Innovative Strategies for Carbon Capture Technologies
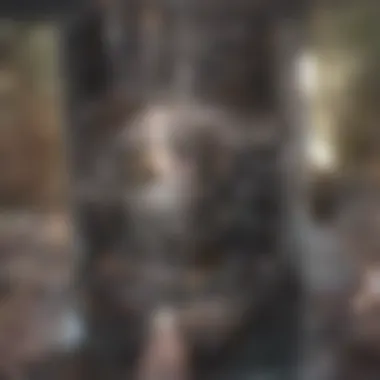
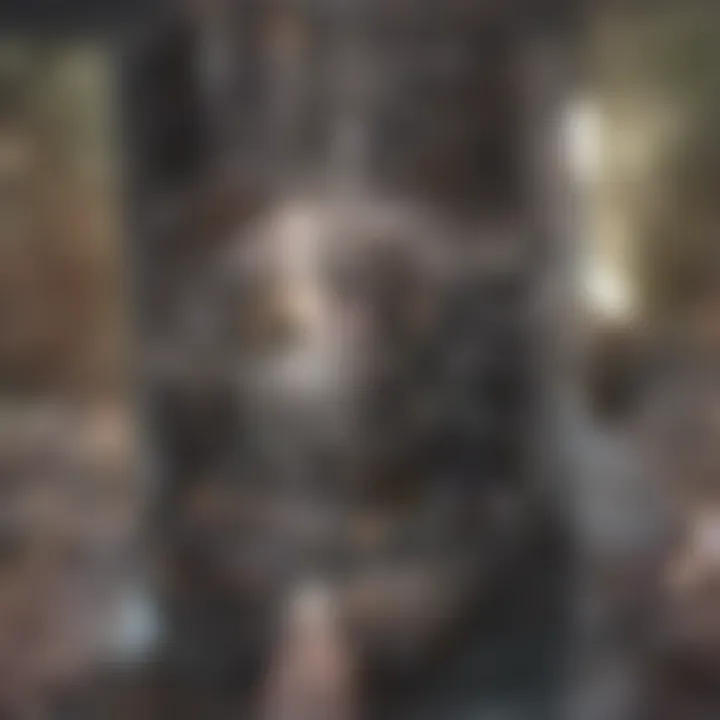
Intro
The accumulation of carbon dioxide in the atmosphere represents a significant challenge in the fight against climate change. With rising concentrations linked to human activities, effective strategies for carbon capture are increasingly important. Understanding the mechanisms, benefits, and challenges associated with these strategies will provide a solid foundation for developing solutions that mitigate atmospheric CO2 levels.
This article delves into various methods of capturing carbon from the air, exploring scientific principles, technological advancements, and future research directions. It emphasizes the need for innovative approaches to address this pressing environmental issue.
Research Background
Overview of the scientific problem addressed
Carbon dioxide is a greenhouse gas that significantly affects global warming. The Intergovernmental Panel on Climate Change (IPCC) has identified the increase in CO2 as a main contributor to climate change. Reducing its concentration in the atmosphere has become a priority for researchers and policymakers alike. Carbon capture technologies are a potential solution to this problem.
Historical context and previous studies
Research on carbon capture dates back several decades, primarily focused on industrial emissions. Early studies centered on techniques such as absorption, membrane separation, and mineralization. More recent advancements involve direct air capture (DAC), a method that extracts CO2 directly from the atmosphere. These developments highlight the importance of evolving technology and methods in the realm of carbon capture.
Findings and Discussion
Key results of the research
The exploration of different carbon capture strategies reveals various approaches that possess distinct advantages and limitations. Current notable methods include:
- Direct Air Capture (DAC): Machines specifically designed to remove CO2 from ambient air.
- Afforestation and Reforestation: Utilizing natural processes by planting trees to sequester carbon.
- Soil Carbon Sequestration: Enhancing organic matter in soil to increase CO2 storage.
Each method presents promising pathways for reducing CO2, but they also come with challenges such as cost, efficiency, and scalability.
Interpretation of the findings
The effectiveness of various methods depends on multiple factors, including technological readiness, economic viability, and environmental impact. Research indicates that DAC, while efficient, remains expensive compared to natural solutions like reforestation. However, as technological innovations emerge, the feasibility of DAC may improve over time.
"Harnessing natureβs capabilities while advancing technology holds the key to successful carbon capture strategies."
Prelims to Carbon Capture
Carbon capture is a critical area of focus in today's environmental discourse. Understanding this topic is essential for acknowledging the consequences of climate change and the necessary actions to mitigate its effects. Carbon dioxide is a major greenhouse gas responsible for global warming, and its accumulation in the atmosphere raises several concerns. Therefore, developing efficient carbon capture strategies is increasingly relevant.
