Thermal Structures: Exploring Their Impact and Importance
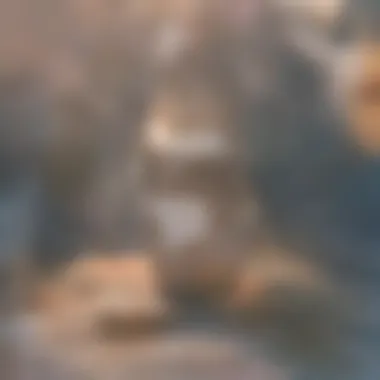
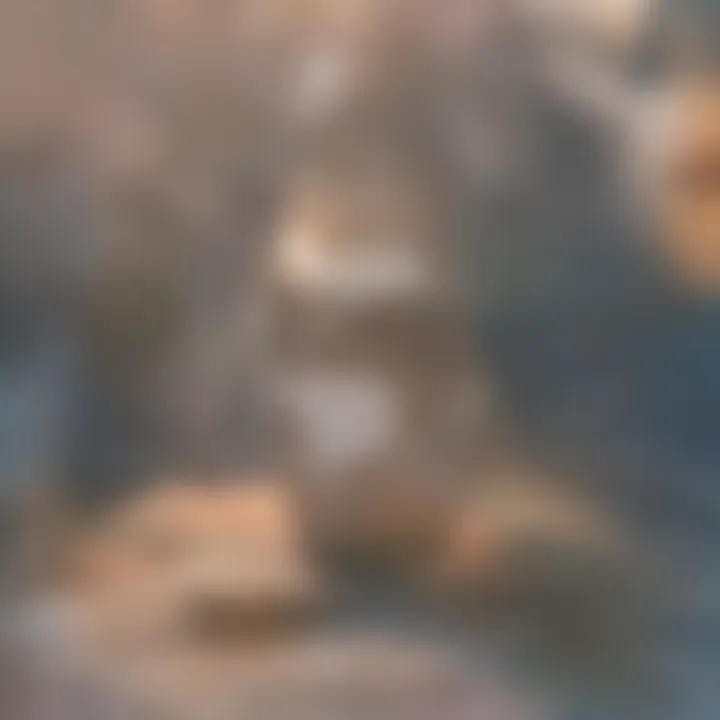
Research Background
Understanding thermal structures initiates with the acknowledgment of heat flow and its implications in various scientific domains. These structures are primarily pivotal in structural engineering, environmental science, and material science. The fundamental problem addressed is how to manage heat to enhance performance, efficiency, and sustainability across numerous applications.
Historically, the study of thermal dynamics has evolved significantly. From early observations of natural phenomena to the development of sophisticated computational models, researchers have built a substantial foundation. Studies by figures such as Fourier and Newton laid groundwork for understanding heat transfer.
Technological advancements have catalyzed this evolution. For instance, the introduction of new materials, such as phase change materials and high-thermal-conductivity composites, has transformed thermal structures, providing new avenues for exploration. Previous studies have consistently emphasized not just on efficiency but also on environmental impact.
Findings and Discussion
Recent research findings showcase a myriad of benefits from optimizing thermal structures. Key results indicate improved energy efficiency in buildings, reduced waste in manufacturing processes, and better performance of electronic devices. These results not only highlight the importance of thermal control but also stress its relevance in achieving sustainability goals.
The interpretation of these findings reveals that effective heat management significantly elevates the lifespan of structures and machinery. For example, buildings designed with enhanced thermal insulation contribute to lower energy consumption, aligning with global sustainability goals. Projects like the Passive House standard illustrate how effective thermal structures lead to minimal energy needs for heating and cooling.
Moreover, the integration of renewable energy sources in thermal structure design emphasizes the importance of collaboration between different scientific disciplines. Such approaches reflect a holistic understanding of the environment, technology, and societal needs and push the boundaries of innovative thinking.
"The insights gained from studying thermal structures pave the way to not only meet human needs but to also respect the delicate balance with our ecosystem."
To conclude, the discourse surrounding thermal structures is complex yet crucial. Their application is indispensable for progress in many sectors. Continued research will refine our understanding, enabling more sustainable practices and innovative solutions.
