identification is essential for initiating appropriate treatment protocols and controlling outbreaks.
Another critical use of qPCR is in the monitoring of viral loads in patients. Regular assessment of viral RNA levels can help healthcare providers understand disease progression or response to antiviral therapies. This monitoring informs treatment decisions, leading to personalized healthcare strategies. Additionally, qPCR assists in genetic testing for hereditary disorders, allowing healthcare professionals to identify genetic mutations that may affect patient outcomes.
The ability of qPCR to quantify nucleic acids makes it particularly useful for analyzing gene expression. Changes in gene expression levels can indicate disease states, making qPCR a valuable tool in oncology. By examining the expression of oncogenes or tumor suppressor genes, clinicians can better understand cancer biology and potentially identify therapeutic targets.
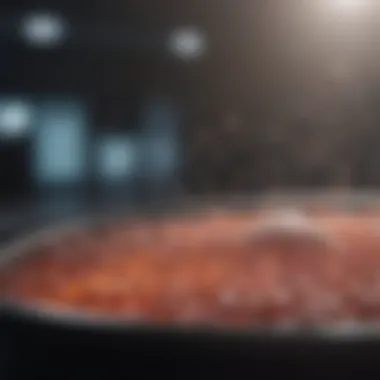
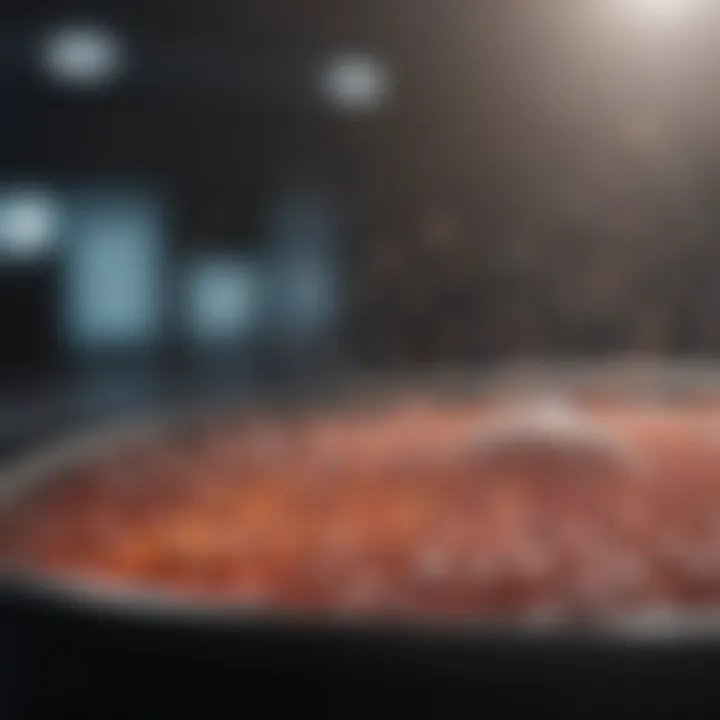
"QPCR enables clinicians to diagnose diseases rapidly and precisely, optimizing patient management and public health response."
Research Applications
In research, qPCR serves as a cornerstone methodology across various fields, including genetics, microbiology, and environmental science. Its ability to quantify nucleic acids allows researchers to investigate gene expression patterns under different conditions. For example, scientists can study how environmental stressors impact the expression of stress-responsive genes in plants or microorganisms.
Moreover, qPCR is instrumental in validating findings from high-throughput sequencing experiments. Researchers can confirm results obtained from RNA sequencing or other omics technologies by quantifying the expression levels of specific genes. This validation is essential to establish the reproducibility of research findings.
Additionally, qPCR facilitates the study of genetic variations. Scientists often use it to investigate single nucleotide polymorphisms (SNPs) and their associations with diseases. By identifying these variations, researchers can develop better strategies for disease prevention and treatment.
Another area where qPCR finds extensive application is in microbiome studies. By quantifying microbial DNA from diverse environments, researchers can assess the diversity and composition of microbial communities. This insight is crucial in understanding how these communities affect health and disease.
In summary, qPCR has far-reaching applications in clinical diagnostics and research. Its precision and reproducibility make it a powerful method for analyzing nucleic acids, leading to advancements in both healthcare and scientific understanding. As technology evolves, we can expect qPCR applications to expand, ultimately enhancing the quality of research and diagnostics across multiple fields.
Future Directions in QPCR Technology
The field of quantitative polymerase chain reaction (qPCR) is in a continual state of evolution. Studying future directions in qPCR technology reveals crucial insights into how this technique will adapt and improve. The growing demand for higher sensitivity, specificity, and throughput drives innovation in qPCR approaches. As professionals in molecular biology navigate changing research needs, understanding these advancements becomes essential. Innovations will not only enhance data accuracy but also result in more comprehensive applications across various scientific fields.
Innovations in qPCR Techniques
Recent developments in qPCR technology include several promising techniques. These range from improvements in instrument design to novel reagent formulations. One notable advancement is the emergence of digital PCR (dPCR). This method further refines quantification by partitioning reactions, providing absolute quantification with minimized bias. Enhanced multiplexing capabilities allow simultaneous amplification of multiple targets in a single reaction. This efficiency reduces both the time and resources needed for experiments.