Effective carbon capture offers multiple benefits. It contributes to reducing atmospheric CO2 levels, thus playing a vital role in climate mitigation. Additionally, these strategies provide a pathway to achieving climate targets set by various international agreements. By mitigating emissions, carbon capture technologies can help secure a sustainable environment for future generations.
However, the journey to effective carbon capture is riddled with challenges. Technical feasibility, economic viability, and public perception are key factors that can influence implementation. Future advancements in the field may offer clearer solutions to these challenges, thereby enhancing the potential for carbon capture to play a role in climate solutions.
Significance of Carbon Capture
The significance of carbon capture lies primarily in its ability to combat climate change. The increasing urgency to address climate issues correlates directly with rising levels of atmospheric carbon. By capturing CO2, these technologies can help stabilize the environment and support the transition to a more sustainable energy system.
Furthermore, carbon capture not only reduces existing CO2 levels but also prevents future emissions. This dual impact highlights its importance in developing comprehensive strategies that address both current pollution and potential future risks. Incorporating carbon capture into broader Climate change strategies is thus critical for achieving long-term successes.
"Carbon capture presents a unique opportunity to mitigate climate change while allowing for continued economic growth and development."
Current Atmospheric Carbon Levels
As of now, atmospheric carbon levels are at an alarming high. The concentration of CO2 has surpassed 400 parts per million, a phenomenon largely driven by human activities such as burning fossil fuels, deforestation, and industrial processes. These escalating levels are directly linked to adverse weather events, rising sea levels, and biodiversity loss.
Monitoring current atmospheric carbon levels provides insight into the urgency of implementing carbon capture strategies. Trends indicate that without significant intervention, these levels may continue to rise, exacerbating the already critical problem of climate change. It is imperative to initiate discussions around actionable strategies and investments in carbon capture technologies to reverse this trend.
Fundamentals of Carbon Capture from Air
The foundational concepts of carbon capture hold significant importance in the discussion of effective strategies for reducing atmospheric CO2 levels. Understanding the core principles allows stakeholders to evaluate the effectiveness of different methods and technologies available today. This section delves into the chemistry of carbon dioxide, the thermodynamics involved in its capture, and the implications these factors have on the development of efficient carbon capture systems.
Basic Principles of Carbon Dioxide Chemistry
Carbon dioxide is a colorless, odorless gas naturally present in the atmosphere. Its basic chemical structure consists of one carbon atom bonded to two oxygen atoms. This simple arrangement makes carbon dioxide a stable molecule, capable of existing in various phases: gas, liquid, and solid.
In the context of atmospheric carbon capture, it is essential to recognize that carbon dioxide is produced both through natural processes and human activities, primarily combustion of fossil fuels. When studying the capture of CO2, researchers focus on the following key points:
- Sorption: The attachment of CO2 molecules to solid surfaces. This process is critical in many capture technologies, helping to segregate CO2 from other atmospheric gases.
- Reaction pathways: Various chemical reactions can capture CO2, such as those involving amines and metal oxides. Understanding these pathways can optimize the capture efficiency.
- Phase transitions: The physical state of carbon dioxide can influence its capture and storage methods. For example, capturing CO2 in liquid form may offer higher densities for transportation and storage.
Thermodynamics of Carbon Capture
The thermodynamic principles governing carbon capture are essential for understanding energy requirements and efficiency levels in various processes. Effective carbon capture must consider the balance between energy input and the output of captured CO2.