Preamble to Thermal Structures
Thermal structures play a pivotal role in numerous fields, such as engineering, architecture, and environmental science. Understanding these structures is not limited to their physical form; it encompasses the underlying principles that govern their behavior in the presence of heat. The design, functionality, and implementation of thermal structures influence energy efficiency, sustainability practices, and overall performance in both natural and engineered systems.
In this section, we explore the significance of thermal structures, their complex interplay with thermal dynamics, and the critical considerations that influence their design and function. The importance of this discussion is rooted in the lessons of past innovations and the ongoing quest for improved thermal management strategies.
Definition and Importance
Thermal structures can be defined as systems specifically designed to manage heat transfer in their environment. This includes not only their material properties but also their shape, size, and orientation. Understanding thermal dynamics is essential for researchers and practitioners alike, as it informs the selection and arrangement of materials and techniques.
The importance of thermal structures lies in their ability to influence a range of outcomes. These structures affect energy consumption, environmental impact, and durability of buildings, vehicles, and various products. For instance, passive solar design optimizes natural heating without relying solely on mechanical systems, thus reducing reliance on fossil fuels. The exploration of various thermal management strategies, enhances resilience against climate variability, ultimately playing a crucial role in sustainability efforts.
Historical Context
The origins of thermal structure design can be traced back to ancient civilizations. For instance, the use of passive heating techniques in ancient Rome showcased a remarkable understanding of heat transfer principles. Romans utilized thermal mass in their structures to absorb heat during the day and release it at night.
Over the centuries, various cultures derived methods to harness or manipulate heat. The Industrial Revolution introduced new materials and techniques that transformed thermal engineering. Innovations such as the steam engine marked a turning point, expanding the application of thermal principles beyond buildings to transport and manufacturing.
In modern times, the urgency around energy conservation and climate change has reinvigorated the field of thermal structures. Recent advancements focus on integrating emerging materials such as phase change materials and smart technologies to improve thermal efficiency. This historical progress is essential for understanding the current landscape and inspiring future innovations.
"The true measure of any structureβs performance is not merely its ability to house occupants but its efficacy in managing thermal dynamics effectively."
Fundamental Principles
Thermal structures play a critical role in managing heat in various contexts. Understanding the fundamental principles behind thermal dynamics is essential for those involved in engineering and design. The concepts of thermodynamics and heat transfer mechanisms are interwoven and lay the groundwork for effective thermal management.
These principles help in deciding how structures react to heat changes. Utilizing this knowledge ensures that materials respond suitably to temperature variations. Furthermore, understanding heat transfer mechanisms enables engineers to predict how heat moves through materials, which is crucial for applications ranging from buildings to aerospace. A solid grasp of these principles ultimately leads to more efficient designs, better performance, and greater sustainability in engineering practices.
Thermodynamics Overview
Thermodynamics is the branch of physics that deals with heat and temperature, in relation to energy and work. It is fundamental to the study of thermal structures because it offers insight into energy transformations and heat transfer within systems. The laws of thermodynamics guide engineers in understanding how energy flows in structures. The first law emphasizes the conservation of energy, while the second law addresses the direction of energy transfer and the potential for extracting work from heat. Understanding these concepts is critical for optimizing energy efficiency and minimizing waste, which is particularly relevant in modern sustainable practices.
Heat Transfer Mechanisms
Heat transfer is a process that can occur through different mechanisms: conduction, convection, and radiation. Each mechanism has distinct characteristics and applications in the context of thermal structures.
Conduction
Conduction is the transfer of heat through direct contact between materials. It occurs at the molecular level as the high-energy molecules collide with lower-energy molecules, imparting heat. The key characteristic of conduction is its ability to transfer heat through solid materials with great effectiveness, making it a popular choice in thermal management. For instance, metals like copper and aluminum, which are excellent conductors, are often used in applications where efficient heat dissipation is essential.
Advantages of conduction include its efficiency in transferring heat across short distances. However, it may have limitations in scenarios where materials do not have high thermal conductivity, leading to slower heat transfer rates. Thus, understanding the balance of materials is crucial for optimizing performance in thermal structures.
