Thermoelectric Coolers: Exploring Innovation and Impact
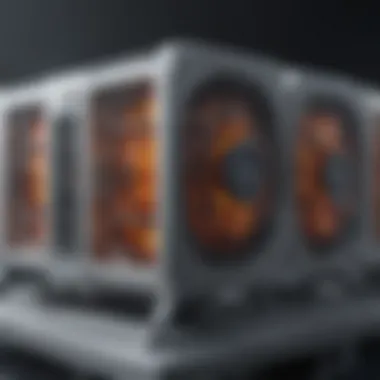
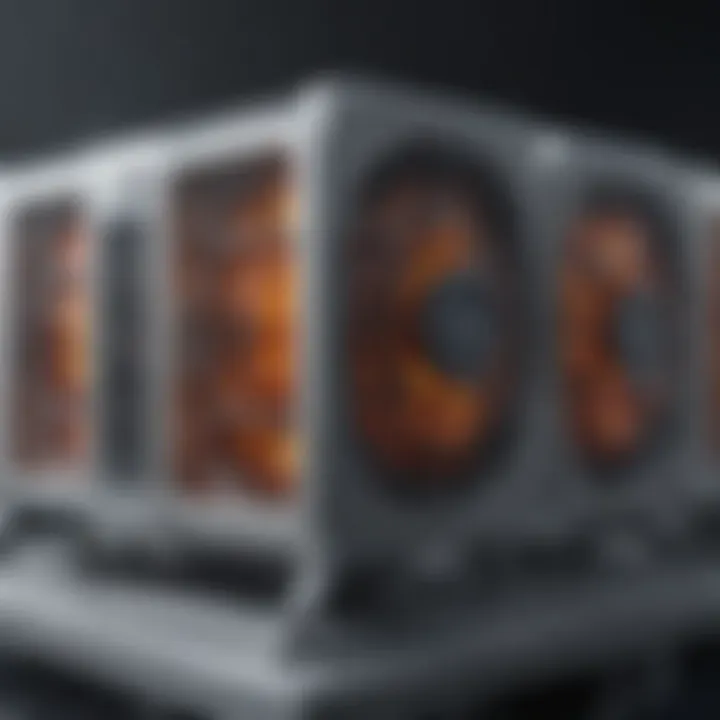
Research Background
Thermoelectric coolers (TECs) have garnered significant attention in recent years due to their unique ability to create temperature gradients using electrical energy. This technology addresses a pertinent scientific problem: the need for efficient and compact cooling solutions in various applications. With rising environmental concerns, conventional cooling methods relying on refrigerants or mechanical systems are often deemed inadequate. The thermoelectric effect, particularly the Peltier effect, forms the cornerstone of this technology, allowing heat to be moved from one side of a junction to another when electric current passes through it.
Historically, the concept of thermoelectricity emerged in the early 19th century. Scientists like Thomas Johann Seebeck and Jean Charles Athanase Peltier laid the groundwork for understanding thermoelectric phenomena. Early applications were limited; however, advancements in semiconductor materials in the latter half of the 20th century revitalized interest in this field. Studies have shown that the efficiency of thermoelectric materials significantly impacts the performance of TECs. Research into materials such as bismuth telluride and lead telluride has demonstrated their potential but also highlighted limitations in efficiency and cost.
Findings and Discussion
Recent advancements in thermoelectric coolers reveal several critical insights. One prominent finding is the improved efficiency of novel thermoelectric materials. For instance, materials with nanostructuring have been shown to enhance the thermoelectric figure of merit, a key parameter in evaluating performance.
Thermoelectric materials exhibit properties that determine how well they can convert heat into electrical energy and vice versa. The ongoing research emphasizes optimizing these materials to achieve better thermoelectric performance. Additionally, hybrid systems employing thermoelectric technology alongside traditional cooling methods present exciting opportunities for enhanced design effectiveness.
This synthesis of findings indicates a broader application range for thermoelectric coolers, particularly in fields such as refrigeration, automotive cooling, and electronics management. Each application comes with its unique set of challenges. In refrigeration, for example, power consumption and heat dissipation remain crucial concerns. In automotive applications, implementing thermoelectric coolers could improve the efficiency of waste heat recovery systems.
"Future research directions should focus on materials that are both cost-effective and capable of operating efficiently at varied temperatures."