Key thermodynamic concepts relevant to carbon capture include:
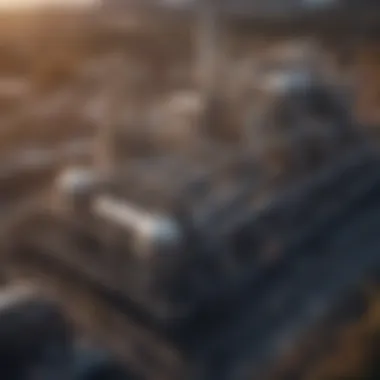
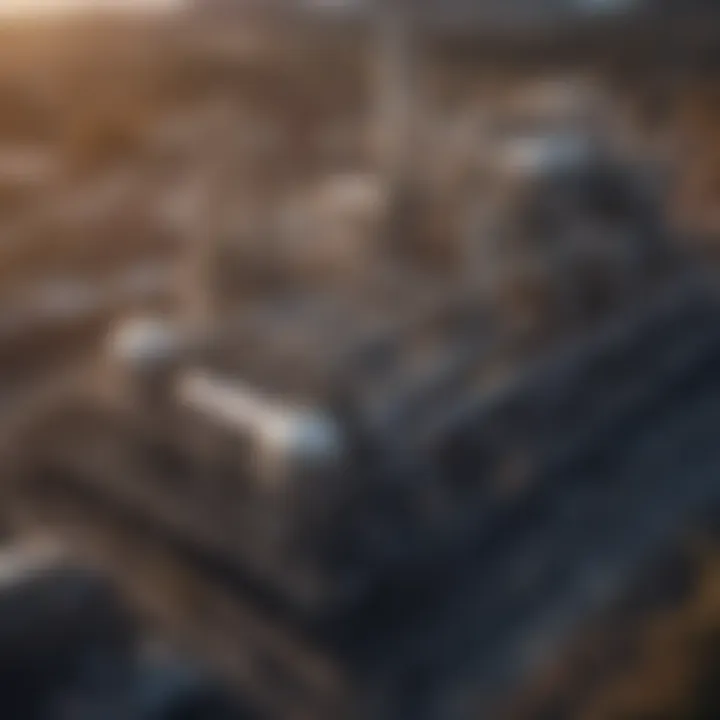
- Gibbs free energy: This indicates whether a reaction process can occur spontaneously. Users of capture technologies must design systems to favor reactions with negative Gibbs free energy changes to enhance capture processes.
- Temperature dependency: The efficiency of many chemical reactions involved in carbon capture is highly temperature-dependent. Higher temperatures can reduce capture efficiency due to undesired side reactions.
- Pressure considerations: Increasing pressure can favor the absorption of CO2 in specific solvents or sorbents, thereby enhancing capture efficacy. Understanding the pressure-volume work is thus crucial for optimizing equipment.
"Understanding the fundamental principles of CO2 chemistry and thermodynamics is crucial for developing effective carbon capture strategies. These factors not only inform technology design but also influence economic viability."
An effective understanding of these principles allows researchers and practitioners to innovate in carbon capture technology. It creates a pathway for advancements in the development of more efficient, cost-effective solutions aimed at addressing the growing challenge of atmospheric carbon.
Direct Air Capture Technologies
Direct Air Capture (DAC) technologies represent a vital component in the multi-faceted approach to combat climate change. They directly target atmospheric carbon dioxide, efficiently capturing this greenhouse gas and mitigating its impact on global warming. As the emphasis on reducing carbon emissions grows, understanding DAC technologies becomes imperative.
Overview of Direct Air Capture
DAC systems operate by chemically binding carbon dioxide from the air, focusing on continuous removal from the atmosphere. This innovation not only has the potential to lower CO2 concentrations but also allows for the utilization of captured carbon in various applications. There are several strategies within direct air capture, including other carbon management practices.
DAC is key to achieving net-zero emissions, as it directly impacts atmospheric concentrations of CO2 while enabling various industrial uses.
In addition to climate benefits, DAC can also generate economic opportunities through carbon credits and partnerships in sustainable industries. However, it requires significant initial investment and technological development.
Mechanical Systems
Mechanical DAC systems utilize large fans to draw in ambient air. Upon entering, the air passes through a series of filters designed to remove carbon dioxide. The primary advantage of this technique lies in its simplicity and reliability, making it suitable for diverse locations. The captured CO2 can either be stored underground or converted for commercial uses, such as in beverage carbonation or synthetic fuel production.
Some key advantages of mechanical systems include:
- Scalable designs allowing adaptation to various site requirements.
- Established supply chain for mechanical components, facilitating easier implementation.
Despite these benefits, operation costs can be high due to energy consumption from fans and systems needed for processing the captured CO2. Therefore, ongoing research is focused on optimizing performance and reducing energy usage.
Chemical Adsorption Techniques
Chemical adsorption techniques leverage specialized materials, known as sorbents, to selectively bind carbon dioxide molecules from the air. These sorbents can be tailored for specific environmental conditions, enhancing their effectiveness in capturing CO2. This method operates based on temperature and pressure variations.
The significant benefits of chemical adsorption include:
- Lower energy requirements compared to some mechanical processes once established.
- High selectivity for CO2 over other gases, reducing contamination in captured streams.
Industries are particularly interested in improving the efficiency of these materials. The development of nanotechnology offers promising improvements in sorbent effectiveness and durability. Continued exploration in this space could lead to substantial advances in DAC technologies, reinforcing their role in climate change mitigation strategies.