Convection
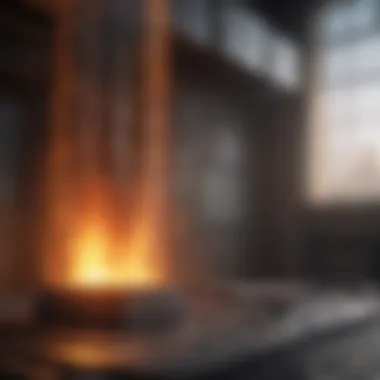
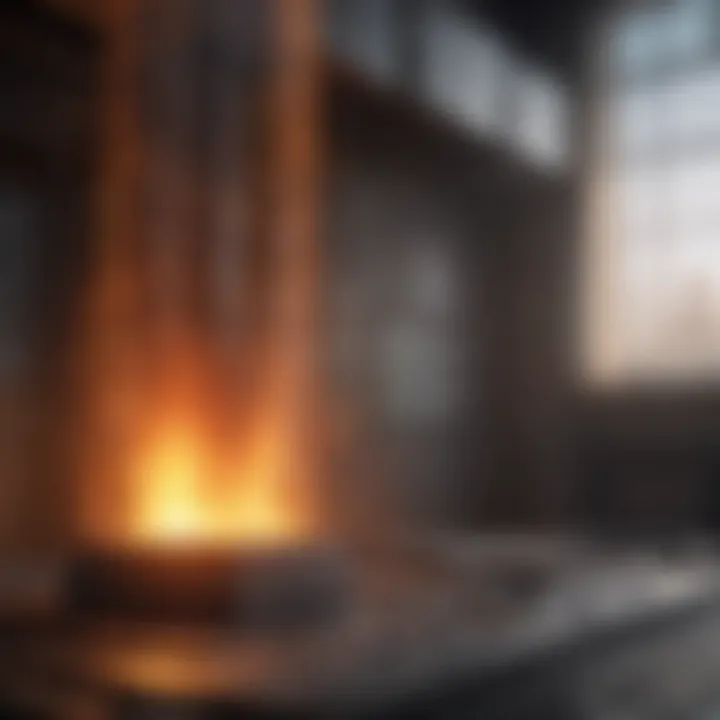
Convection is the transfer of heat by the movement of fluids, including liquids and gases. This mechanism can be natural, such as when warm air rises and cool air descends, or forced, where external means like fans or pumps accelerate fluid flow. The primary characteristic of convection lies in its capacity to move larger volumes of heat than conduction.
Convection is particularly useful in various engineering applications, including heating and cooling systems of buildings. Its unique feature is the various design strategies available, such as the use of fins or other surface enhancements that improve heat exchange efficiency. However, the disadvantages of convection include dependence on fluid characteristics, which can change under different operating conditions, thus affecting performance.
Radiation
Radiation is the transfer of heat in the form of electromagnetic waves, such as infrared radiation. Unlike conduction and convection, radiation does not require a medium, allowing it to occur in a vacuum. The key characteristic of radiation is how quickly it can transfer heat over long distances.
This mechanism plays a crucial role in heat management strategies. For example, it is significant in passive solar design, where structures utilize solar radiation to maintain comfortable indoor temperatures. The unique feature of radiation is its reliance on surface emissivity, which can affect how much energy is absorbed or emitted. One disadvantage of radiation is that its effectiveness diminishes with distance, requiring careful placement of absorptive surfaces to optimize thermal performance.
Overall, understanding these heat transfer mechanisms is vital for engineers and designers working in thermal structures. Each mechanism presents its own set of benefits and challenges, influencing material selection and design choices in thermal management.
Material Considerations
Importance of Material Considerations
Material considerations play a critical role in the effectiveness of thermal structures. The right material choice influences not only the thermodynamic performance but also the overall durability and sustainability of structures. Understanding various types of thermal materials is essential for optimizing heat management strategies. Materials impact how heat is conducted, insulated, or stored, which directly affects energy efficiency. Utilizing the appropriate materials in design can lead to significant advancements in both engineered and natural environments.
Types of Thermal Materials
Conductors
Conductors possess the ability to transfer heat efficiently. This characteristic makes them crucial in applications where rapid heat transfer is necessary. Metals like copper and aluminum are commonly used conductors due to their high thermal conductivity.