The performance metrics for evaluating thermoelectric coolers include cooling capacity, efficiency, and heat transfer rates. Researchers continue to assess these factors in real-world applications to better understand their capabilities and limitations.
Prelude to Thermoelectric Coolers
Thermoelectric coolers place at the intersection of science and practical technology, aiming to offer an efficient solution for temperature control. This section sets the stage for understanding these devices. Its significance lies in their potential to revolutionize traditional cooling systems. Their solid-state nature, compact size, and ability to provide precise temperature management are appealing.
Advancements in materials and design have further enhanced their capabilities. Such progress leads to broader applications across various industries, from consumer electronics to automotive systems. Understanding their definition and functionality ensures professionals can leverage this technology effectively.
Definition and Functionality
Thermoelectric coolers (TECs) utilize the Peltier effect to create a heat flux between two different materials. When an electric current flows through the junction of these materials, one side cools down while the other side heats up. This principle allows for heat management without the moving parts common in mechanical systems, such as compressors.
TECs serve dual functions: cooling and heating. In cooling mode, they can be employed to lower the temperature of a device or environment. In heating mode, they can maintain warmth. This versatility is one of their major advantages. Their operation is efficient in controlled environments such as laboratory settings and sensitive electronics where excessive heat can lead to failure.
Historical Context
Thermoelectric technology is not new; its roots extend back to the early 19th century. It began with the discovery of the Seebeck effect in 1821, followed by the Peltier effect later in 1834. Initially, these effects were theoretical curiosities, limited to laboratory settings.
As technology advanced, material science evolved. The advent of bismuth telluride in the mid-20th century marked a turning point. It provided a practical material suitable for commercial applications of thermoelectric coolers. Over the decades, interest increased, paralleling global demands for energy-efficient technologies. As environmental concerns grew, so too did investments into clean cooling technologies.
Thermoelectric coolers bridge the gap between traditional cooling technologies and modern energy-efficient solutions.
Today, they are integral in a variety of high-tech applications. From medical devices to environmental monitoring systems, the relevance of thermoelectric coolers continues to expand.
Principles of Thermoelectric Cooling
Understanding the principles behind thermoelectric cooling is crucial for comprehending the functionality and efficiency of thermoelectric coolers. This section elucidates some fundamental thermoelectric effects that underpin these devices. By grasping these principles, students, researchers, and professionals can appreciate the technological innovations and limitations of thermoelectric coolers. Notably, the Seebeck, Peltier, and Thomson effects provide insights into how thermoelectric materials can harness and manipulate heat energy.
The Seebeck Effect
The Seebeck effect describes the generation of an electric voltage when there is a temperature difference across two different conductive materials. When one end of a thermoelectric material is heated while the other remains cooler, charge carriers (either electrons or holes) migrate from the hot side to the cold side. This movement results in a voltage that can be harnessed for power generation.
In practical applications, the Seebeck effect is significant for creating thermoelectric generators. It enables the conversion of waste heat into electricity, a valuable capability for improving energy efficiency across various industries. Some common applications include powering sensors in remote locations or in automotive systems where waste heat is abundant.
The Peltier Effect
Contrasting the Seebeck effect, the Peltier effect is the principle behind the cooling function of thermoelectric coolers. When an electrical current flows through two dissimilar conductors, heat is either absorbed or released at the junctions where they meet. If one junction absorbs heat, it operates as a cooling device. This effect is utilized in devices that provide precise temperature control such as portable coolers and refrigeration systems.
Essentially, the efficiency of the Peltier effect is determined by the thermoelectric materials used and their specific properties. Hence, understanding this effect is critical for optimizing the application of thermoelectric coolers in various fields, including consumer electronics and automotive cooling.
The Thomson Effect
The Thomson effect gives insight into the relationship between heat and electrical current flowing through a conductive material. It describes how, when an electric current passes through a conductor with a temperature gradient, heat is either absorbed or released depending on the direction of the current. In simple terms, this means that if the current flows from a hot region to a cold one, heat is released, and vice versa if the current travels in the opposite direction.
Although this effect is less commonly discussed compared to the Seebeck and Peltier effects, it still plays a role in fine-tuning the performance of thermoelectric devices. Understanding the Thomson effect allows engineers to optimize designs for better thermal management and energy use, further enhancing the technology's application in thermoelectric cooling solutions.