Biological Carbon Capture Methods
Biological carbon capture methods harness natural processes to absorb carbon dioxide from the atmosphere. These strategies are critical as they not only mitigate the levels of greenhouse gases but also enhance ecosystem health. Emphasizing biological systems leads to sustainable approaches, utilizing existing natural mechanisms that have evolved over millions of years. The benefits of employing these methods include potential cost-effectiveness, scalability, and alignment with biodiversity goals. Key elements of biological carbon capture involve understanding plant physiology, microbial activity, and soil health.
Role of Photosynthesis
Photosynthesis is fundamental to biological carbon capture. This process involves plants, algae, and certain bacteria converting sunlight, together with carbon dioxide and water, into glucose and oxygen. The most recognized plants, trees for example, play a vital role in sequestering carbon. They absorb carbon dioxide during photosynthesis, effectively lowering atmospheric concentrations.
Moreover, the carbon that is captured gets stored in the plant biomass, including roots, stems, and leaves. Forests, particularly tropical rainforests, have significant capacity for carbon storage. Hence, understanding photosynthesis can lead to strategies that enhance plant growth and biomass accumulation, which in turn improves carbon capture rates.
Optimizing Algal Sequestration
Algal sequestration represents a promising avenue for enhanced carbon capture. Algae, due to their rapid growth rates and high surface area to volume ratio, can absorb carbon dioxide much more efficiently than traditional crops. Utilizing algae in carbon capture involves cultivating them in managed environments, such as photobioreactors. This allows for maximum exposure to sunlight and nutrient-rich water, optimizing their growth.
Furthermore, algae can be processed to produce biofuels, thus offering a dual benefit of carbon capture and renewable energy production. A few species, such as Chlorella and Spirulina, have demonstrated effective carbon uptake. However, challenges remain, such as system scalability and ensuring economic viability. Advances in genetic engineering and biotechnology hold potential to improve algal efficiency in carbon sequestration.
Soil Carbon Enhancement Strategies
Soil carbon enhancement plays a significant role in biological carbon capture methods. Healthy soil acts as a significant carbon sink. Practices such as cover cropping, no-till farming, and organic amendments have proven effective in increasing soil organic carbon stocks.
Regenerative agriculture techniques focus on improving soil health, which directly influences its ability to sequester carbon. For instance, incorporating perennial crops can enhance root systems that stabilize soil and capture carbon beneath the surface. Additionally, enhancing microbial activity through specific soil management practices promotes organic matter formation, further contributing to carbon storage.
Material Innovations in Carbon Capture
The topic of material innovations in carbon capture is critical in addressing the urgent need to mitigate carbon dioxide levels in the atmosphere. These innovations can significantly enhance the effectiveness and efficiency of carbon capture processes. As climate change accelerates, new materials serve as a cornerstone for developing methods capable of capturing atmospheric CO2 at scale. This section discusses advancements in sorbent materials and the role of nanotechnology to illustrate the importance of these innovations.
Advancements in Sorbent Materials
Recent developments in sorbent materials focus on improving their selectivity, capacity, and regeneration potential. Sorbents that exhibit high surface area and porosity are particularly valuable. They enhance the adsorption processes, enabling the capture of more CO2 in smaller volumes. Materials such as metal-organic frameworks (MOFs) and zeolites have emerged as promising candidates. Their intricate structures allow them to bind CO2 molecules effectively.
- Metal-Organic Frameworks (MOFs):
- Zeolites:
- MOFs consist of metal ions coordinated to organic ligands, forming porous structures.
- Their tunable properties make them adaptable to different environmental conditions.
- These are crystalline aluminosilicates with a well-defined pore structure.
- Their stability and reusability make them suitable for industrial applications.