One key feature of conductors is their low resistance to heat flow, allowing temperatures to equalize quickly across surfaces. For instance, in HVAC systems, using conductors can enhance the effectiveness of heat exchangers, contributing to better climate control within buildings, making it a beneficial choice for effective thermal management.
However, conductors also come with disadvantages. They can lead to heat loss in non-required areas, impacting energy efficiency. Managing this loss is important to maintain system performance.
Insulators
Insulators serve the opposite purpose of conductors. Their primary role is to resist heat flow. Materials like fiberglass, foam, and specific ceramics exemplify effective insulation. These materials prevent unwanted heat transfer, maintaining desired temperatures within a structure.
The key characteristic of insulators is their high thermal resistance. Insulators are often utilized in building envelopes to enhance energy efficiency, minimizing heating and cooling demands. This makes them particularly popular for sustainable construction practices.
Nevertheless, insulators also have unique features that present challenges. Over time, certain insulating materials can degrade or lose their effectiveness, leading to increased energy costs. Proper selection and maintenance are needed to maximize their benefits.
Phase Change Materials
Phase change materials (PCMs) uniquely absorb and release thermal energy during phase transitions. Their primary advantage lies in their ability to store heat during one phase and release it during another. This property makes them useful in regulating thermal performance in buildings and other applications.
One key characteristic of PCMs is their latent heat storage capability. Such materials can help stabilize temperature fluctuations, improving comfort levels while reducing energy consumption. In this context, they are increasingly seen as beneficial for energy conservation efforts.
However, they also have disadvantages, such as higher costs compared to traditional insulation strategies. Their effectiveness is also highly dependent on the ambient conditions, which necessitates careful evaluation prior to implementation.
Material Properties Affecting Thermal Performance
The thermal performance of materials is governed by several intrinsic properties, including thermal conductivity, specific heat capacity, and density. These properties dictate how effectively materials conduct, store, and dissipate heat.
- Thermal Conductivity: Determines how quickly heat transfers through a material. A high thermal conductivity indicates efficient heat transfer, whereas a low value means better insulation.
- Specific Heat Capacity: Indicates the amount of energy required to raise the temperature of a unit mass of material by one degree Celsius. Materials with high specific heat can store more energy.
- Density: Influences how much heat a material can hold. Generally, denser materials can store more energy, but this also varies with other properties.
Design Methodologies
Design methodologies in the context of thermal structures are essential to ensure effective thermal management. These methodologies guide engineers and designers in planning, analyzing, and optimizing thermal performance in various applications. Whether in building engineering or aerospace, the consideration of thermal design is crucial for safe and sustainable solutions.
Effective thermal design criteria are based on several factors. First, it is necessary to understand the specific thermal requirements of a project. This includes factors such as environmental variables, heat loads, and the desired performance outcomes. By analyzing these criteria, designers can select suitable materials and construction techniques that promote energy efficiency while maintaining structural integrity.
Moreover, integrating sustainability into design methodologies is increasingly important. This means not just focusing on immediate thermal performance, but also considering long-term impacts on energy consumption. Engineers must balance initial costs with ongoing operational expenses, ensuring that thermal structures contribute positively to environmental goals.
Thermal Design Criteria
When developing thermal structures, several design criteria should be considered:
- Thermal Resistance: The ability of the structure to resist heat flow is fundamental. This can be achieved through proper insulation strategies.
- Thermal Mass: Materials that can store heat effectively help maintain stable temperatures within structures. Concrete, brick, and stone are often used for this purpose.
- Adjustable Features: Incorporating elements that can adjust to varying heat loads can enhance a structure's thermal efficiency. For example, adjustable shading devices can control sunlight exposure.
- Energy Efficiency Standards: Compliance with international and national standards is essential. Adhering to guidelines such as the ASHRAE standards for energy efficiency helps meet performance goals.
"Designing thermal structures requires a careful balance of performance, cost, and sustainability."