Materials for Thermoelectric Coolers
The choice of materials is fundamental in determining the efficiency and performance of thermoelectric coolers. The materials used influence not only the thermoelectric effects but also the overall design and application of these devices. Effective cooling relies on maximizing the thermoelectric performance characteristics. This section explores various materials and their unique properties that affect the performance of thermoelectric coolers.
Types of Thermoelectric Materials
Bismuth Telluride
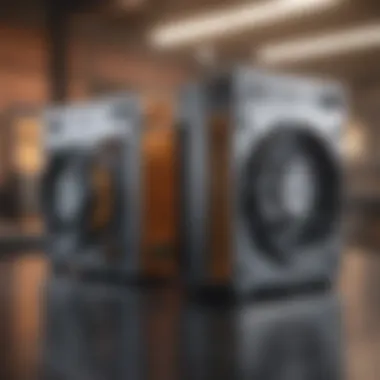
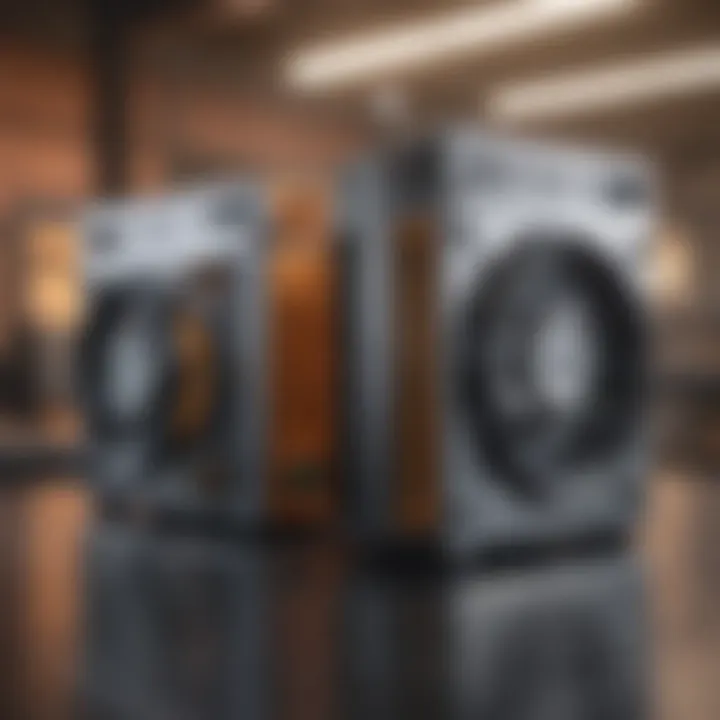
Bismuth Telluride is perhaps the most widely used thermoelectric material. Its effectiveness near room temperature makes it ideal for various applications, including electronics cooling and portable refrigeration. The key characteristic of Bismuth Telluride is its high efficiency as a thermoelectric material, which is critical when considering power consumption in devices. Its unique feature lies in its ability to function in both P-type and N-type forms, providing great versatility.
However, there are disadvantages. The performance drops significantly at higher temperatures, limiting its application in high-heat environments. The material also tends to be expensive, impacting overall system costs.
Lead Telluride
Lead Telluride stands out in high-temperature applications. It is particularly effective in situations that demand robustness. The key characteristic of Lead Telluride is its high thermoelectric performance at elevated temperatures, often exceeding the capabilities of Bismuth Telluride. Furthermore, it exhibits advantageous mechanical properties, which allow for easier handling during manufacturing.
The unique feature of this material is its extended operational stability at high temperatures. Nevertheless, Lead Telluride also comes with disadvantages, such as potential toxicity and environmental concerns associated with lead, which complicate its usage and disposal.
Silicon-Germanium Alloys
Silicon-Germanium alloys are known for their excellent performance in high-temperature applications, particularly for space and aerospace usage. The key characteristic of Silicon-Germanium is its good balance between electrical properties and thermal performance. It generally has a lower cost compared to other high-performance thermoelectric materials, which makes it appealing for large-scale applications.
The unique feature of Silicon-Germanium alloys is their capability to operate effectively in harsh environments. However, their performance can be less effective at lower temperatures compared to materials like Bismuth Telluride, presenting a limitation in diverse operational ranges.