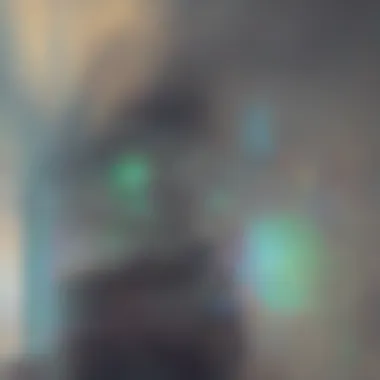
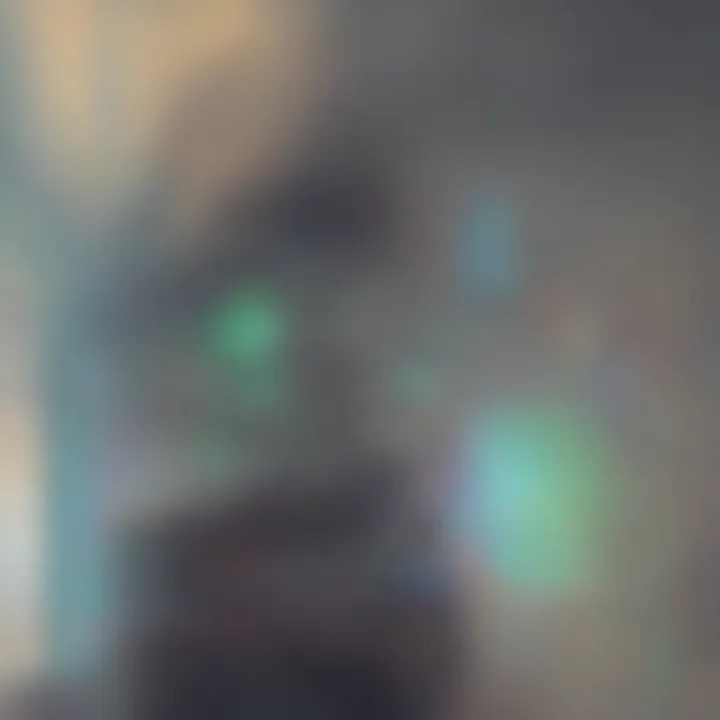
The ongoing research aims to further enhance the efficacy of these materials. Scientists explore new combinations and alterations to existing frameworks, prioritizing the goal of increasing capture rates while minimizing energy costs during regeneration.
Nanotechnology Applications
Nanotechnology presents a frontier in material innovations for carbon capture. By manipulating materials at the nanometer scale, researchers create unique characteristics that can lead to improved performance in carbon capture systems.
- Enhanced Surface Area:
Nanomaterials often possess significantly greater surface area compared to their bulk counterparts. This can lead to increased adsorption sites for CO2. - Targeted Delivery Systems:
Nanoparticles can serve as carriers for catalysts needed in chemical absorption processes. These systems provide more efficient reaction pathways to convert CO2 into useful products. - Self-Regenerating Materials:
Some research focuses on creating materials that can change properties in response to environmental stimuli. This ability can lead to a reduction in energy requirements for regeneration.
"Innovative materials will shape the future of carbon capture, allowing us to harness and store greenhouse gases more effectively."
Overall, the advancements in sorbent materials and nanotechnology applications reveal a profound potential for improving carbon capture strategies. Fostering research in these areas may ultimately lead to more efficient and economical carbon drawdown solutions.
Experimental Evidence and Case Studies
The study of carbon capture technologies demands a solid foundation in experimental evidence and case studies. These elements act as crucial pillars in understanding the efficiency and viability of various methods. By examining real-world applications and outcomes, researchers and stakeholders can evaluate the practical implications of carbon capture solutions. This analysis also helps identify best practices and possible pitfalls, making it essential for guiding future investments in technology and policy formulations.
Investigation in this area yields myriad benefits. Firstly, successful projects provide templates for scalability and adaptation in different geographical contexts, enhancing global capacity to mitigate climate change. Furthermore, lessons drawn from unsuccessful attempts can prove invaluable, helping avoid repeated mistakes and refining strategies for better outcomes.
To conclude, the emphasis on empirical evidence and case study analyses not only enriches academic discourse but also bolsters public policy and funding for innovative carbon capture initiatives. These insights are imperative for capturing and utilizing the ever-increasing quantities of atmospheric CO2, thus contributing significantly to global climate targets.
Successful Projects Globally
A number of successful projects worldwide showcase the potential of carbon capture technologies. These endeavors range from pilot plants to fully operational facilities, proving their capability in reducing CO2 emissions.
- Climeworks: Based in Switzerland, Climeworks operates direct air capture facilities, effectively removing carbon dioxide from the atmosphere. Their Orca plant is noted for capturing around four thousand tons of CO2 annually. Climeworks uses a patented filter technology that absorbs CO2 when air passes through it.
- Calera Corporation: This American company has developed a technology that utilizes CO2 to produce carbonates in concrete production. The process permanently locks away CO2 while generating valuable materials, contributing to reduced emissions in infrastructure.
- The Drax Power Station: Located in the UK, this biomass power station has also ventured into carbon capture and storage (CCS) by blending biomass with fossil fuels. This initiative could lead to negative emissions, a critical factor in climate mitigation efforts.
These successful projects underline the diversity in strategies for carbon capture. They serve as prototypes not only for technology but for potential implementation in various settings, helping shape the future of energy and sustainability practices.
Lessons Learned from Failures
While there are success stories, failures in carbon capture projects reveal essential insights as well. Understanding what went wrong can assist in shaping more effective strategies in the future.
- The TCM Project (Norway): The Technology Centre Mongstad (TCM) aimed to showcase CCS technologies. However, it struggled with high costs and technical issues, ultimately leading to reduced operational capacity. Its lessons include the need for clear economic evaluations before embarking on large-scale projects.