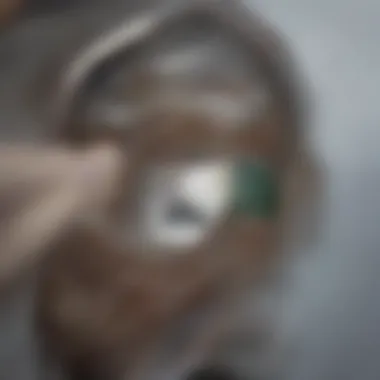
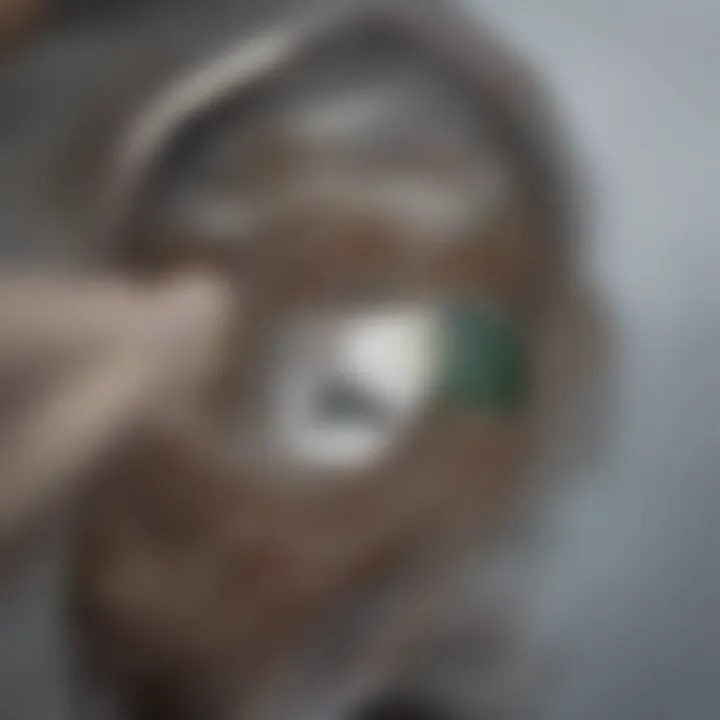
Modeling and Simulation Tools
To build effective thermal structures, modeling and simulation tools are integral. These tools allow for detailed analysis of thermal dynamics within a structure before construction begins. By simulating various scenarios, designers can predict performance under different conditions.
Several software tools are used in this field. Programs like ANSYS and COMSOL Multiphysics enable engineers to visualize heat flows, assess thermal resistance, and study interactions between various materials. These simulations provide valuable insights that help optimize designs.
The benefits of these tools include:
- Predictive Analysis: They enable predicting how materials will behave under different thermal loads.
- Iterative Design: Changes can be easily made and tested without costly physical prototypes.
- Risk Reduction: Identifying potential issues during the design phase reduces the risk of failures after construction.
By integrating rigorous modeling and simulation processes, designers can develop thermal structures that are not only efficient but also resilient to the challenges posed by varying environmental conditions.
Applications of Thermal Structures
Understanding applications of thermal structures is essential. These applications reveal how thermal dynamics influence everyday engineering solutions. From buildings to aerospace, thermal structures impact energy consumption, efficiency, and sustainability. They also dictate performance and safety in different environments. Exploring their applications not only showcases their importance but also offers insights into future advancements.
In Building Engineering
HVAC Systems
HVAC Systems, or Heating, Ventilation, and Air Conditioning systems, play a crucial role in maintaining comfort. They regulate indoor climates efficiently. One specific aspect of HVAC Systems is energy efficiency. Energy-efficient designs minimize consumption and direct costs. This characteristic appeals to builders and homeowners. Additionally, modern HVAC Systems often integrate smart technology. This technology enhances user control and optimizes performance.
The unique feature of HVAC Systems is their ability to adapt. Systems can tailor heating and cooling based on occupancy and external climate. However, they require regular maintenance for optimal performance. This characteristic can be a downside, particularly for older systems that may inefficiently consume energy.
Passive Solar Design
Passive Solar Design focuses on maximizing natural heat. This design helps reduce reliance on mechanical heating and cooling devices. A key characteristic of this design is its integration with building orientation and materials. For instance, strategically placed windows allow sunlight to warm spaces. This practice is a popular choice for sustainable building projects.
A unique feature of Passive Solar Design is its cost-effectiveness over time. It often reduces energy bills significantly. However, effective Passive Solar Design requires careful planning. If not executed correctly, it can lead to undesirable heat gain or loss. Overall, these features make Passive Solar Design an innovative solution for energy-conscious builders.