Material Properties Influencing Performance
Electrical Conductivity
Electrical conductivity plays a crucial role in the performance of thermoelectric coolers. It directly impacts how effectively the material can transport electric current. Higher electrical conductivity can lead to lower resistive losses in thermoelectric devices. This characteristic is particularly valuable in applications demanding efficiency and longevity.
However, high electrical conductivity must be balanced with lower thermal conductivity. This trade-off is essential, as materials must maintain a compact thermal gradient to achieve effective cooling.
Thermal Conductivity
Thermal conductivity is another vital property that significantly affects the performance metrics of thermoelectric coolers. Low thermal conductivity in a thermoelectric material is desirable to create a temperature gradient. This property allows more efficient cooling and heating by limiting heat flow from hot to cold areas.
On the contrary, materials with high thermal conductivity can reduce the effectiveness of the thermoelectric module, leading to wasted energy and diminished performance. Therefore, selecting materials with the right balance of thermal conductivity is key in design.
Seebeck Coefficient
The Seebeck coefficient measures the voltage generated in response to a temperature difference across a material. A high Seebeck coefficient is essential for enhancing the thermoelectric efficiency of a cooler. This property defines how effectively a material converts temperature differences into electric voltage, which is critical for thermoelectric function.
Yet, achieving a high Seebeck coefficient can be challenging. This is often accompanied by lower electrical conductivity, which complicates the design of efficient thermoelectric systems. Understanding these interconnected properties is crucial in advancing the performance of thermoelectric coolers.
Design and Construction of Thermoelectric Coolers
The design and construction of thermoelectric coolers (TECs) is essential for understanding how these systems operate efficiently. These devices convert electrical energy into temperature differences with minimal moving parts. This solid-state nature results in a range of design considerations that can impact performance, reliability, and longevity. Effective design choices can lead to higher efficiency and lower costs, making the study of this topic crucial for researchers and engineers alike.
Module Configuration
Series Configuration
In a series configuration, multiple thermoelectric modules are connected end-to-end, enabling the cumulative effect of cooling across the units. The main characteristic of this setup is the increase in voltage and cooling capacity while keeping the current flow consistent. One significant benefit of series configuration is its scalability; for more substantial cooling needs, additional modules can simply be added without redesigning the entire unit.
The unique feature of series configurations is that they often manage to achieve a high temperature differential across the cooler. This can result in a more substantial cooling effect in specific applications such as industrial chillers or high-performance electronics. However, an important disadvantage is that if one module fails, the entire system may cease to function, making reliability a crucial consideration.
Parallel Configuration
In contrast, a parallel configuration involves connecting several thermoelectric modules such that they share the same voltage. The primary characteristic of parallel setups is that they can maintain cooling effectiveness even if one or more modules fail, improving reliability. This modular approach can be particularly advantageous in applications requiring continuous operation, such as refrigerators and other household appliances.
The unique feature of parallel configuration is its ability to distribute heat load evenly across the modules. This setup results in a smoother performance under variable thermal loads and is often seen as a more robust choice for applications in consumer electronics. However, the major drawback is lower overall cooling capacity compared to series configurations, which may be limiting in specific high-demand scenarios.
Heat Sink Design
Heat sink design plays a pivotal role in thermoelectric cooler performance. A heat sink's primary function is to dissipate the heat generated by the thermoelectric modules effectively. The material and geometry of the heat sink must be chosen carefully to ensure optimal thermal conductivity and surface area. Common materials used include aluminum and copper, each offering unique advantages in terms of weight and heat dissipation capabilities.
Key aspects of heat sink design include:
- Size: Larger heat sinks provide more surface area for heat dissipation but can increase the system's overall size and weight.
- Shape: Fins or other geometries can enhance surface area and improve airflow around the heat sink, increasing efficiency.
- Thermal Interface Material: Proper thermal interface materials enhance the contact between the heat sink and the thermoelectric module, further improving thermal conductivity.
Performance Metrics for Thermoelectric Coolers
Understanding the performance metrics of thermoelectric coolers is crucial for evaluating their effectiveness and efficiency. These metrics help quantify how well a thermoelectric cooler operates and guide improvements in materials and designs. Using defined metrics can help researchers and engineers assess the viability of these systems in various applications. The primary performance metrics include the coefficient of performance, thermal resistance, and cooling capacity.