- The Gorgon Project (Australia): The Gorgon LNG project experienced significant delays and cost overruns linked to environmental concerns over carbon storage. This highlighted the importance of transparent dialogue with regulators and the surrounding community regarding environmental impacts.
- SaskPowerβs Boundary Dam: Initially touted as a flagship CCS project, it faced underperformance issues due to mechanical failures and economic challenges. This served as a reminder of the necessity for robust maintenance plans and extensive testing prior to deployment.
These failures can be instrumental in redirecting efforts toward more innovative and sustainable methods. Learning from these cases is crucial for refining technologies and processes, ultimately leading to more successful carbon capture initiatives in the future.
Economic Considerations of Carbon Capture
Economic considerations play a critical role in the adoption and development of carbon capture technologies. The significant investment required for research, development, and implementation means that financial viability is an important factor. This section delves into the essentials of economic analysis regarding carbon capture, focusing on cost-effectiveness, funding trends, and broader economic implications. The viability of these strategies is closely linked to their capacity to deliver demonstrable benefits in the fight against climate change.
Cost-Benefit Analysis
Cost-benefit analysis (CBA) serves as a fundamental tool for assessing the economic feasibility of carbon capture projects. The aim is to weigh the total expected costs against the total expected benefits over a specified period of time. This analysis not only includes the upfront investment but also ongoing operational costs, maintenance expenses, and potential revenues from carbon credits.
In conducting this analysis, key factors to evaluate include:
- Initial Capital Expenditure: This includes costs related to technology purchase, facility construction, and commissioning.
- Operational Costs: Regular expenses necessary to keep the technology functioning, such as energy consumption and labor.
- Environmental Impact: The long-term benefit of reduced carbon emissions, impacting climate change mitigation.
- Market Dynamics: Price fluctuations for carbon credits, which can create revenue streams.
Itβs important to realize that while initial costs may be high, the long-term benefits could greatly outweigh them. Additionally, incorporating external costs associated with climate change can further enhance the case for effective carbon capture strategies.
Funding and Investment Trends
Funding for carbon capture initiatives is becoming increasingly important as global attention turns to climate crisis. Various sectors are investing heavily in this area, motivated by both environmental responsibility and economic opportunity. Here are the prevailing trends:
- Public Financing: Governments worldwide are committing funds to advance carbon capture technologies. Initiatives such as tax incentives and grants can significantly decrease the financial burden on companies pursuing these technologies.
- Private Sector Involvement: Corporations recognize the potential of carbon capture to mitigate their emissions. As businesses strive for sustainability, investment from the private sector is surging, leading to innovative collaboration on funded projects.
- International Collaborations: Global partnerships are forming to share research and development costs, such as those seen in organizations focusing on climate-related technologies.
- Venture Capital: More start-ups are entering the carbon capture space. Venture capital investment is accelerating innovation by funding promising technologies that may otherwise lack the required capital to enter the market.
According to recent reports, global investments in carbon capture technologies are predicted to increase substantially, with public-private partnerships forming a crucial backbone for future advancements in this field.
"Investment in carbon capture is not simply about reducing emissions; it's about positioning in a rapidly evolving market, ensuring that sustainability aligns with profitability."
In wrapping up this section, it is clear that a robust economic framework is foundational to the successful implementation of carbon capture technologies. Understanding the cost-benefit landscape and staying abreast of funding trends are essential for stakeholders aiming to make informed decisions about the future of carbon capture.
Regulatory and Policy Framework
The regulatory and policy framework surrounding carbon capture is critical in shaping the future of air purification technologies. Effective regulations can facilitate the adoption of innovative carbon capture methods, provide funding opportunities, and set targets for carbon reduction. Understanding these policies is essential for researchers and practitioners in this field.
Key elements include international agreements and national policies that guide carbon capture efforts. These agreements not only define the roles of countries in reducing carbon emissions but also promote collaboration in research and development.
International Agreements
International agreements play a pivotal role in setting global standards for carbon mitigation. Treaties such as the Paris Agreement establish overarching goals for carbon reduction among countries. They encourage nations to commit to specific greenhouse gas reduction targets, which indirectly enhances carbon capture initiatives.