Role in Aerospace Engineering
Thermal Protection Systems
Thermal Protection Systems (TPS) are vital in aerospace engineering. They protect spacecraft from extreme temperatures during launch and re-entry. A specific aspect of TPS is material selection. Advanced materials withstand high heat without degrading. This characteristic is crucial for ensuring the safety of missions.
The unique feature of TPS lies in their design. Systems can be tailored for specific missions, enhancing resilience. However, development can be expensive and time-consuming. These challenges often lead to delays but are necessary for ensuring reliability during operations.
Heat Shield Technologies
Heat Shield Technologies involve designs that dissipate heat effectively. They play a key role during atmospheric re-entry. A notable characteristic of these technologies is their use of ablative materials. These materials char and erode away, absorbing heat. Because of this, they serve as effective barriers against heat.
One unique feature of Heat Shield Technologies is their adaptability. Modern shields can be engineered to meet the demands of various spacecraft. However, these technologies also face challenges. The durability of materials can vary under differing conditions, impacting performance. Overall, these technologies make significant contributions to safe space travel.
Environmental Considerations
The realm of thermal structures extends beyond engineering disciplinary boundaries, intersecting significantly with environmental sustainability. Understanding environmental considerations is crucial as it is here that thermal structures contribute to energy efficiency and sustainable practices.
Impact on Energy Efficiency
Energy efficiency represents a cornerstone of contemporary sustainability initiatives. In the context of thermal structures, effective heat management can drastically reduce energy consumption in both residential and commercial settings. Poorly designed thermal systems can lead to substantial energy loss, increasing operational costs and environmental impact. In contrast, optimal thermal design minimizes this loss, ensuring that energy consumption aligns with the buildingβs functional requirements.
Key benefits include:
- Reduced Operational Costs: By employing high-efficiency thermal materials and optimizing design, buildings can save significant amounts on energy bills.
- Lower Greenhouse Gas Emissions: Efficient energy use directly correlates with reduced emissions, making buildings more sustainable.
- Improved Comfort: Effective thermal structures maintain consistent internal temperatures, enhancing occupant comfort.
Advanced technologies like insulation made from recycled materials or reflective roofing significantly enhance energy efficiency. These innovations directly contribute towards achieving globally recognized energy standards such as LEED (Leadership in Energy and Environmental Design).
Sustainable Thermal Practices
Sustainable thermal practices focus on integrating environmental principles into the design and execution of thermal structures. This means not only selecting appropriate materials but also considering their life cycle and long-term implications.
Considerations include:
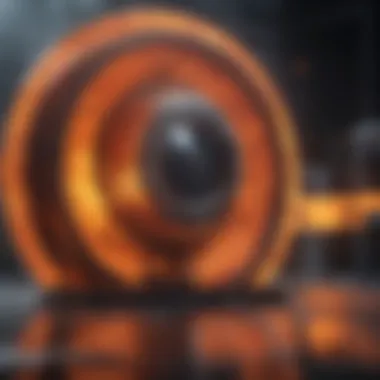
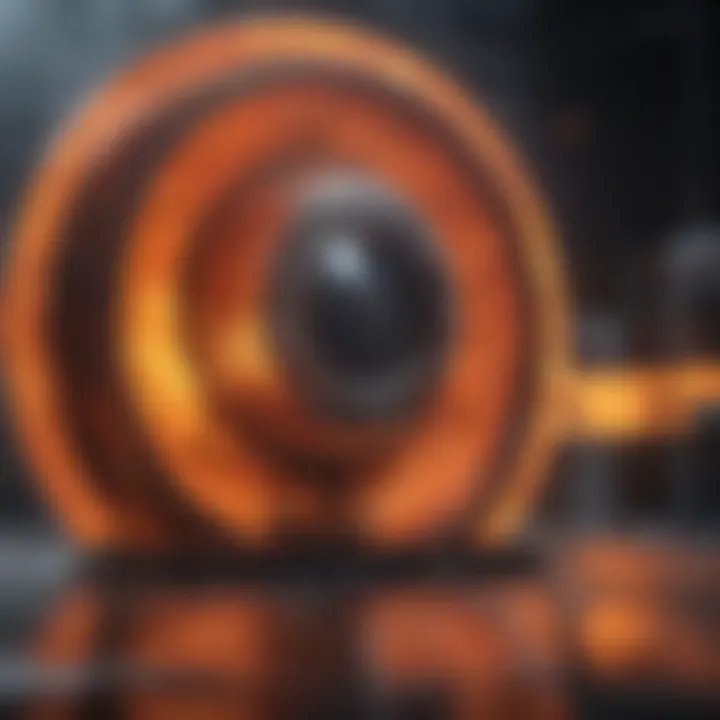
- Material Selection: Using renewable or recycled materials diminishes the environmental footprint of thermal systems. Materials like cellulose insulation or green concrete are examples.