Coefficient of Performance
The Coefficient of Performance (COP) measures the efficiency of a thermoelectric cooler. It is defined as the ratio of the heat removed from the cold side to the electrical power input. A higher COP indicates a more efficient cooling process, which is essential in applications where energy consumption is a primary concern.
The formula for COP is:
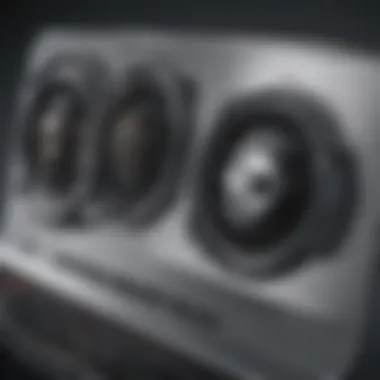
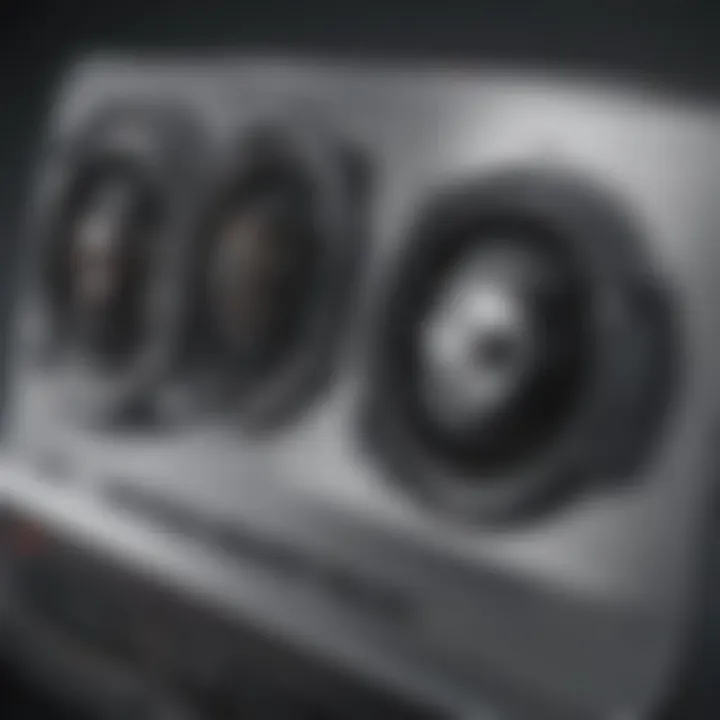
Where
- ( Q_c ) = heat extracted from the cold side
- ( W ) = electrical work input
A high COP reduces operational costs and extends the lifespan of systems relying on thermoelectric cooling. By focusing on improving the COP, researchers can enhance the overall appeal of this technology.
Thermal Resistance
Thermal resistance is another vital performance metric for thermoelectric coolers. It describes the ability of a cooler to resist heat flow from the hot side to the cold side. Low thermal resistance is desired to maximize cooling efficiency.
In practical terms, a thermoelectric cooler with high thermal resistance will struggle to maintain the desired temperature difference. As such, understanding and optimizing thermal resistance enhances the performance of cooling systems, especially in sensitive applications.
Factors influencing thermal resistance include:
- Material properties: Different materials exhibit varying conductivity.
- Physical configuration: The arrangement of the thermoelectric elements impacts performance.
- Heat sink design: Effective heat management optimizes thermal pathways, lowering resistance.
Cooling Capacity
Cooling capacity measures the maximum heat load a thermoelectric cooler can remove from an object or environment. This metric is essential in determining the suitability of a cooler for specific applications, whether in consumer electronics or industrial systems.
Cooling capacity depends on several factors, including:
- Temperature gradient: The greater the temperature difference between the hot and cold sides, the better the cooling performance.
- Material efficiency: The effectiveness of the thermoelectric materials can significantly influence cooling capacity.
- Module size: Larger modules can generally transfer more heat but may also require more power.
A clear understanding of cooling capacity enables designers to align thermoelectric coolers with the requirements of various uses effectively.