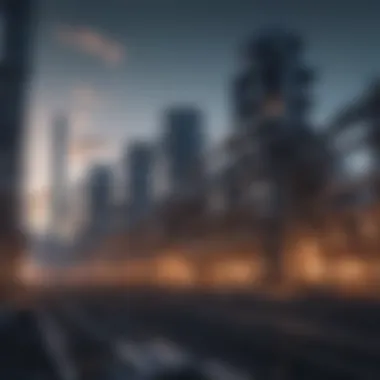
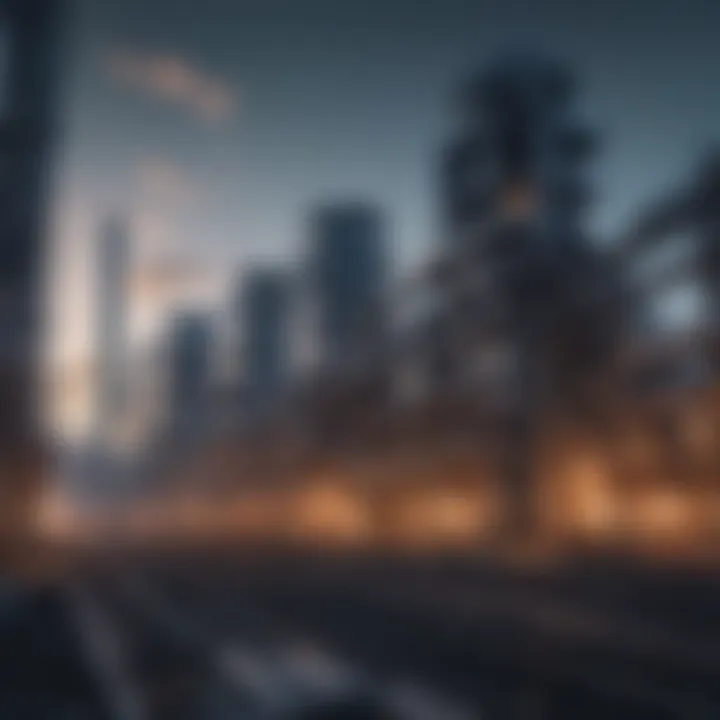
The mechanisms established under these agreements often include:
- Emission trading systems: Allow countries to trade carbon credits, promoting investment into carbon capture technologies.
- Joint mechanisms for emission reductions: Enable collaboration and sharing of best practices across borders.
- Financial aid and technology transfer: Assist developing nations in implementing carbon capture programs.
These agreements create a framework within which countries can develop their strategies for carbon capture. They foster a collective sense of responsibility and often serve as a basis for national legislation.
National Policies and Incentives
National policies are another vital component in establishing a successful carbon capture strategy. Countries typically develop specific regulations and incentives to encourage investments in carbon capture technologies. These might include tax incentives for companies that invest in carbon capture solutions, subsidies for research and development, or grants for pilot projects.
Some critical aspects of national policies include:
- Regulatory frameworks: Clear guidelines on how carbon capture technologies should be implemented and monitored.
- Incentives for innovation: Financial support for companies that develop advanced carbon capture technologies.
- Public engagement strategies: Educating citizens about carbon captureβs benefits and the importance of reducing emissions.
These policies can help reduce barriers that hinder the adoption of carbon capture technology. They also create a favorable business climate that promotes investment and research.
"Effective regulatory frameworks not only promote the deployment of carbon capture technologies but also ensure their transparency and accountability."
Public Perception of Carbon Capture
Public perception of carbon capture plays a pivotal role in the advancement and implementation of these technologies. The effectiveness of carbon capture efforts is not only determined by scientific and technological advancements but also by how they are viewed by society. Thus, it is critical to understand the beliefs, attitudes, and acceptance levels of different communities towards carbon capture. This comprehension can steer the direction of policy-making, funding allocation, and innovation in carbon capture technologies.
Awareness and Education
The first step in shaping public perception is enhancing awareness about carbon capture technologies. Many individuals are still unfamiliar with the basic principles and benefits of carbon capture. Education serves as a powerful tool in transforming perceptions. Educational initiatives can take various forms, ranging from informational campaigns to inclusion in academic curriculums.
- Targeted Communication: Providing clear, concise information about how carbon capture works can demystify the technology. This can include visual aids, interactive displays, or informative videos that simplify complex concepts.
- Community Engagement: Hosting local workshops or public forums can help in disseminating knowledge while allowing for community input. Engaging with stakeholders at various levels ensures the concerns and interests of the public are addressed.
- Partnerships with Institutions: Collaborating with schools, universities, and non-profit organizations can amplify outreach efforts. These partnerships can foster a culture of scientific inquiry and environmental responsibility.
Challenges of Acceptance
Despite educational efforts, several challenges exist in achieving widespread public acceptance of carbon capture initiatives. Misunderstandings about the risks and impacts associated with these technologies may breed skepticism and resistance.