- Lifecycle Analysis: Analyzing the energy and materials consumed in the entire lifecycle of thermal structures, from extraction to disposal, leads to more informed decisions.
Engaging in research for innovative solutions is crucial. Emerging trends like phase change materials or thermally activated building systems suggest a forward-thinking approach to temperature control and energy management.
"By integrating sustainable practices in thermal design, we not only enhance efficiency but also ensure long-term viability for future generations."
As we move forward, the integration of sustainability into thermal structures will not only improve efficiency but will also drive innovation. Awareness and action towards these environmental considerations can lead to substantial improvements in energy use and the overall ecological footprint of various structures.
Challenges in Thermal Structure Engineering
The field of thermal structure engineering faces a multitude of challenges that significantly affect design and performance. With the increasing complexity of thermal management systems, understanding these challenges becomes essential for students and professionals alike. The importance of addressing these issues cannot be overstated, as they directly impact efficiency, sustainability, and functionality of structures in various applications. This section highlights the common issues faced in design and implementation, along with the future challenges that require research and innovation.
Common Issues in Design and Implementation
When engineers design thermal structures, several common issues arise that may hinder the ultimate performance of these systems. These issues often stem from inadequate understanding of thermal properties, material selection, and integration with existing frameworks.
- Material Limitations: Many materials have inherent thermal properties that do not always align with the performance requirements of a specific application. For instance, a material may have excellent insulative properties but lacks mechanical strength, making it unsuitable for structural support.
- Thermal Bridging: This issue occurs when a highly conductive material creates a path for heat to flow through a thermal insulator, thus undermining the purpose of thermal barriers. Designers must carefully evaluate node connections to prevent problematic heat flow paths.
- Temperature Variability: In many applications, variations in temperature can lead to expanded or contracted materials, impacting structural integrity. Engineers must consider these effects during the design phase.
- Component Interaction: The interactions between different parts of a system can lead to unexpected results. Thermal expansion of one component can affect its neighbors, leading to thermal stress and potential failure of the structure.
The potential consequences of overlooking these issues can include increased energy costs, reduced lifespan of materials, and ultimately, failure of the thermal structure.
Future Challenges and Research Directions
The landscape of thermal structure engineering is swiftly changing. As new technologies emerge, they bring forward new challenges that demand innovative solutions. Here are some future challenges and directions for research in this field:
- Advanced Materials Development: Research into materials that can maintain thermal efficiency while being durable and cost-effective is essential. Materials that can reduce energy consumption and improve thermal performance are in high demand.
- Integration of Smart Technologies: As automation and smart technologies advance, integrating these into thermal structures presents both challenges and opportunities. Engineers will need to develop systems that not only monitor thermal performance but also adapt to changes in real-time.
- Sustainability Considerations: Engineers are increasingly tasked with ensuring that thermal structures contribute to environmental sustainability. This involves developing systems that minimize waste and energy use while maximizing performance.
- Predictive Modeling and Simulations: With the complexity of thermal structures, advanced modeling tools will be crucial for predicting behaviors under various conditions. Research must focus on refining these models to enhance precision.
"Innovation in thermal structure engineering cannot come without addressing the fundamental challenges first. Ignoring them might lead to obsolescence in designs and practices."
These challenges require collaborative efforts from academia and industry to push the boundaries of thermal structure technology. Exploring these areas will not only foster advancements but also ensure that future thermal structures meet emerging societal needs in a sustainable manner.