The incorporation of CRISPR-based techniques has started to influence qPCR. This integration enhances specificity while expanding the range of detectable targets, particularly in diagnostic applications. Such innovations can lead to more precise and faster detection of pathogens in clinical samples, which is vital during outbreaks.
Integration with Other Technologies
As qPCR technology develops, so does its integration with other analytical methods. Combining qPCR with next-generation sequencing (NGS) leverages the strengths of both techniques. This integration allows for comprehensive genomic studies, enabling nuanced analysis of gene expression profiles and mutation detection.
Moreover, qPCR's compatibility with microfluidics technology represents another frontier of advancement. By miniaturizing the qPCR process, researchers can achieve higher throughput and automation. This shift leads to more efficient experiments with reduced reagent costs, thus fostering more extensive applications in research and diagnostics.
Best Practices for QPCR
The effectiveness and reliability of quantitative PCR (qPCR) are heavily influenced by the implementation of best practices. Adopting stringent quality control measures can greatly improve the accuracy of results, which is essential for valid conclusions in research and diagnostics. Given the complexity of qPCR processes, it is crucial to prioritize elements like protocol standardization and equipment maintenance.
Standardizing Protocols
Standardizing protocols is fundamental in ensuring that qPCR experiments produce consistent results. This involves creating a detailed, reproducible outline for each step of the process, from sample preparation to data analysis. Establishing a uniform set of instructions minimizes variability across different experiments.
Key considerations for standardizing protocols include:
- Reagent Consistency: Always use the same brands and batches of reagents for every run. Different sources may yield variations in performance.
- Temperature Conditions: Clearly define and adhere to all temperature conditions for each operational stage. Variations can produce inconsistent results.
- Timing: Ensure that timing for each amplification cycle is strictly followed. Over time, slight deviations can lead to cumulative errors.
- Documentation: Keep thorough records of every experiment. This includes all reagents used, their concentrations, and equipment settings. Such meticulous documentation ensures reproducibility and helps in identifying factors if something goes wrong.
By standardizing protocols, researchers can focus on the intent of their work without worrying about the intricacies of each experimental setup.
Maintaining Equipment
The reliability of qPCR heavily depends on the performance of the thermal cyclers and other equipment used in the process. Regular maintenance ensures that machines function correctly and produce reliable data.
To maintain equipment effectively:
- Routine Calibration: Regularly calibrate thermal cyclers according to manufacturer specifications. This is vital to maintain accuracy in temperature control, which directly affects amplification.
- Cleaning Protocols: Implement appropriate cleaning procedures for equipment. Contamination from leftover samples can lead to erratic results.
- Software Updates: Keep the software used for qPCR analysis updated. Software improvements can enhance performance or offer additional analytical capabilities.
- Assessing Performance: Periodically conduct performance assessments of equipment using control samples. Any deviations from expected performance should be addressed promptly.
"Regular equipment maintenance is not just a good practice; it is essential for ensuring the integrity of qPCR results."
Culmination
The conclusion is a significant part of this article as it synthesizes the key elements of quantitative polymerase chain reaction (qPCR) and reinforces its relevance in molecular biology. In a field where precision and accuracy are paramount, understanding the qPCR process encapsulates not just technical know-how but also positions researchers to make informed decisions in their experiments.
Firstly, the summary of key points serves to remind readers of the critical steps involved in qPCR. This includes sample preparation, reagent selection, thermocycler settings, and data analysis. Each of these stages is interconnected and impacts the reliability of results. A strong grasp of these components allows scientists to mitigate errors and enhance the reproducibility of their findings, which is essential in any scientific inquiry.
Secondly, the implications for future research cannot be overstated. As qPCR technology continues to evolve, new methodologies and applications emerge. The knowledge of current practices offers a solid foundation for innovation within the field. Understanding the intricacies of qPCR facilitates its integration with developing technologies and expands its applicability in clinical and research environments.
In summary, wrapping up the article highlights the vital role that qPCR plays in advancing molecular biology, acknowledging both its current utility and future potential. Researchers and practitioners are encouraged to embrace these insights to optimize their own protocols and seek continuous improvement in their work.
"In a world of ever-evolving scientific techniques, staying informed about the latest advancements in qPCR is key to unlocking new discoveries in molecular biology."
As such, the conclusion encapsulates not only the content of this guide but also serves as a call to action for continuous learning and adaptation within the scientific community.
Citations and Further Reading
Citations are not just a formality; they are essential for communicating the credibility of claims made in scientific papers. Different types of references can aid in various aspects of learning:
- Primary literature provides original research findings, crucial for understanding novel qPCR applications.
- Review articles summarize existing knowledge, making them excellent resources for an overview of qPCR techniques and advancements.
- Protocols and guidelines offer specific methodologies that can enhance consistency and reliability in experiment execution.
Benefits of proper citations in qPCR research include:
- Enhancing reproducibility, as readers can refer to similar studies to confirm the methodology.
- Avoiding plagiarism, ensuring fair acknowledgment of original ideas and findings.
- Fostering collaboration, as sharing quality references among peers leads to more robust discussions and shared discoveries.
In summary, references and citations provide a necessary backbone for the credibility and progress of qPCR studies. For further exploration, researchers might consider the following resources:
- Wikipedia on qPCR
- Britannica on Biological Techniques
- Reddit discussions on molecular biology
In future work, involving a range of references can greatly enhance the understanding and accessibility of qPCR content, ensuring it reaches a broader audience effectively.