- Misinformation: The proliferation of false information can severely hinder acceptance. Fact-checking and providing scientifically-backed data is crucial in countering myths surrounding carbon capture.
- Perceived Risks: Communities may express concerns over safety, environmental impacts, or potential economic disadvantages posed by carbon capture technologies. Addressing these fears through transparent communication and inclusive discussions can help alleviate concerns.
- Equity and Accessibility: The implementation of carbon capture strategies must consider social equity. There can be apprehensions about who benefits most from these technologies. A clear framework for equitable benefits can enhance trust and acceptance among various demographic groups.
Future Directions in Carbon Capture Research
The realm of carbon capture is at a pivotal juncture, where ongoing research will determine the effectiveness of future strategies. Advancements in carbon capture technology hold potential to mitigate climate change significantly. Understanding these future directions is vital, especially as carbon dioxide levels continue to rise. This section dissects the emerging technologies and emphasizes the value of interdisciplinary methodologies in furthering research thereby enhancing practical applications.
Emerging Technologies
New technologies are constantly surfacing in carbon capture. These innovations are crucial for scaling successful methods. One promising development is the use of advanced sorbent materials. These materials can capture carbon more efficiently at lower costs. For example, metal-organic frameworks (MOFs) have shown high capacity and selectivity for CO2.
Another area of research focuses on direct and indirect air capture systems which utilize cutting-edge filtration techniques. These systems not only enhance efficiency but can potentially integrate renewable energy sources.
"Innovations in technology are essential to make carbon capture economically feasible on a larger scale."
Additionally, bioengineering is gaining traction. Modified organisms can harness natural processes, like photosynthesis, to optimize carbon capture from the atmosphere. This bio-inspired approach may offer unique solutions that are both sustainable and effective in the long term.
Interdisciplinary Approaches
Addressing the challenges of carbon capture requires input from various fields. By merging the expertise of scientists, engineers, economists, and psychologists, a more comprehensive strategy emerges. Interdisciplinary collaboration fosters holistic understanding and problem-solving.
For instance, researchers in environmental science can work with political scientists to formulate effective policies that promote carbon capture technologies. If public acceptance is low, psychologists can help tailor communication strategies that inform and engage communities.
Moreover, engineers must collaborate with chemists to enhance the design of carbon capture systems, ensuring that they are both effective and sustainable. This teamwork ultimately leads to innovative solutions that address multiple dimensions of the problem simultaneously.
In summary, the future of carbon capture research is hopeful. Continued innovation in technology combined with interdisciplinary collaboration can significantly improve our capacity to manage atmospheric carbon levels. The success of these endeavors will contribute directly to global climate change mitigation efforts.
Closure
In examining the multifaceted approaches to carbon capture, the importance of the conclusion becomes evident. It ties together the significant findings from diverse sections of this article and reinforces the urgency of addressing atmospheric carbon levels. The strategies for carbon capture are not merely technological solutions; they represent fundamental shifts in our approach to climate change.
Summary of Key Findings
The article outlined crucial insights:
- Direct Air Capture Technologies: Innovations such as mechanical systems and chemical adsorption are pivotal in removing CO2 from the atmosphere.
- Biological Methods: Leveraging natural processes like photosynthesis and optimizing algal sequestration shows the potential of biological interventions in carbon capture.
- Economic Viability: It is essential to evaluate the cost-effectiveness of these methods and to understand funding trends. Investment in carbon capture technologies could accelerate their adoption and efficacy.
- Policy Framework: Regulatory support and international cooperation are vital for fostering technological advancements and their deployment at a global scale.
- Future Directions: Ongoing research must prioritize interdisciplinary approaches and emerging technologies to enhance the efficiency of carbon capture initiatives.
These findings underscore the necessity for a holistic approach in tackling carbon emissions, ensuring that each method complements the others towards a singular goal of reducing atmospheric CO2.
Call to Action for Ongoing Research
The need for ongoing research in carbon capture strategies is both critical and urgent. Future studies should delve into the following areas:
- Innovating Materials: Research should continue on sorbent materials to improve absorption capacities and reduce costs.
- Scaling Up Technologies: Efforts to pilot and implement carbon capture projects at scale will provide valuable data and insights into their practicality and effectiveness.
- Public Engagement: Understanding public perception and overcoming resistance to new technologies will bridge the gap between research, policy, and implementation.
- Global Collaboration: Scientists and policymakers must work together internationally to share best practices and accelerate technological transfer among countries.
The time to act is now. Continued investment in research not only advances the specific field of carbon capture but also serves to instill hope in our global trajectory toward mitigating climate change.
Through relentless pursuit and collaboration, we can transform our understanding of carbon capture into actionable strategies that preserve the planet for future generations.