In summary, assessing the performance metrics of thermoelectric coolers is critical. Performance metrics—COP, thermal resistance, and cooling capacity—provide insights into the viability of these systems, guiding advancements in this technology and ensuring its longevity in various applications.
Applications of Thermoelectric Coolers
The exploration of thermoelectric coolers (TECs) is incomplete without discussing their applications. TECs hold significant potential across various industries due to their unique capabilities. They provide specific benefits that other cooling methods struggle to offer, such as solid-state operation and compact design. This makes them valuable in environments where space and reliability are crucial.
TECs are utilized in numerous fields, each benefiting from the inherent advantages of thermoelectric technology. They find applications in consumer electronics, automotive systems, refrigeration, and medical devices, transforming conventional cooling methods.
Consumer Electronics
In the realm of consumer electronics, thermoelectric coolers have found their place in devices that require precise temperature control. Products like portable coolers, mini refrigerators, and even some gaming consoles use TECs for efficient cooling. The ability to directly control temperature without moving parts leads to increased durability and longer lifespans for these devices.
There are also advantages in terms of energy efficiency. For instance, laptops and high-performance computing systems use TECs to manage heat generated by processors. As a result, improved performance is achieved without significant energy consumption. This feature is important when considering the growing demand for sustainable technologies.
Automotive Cooling
In the automotive industry, thermoelectric coolers are increasingly employed to improve cooling systems. They can manage the temperature of critical components such as batteries in electric vehicles. As electric cars gain popularity, the need for reliable and compact cooling solutions intensifies.
TECs also serve to enhance passenger comfort. Some models utilize them for seat cooling, offering a consistent and controlled climate within the vehicle. This approach can lead to energy savings, as it reduces the reliance on traditional air conditioning units, which often consume more power.
Refrigeration Systems
Refrigeration systems present a promising application area for thermoelectric coolers. They can effectively replace conventional compressor-based systems in specific contexts. For instance, small scale refrigeration units benefit from TECs due to their compact size and lack of moving parts. Furthermore, they are quieter, an essential factor in residential setups.
Their versatility extends to specialized refrigeration applications, such as in pharmaceutical storage. In these settings, maintaining consistent temperatures is crucial. Thermoelectric cooling offers reliable performance without risk of chemical leaks or mechanical failures that could arise from traditional cooling systems.
Medical Applications
In the medical field, thermoelectric coolers play a critical role in certain technologies. They are used for precise temperature management in devices like portable blood storage units and diagnostic equipment. Given the sensitivity of biological samples, the ability to maintain a stable environment is vital.
Moreover, TECs can be integrated into wearable devices for health monitoring. These devices benefit from the compact and lightweight nature of thermoelectric coolers, allowing for in-the-field use without significant weight burden on the user. This demonstrates the adaptability of TECs in developing efficient medical technologies.
"The applicability of thermoelectric coolers spans numerous sectors, proving their potential as viable alternatives to traditional cooling systems."
TECs demonstrate significant promise across various platforms, helping to bridge the gap between efficiency and functionality. The different applications outlined above highlight how thermoelectric cooling is shaping industries, paving the way for advancements that prioritize performance and sustainability.
Advantages and Limitations of Thermoelectric Coolers
The section on the advantages and limitations of thermoelectric coolers plays a crucial role in understanding their effectiveness and applicability across various fields. Identifying both the benefits and challenges associated with these cooling devices provides valuable information for researchers and practitioners considering their use.