Advancements in Thermal Structure Technology
The study of thermal structures continues to evolve, as innovative developments in technology push the boundaries of design and functionality. This section focuses on how advancements in thermal structure technology are reshaping various industrial sectors, enhancing efficiency and sustainability in heat management systems. The integration of new materials and automation in thermal architectures is notable.
Innovative Materials and Techniques
Emerging materials are crucial in enhancing the performance of thermal structures. Traditional materials like concrete and steel have served well, but the increasing demand for energy efficiency has driven research towards innovative alternatives.
Materials such as aerogels and phase change materials are gaining traction. Aerogels are known for their outstanding insulative properties due to their low density, which makes them highly effective in minimizing thermal transfers. Meanwhile, phase change materials have the unique ability to absorb or release heat during phase transitions, thus stabilizing temperature in a controlled environment.
The use of nanotechnology is also becoming prevalent. Nanocomposites improve strength while maintaining lightweight characteristics. This combination is invaluable in applications where weight reduction is imperative, such as in aerospace engineering.
"Innovative materials can significantly reduce heat loss while improving structural integrity and safety."
The exploration of self-healing materials shows promise as well. These materials can repair themselves when damaged, extending their lifespan and reliability. Research into biomimetic techniques, inspired by natural processes, is also contributing to advancements in thermal structure technology.
Automation and Smart Thermal Systems
Automation plays a pivotal role in enhancing the efficiency and effectiveness of thermal structures. Smart thermal systems incorporate sensors, machine learning, and IoT technology to continuously monitor and adjust thermal performance. This real-time feedback mechanism enables automatic adjustments to heating, cooling, and insulation strategies based on current conditions.
Utilizing systems such as thermostats coupled with machine learning algorithms allows for optimized energy consumption. These systems learn users' habits over time, adjusting temperature settings to maximize both comfort and efficiency.
The advent of building management systems further enhances this capability. These systems unify control of HVAC, lighting, and other related components, thus improving overall energy performance and reducing operational costs. The significance of automation in mitigating environmental impact cannot be overstated as it optimizes resource usage, ultimately leading to lower carbon footprints.
The End
The conclusion serves as a critical component of this article, encapsulating the essence of thermal structures and their diverse contributions across multiple disciplines. Understanding the implications of thermal structures extends beyond mere academic interest; it is crucial for practical applications in fields such as architecture, aerospace, and environmental science. The insights provided herein reinforce the importance of heat management methodologies and material selection in crafting effective thermal solutions.
Summary of Key Points
In summary, the article delves into various facets of thermal structures, shedding light on:
- Definition and Importance: It establishes the foundational understanding of thermal structures and their vital role in both natural and engineered environments.
- Fundamental Principles: A comprehensive overview of thermodynamics, as well as heat transfer mechanisms, setting the stage for subsequent discussions.
- Material Considerations: This section highlights the types of materials used in thermal structures, focusing on conductors, insulators, and phase change materials. Additionally, it emphasizes the significance of material properties in overall thermal performance.
- Design Methodologies: Addressing crucial design criteria and the tools available for modeling and simulation, which aid in the development of effective structures.
- Applications: The article explores applications in building engineering and aerospace, pinpointing specific systems that utilize thermal structures effectively.
- Environmental Considerations: Discussing the impact of thermal structures on energy efficiency and the promotion of sustainable practices.
- Challenges: Outlining common issues faced in engineering thermal structures and directions for future research.
- Advancements: Highlighting innovative materials and the role of automation in enhancing thermal system performance.
Implications for Future Research
Looking ahead, it is clear that the study of thermal structures holds vast potential for advancements in both theoretical and practical dimensions. Research can focus on:
- Emerging Materials: Investigating new materials that offer improved thermal properties, potentially leading to more efficient designs.
- Smart Systems: The integration of IoT technologies to create adaptive thermal management systems that respond dynamically to changing conditions.
- Sustainability Practices: Developing methodologies that reduce environmental impact while optimizing thermal efficiency.
- Interdisciplinary Approaches: Encouraging collaboration among various fields to tackle complex thermal challenges effectively.
As we progress, the importance of thermal structures will only increase, necessitating ongoing exploration and innovation to meet contemporary and future demands.