Benefits of Thermoelectric Cooling
Solid-State Operation
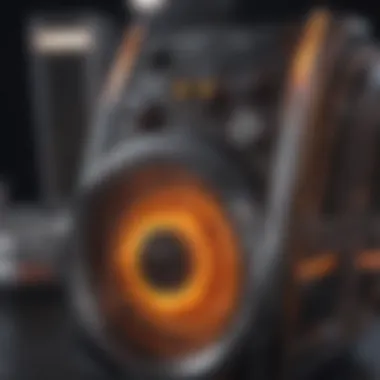
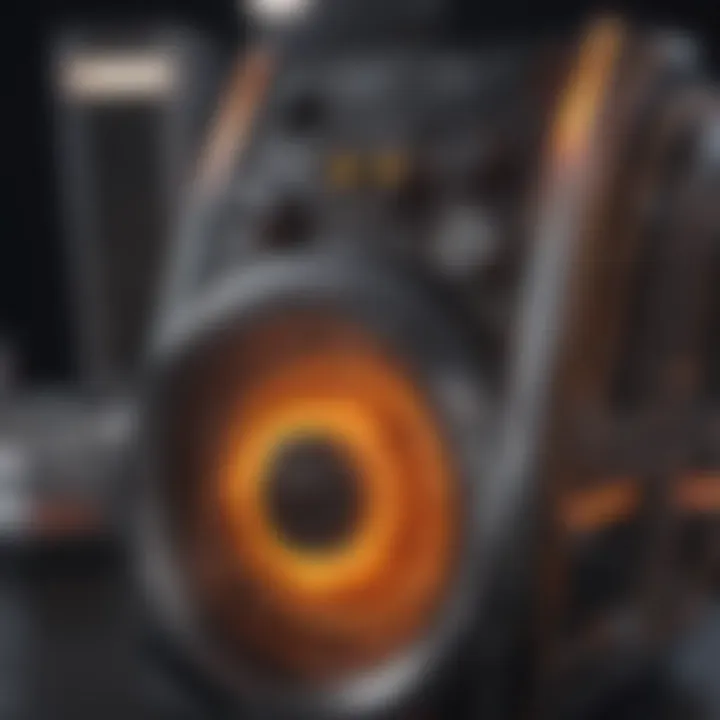
Solid-state operation is one of the hallmark features of thermoelectric coolers. This characteristic contributes significantly to their appeal in modern technology. Unlike traditional refrigeration systems that utilize moving parts or refrigerants, thermoelectric coolers operate on the principles of thermoelectricity and have no moving components. This leads to increased durability and reduced maintenance needs.
Another key aspect of solid-state operation is energy efficiency. Thermoelectric coolers can achieve a significant degree of control over temperature without the complexities often associated with mechanical systems. The absence of refrigerants also aligns with environmental sustainability goals.
However, a notable disadvantage is that while solid-state devices can be extremely reliable, they may not deliver the same cooling power as conventional systems in larger applications.
Compact Design
Compact design is another significant aspect related to thermoelectric coolers. Their small size allows them to fit into a variety of applications, from portable coolers to integrated systems in electronics and automotive applications. A compact design simplifies installation, making these systems suitable for both small-scale uses and larger, more complex applications.
This feature is beneficial as it enables innovation across sectors. For instance, in consumer electronics, the thin profile of thermoelectric coolers can integrate seamlessly with devices requiring efficient thermal management. Despite the advantages, the compact nature may sometimes limit the cooling capacity available for larger tasks, necessitating careful consideration regarding the requirements.
Challenges in Performance
Efficiency Limitations
Efficiency limitations are among the key challenges faced by thermoelectric coolers. The performance of these coolers, often measured through their coefficient of performance, tends to be lower than that of traditional cooling methods. This limitation is particularly pronounced in environments requiring significant cooling power or large temperature differentials.
Understanding efficiency is essential for applications where energy consumption is a critical factor. Users need to assess whether the lower efficiency can be justified by the benefits provided, such as solid-state operation or a smaller size. A further complication arises from temperature variations because their cooling effectiveness declines as the temperature differential increases.
Cost of Materials
Cost of materials also represents a significant challenge in the adoption of thermoelectric coolers. The materials required for efficient thermoelectric performance, such as bismuth telluride and lead telluride, can be relatively expensive. This cost can hinder widespread implementation, particularly in less specialized applications where budget constraints are prevalent.
While advances in material science are gradually improving the cost-effectiveness of thermoelectric materials, it remains an important consideration. Balancing performance with budget constraints is crucial for manufacturers and end-users alike. If future research can reduce the costs associated with high-performance materials, it could lead to broader acceptance and utilization of thermoelectric technology.
"Understanding both the advantages and limitations of thermoelectric coolers is essential for informed decision-making regarding their application in varied domains."
Overall, evaluating both the benefits and limitations of thermoelectric coolers is imperative for harnessing their full potential. This balance of understanding enables strategic decisions, leading to more effective implementations in the quest for efficient cooling solutions.
Future Directions in Thermoelectric Technology
The exploration of thermoelectric technology has not reached its zenith; instead, it stands at the precipice of significant advancements. This section highlights what shapes the future of thermoelectric coolers, emphasizing both innovations in materials and the potential for energy harvesting. These elements are crucial because they can dramatically affect efficiency, application range, and overall impact on sustainable technologies.
Innovations in Materials
Current materials used in thermoelectric coolers have limitations, primarily in efficiency and cost. Enhancing the thermoelectric properties of materials could lead to substantial improvements in their performance. Bismuth telluride and lead telluride are common materials that exhibit reasonable performance ratings, but new discoveries are paving the way for more effective alternatives.
Ongoing research aims to identify novel materials that possess a higher figure of merit (ZT), which is a key indicator of thermoelectric efficiency. For example, nanostructured materials and organic compounds are gaining attention. These materials may exhibit better thermal and electrical conduction, aiding in the enhancement of energy conversion efficiency. Moreover, exploring 2D materials like graphene can provide unique properties advantageous for efficiency.
Using computational models and simulations can also assist researchers in predicting the effectiveness of various compounds before physical trials. Consequently, innovations in material design and synthesis techniques are critical for pushing thermoelectric technology forward.
Potential for Energy Harvesting
Beyond mere cooling applications, thermoelectric materials have the capability to harvest waste energy. This aspect presents a fascinating area of growth. The energy that escapes as heat in industrial processes, vehicles, or even household appliances can be converted into electricity through thermoelectric generators. Such capability holds the potential to revolutionize energy solutions by converting what is typically lost energy into usable power.
Implementing thermoelectric harvesting systems in practical scenarios can lead to energy efficiency improvements in multiple domains. For instance, automotive manufacturers can integrate these systems to power auxiliary functions, thereby reducing the total energy demand from the engine.
Energy harvesting not only complements the drive towards renewable sources but also aligns with the goals of minimizing environmental impact. The future of thermoelectric applications will stem largely from these developments, leading to new ways of integrating energy solutions that prioritize sustainability and efficiency.
"As we approach stricter regulations on energy consumption, the role of thermoelectric technology will only grow in importance."
Both of these directions highlight the potential held within thermoelectric technology. By improving materials and focusing on energy harvesting, there is a path forward that embraces innovation and sustainability. The results could be the key to making thermoelectric coolers viable as a major part of future energy solutions.
Ending
The conclusion section of an article serves as a pivotal component, summarizing the core discussions and insights generated throughout the text. In this case, the exploration of thermoelectric coolers has highlighted several crucial aspects that deserve emphasis. As thermoelectric coolers gain traction in various industries, understanding their function and potential is essential.
Thermoelectric coolers stand out primarily for their solid-state operation, which provides an advantage over traditional cooling technologies. This characteristic eliminates moving parts, leading to reduced maintenance and higher reliability. Additionally, their compact design makes them suitable for integration into various applications, from consumer electronics to automotive systems.
However, limitations exist, particularly regarding efficiency and material costs. Addressing these challenges is fundamental for the advancement of thermoelectric cooling technology. The conclusion not only distills the key points presented in the article but also underscores the ongoing importance of research and innovation in this field.
"The future of thermoelectric coolers lies in the continuous development of materials and designs that enhance efficiency while minimizing costs."
Key Takeaways
- Thermoelectric coolers offer a unique solution for cooling needs across multiple domains.
- Their solid-state operation results in fewer mechanical failures compared to traditional systems.
- Challenges surrounding efficiency and cost must be overcome for broader adoption.
- Future advancements may hinge on innovative materials and designs, making the field a promising area for research.
Implications for Future Research
The implications for future research in thermoelectric technology are notably significant. Continued innovation in materials science is essential for improving the efficiency of thermoelectric materials. Researchers must explore new compounds and synthesis methods that enhance the thermoelectric figure of merit.
Additionally, optimizing designs for specific applications could lead to tailored solutions that maximize performance. This involves not just material improvements but also a deep understanding of thermodynamics and heat transfer characteristics in varied contexts.
Significantly, the potential for energy harvesting using thermoelectric coolers represents an exciting frontier. As energy demands increase and sustainability becomes imperative, harnessing waste heat could provide an alternative source of power. Future studies should insightfully dwell on these prospects, examining both technical feasibility and economic justification.
By addressing these elements, the field of thermoelectric cooling can not only optimize current applications but also pave the way for new uses that leverage the unique features of thermoelectric technology.