Understanding Mass Spectrometer Resolution
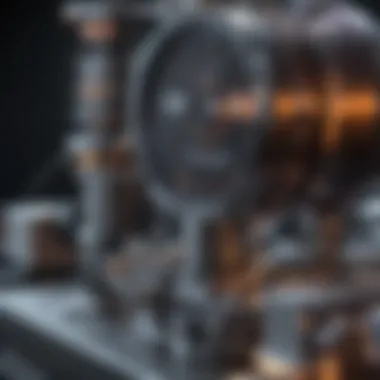
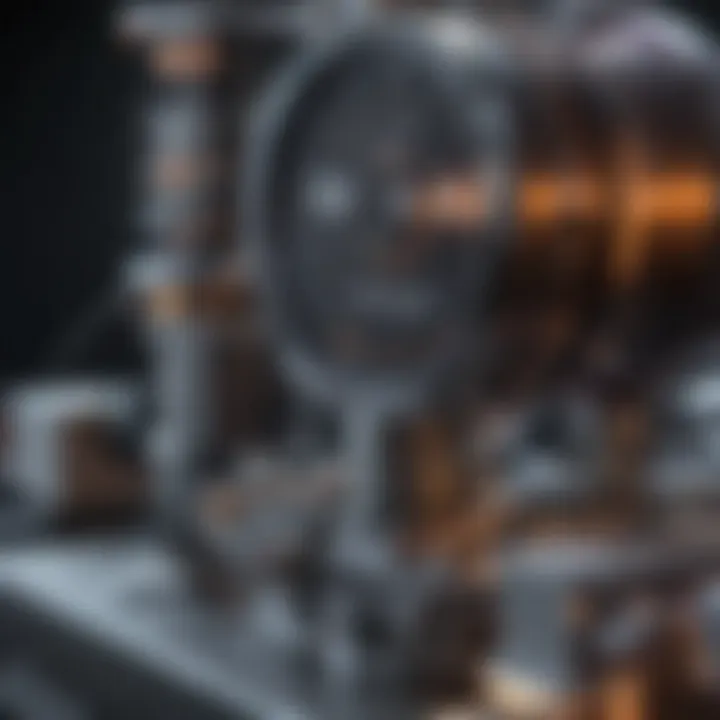
Research Background
Mass spectrometry, an indispensable tool in analytical chemistry, serves as a bridge to understanding the molecular and elemental makeup of substances. At its core, the process involves ionizing chemical compounds to generate charged molecules or molecule fragments and measuring their mass-to-charge ratios. This precision in identifying constituents is a focal point in many scientific fields, including pharmaceuticals, environmental science, and proteomics.
Overview of the Scientific Problem Addressed
Resolution within mass spectrometry refers to the ability to distinguish between two closely related mass peaks. High resolution allows scientists not just to detect, but to discriminate between substances that might otherwise look indistinguishable on a lower resolution instrument. Inaccuracies in resolution can lead to misidentification of compounds, which ultimately affects the quality of the data obtained in studiesāfrom environmental monitoring to drug development.
Historical Context and Previous Studies
The history of mass spectrometry dates back to the early 20th century. J.J. Thomsonās foundational work on the cathode ray tube laid the groundwork for later innovations in mass analysis. Since then, advancements such as the introduction of time-of-flight (TOF) and quadrapole mass spectrometers have progressively enhanced resolution capabilities. For instance, early devices struggled with overlapping mass peaks. Over time, the scientific community has sought to improve resolution through various means. Research papers from the 1990s onward frequently highlight the evolution of these methods, establishing a rich tapestry of knowledge that continually shapes current understanding.
Findings and Discussion
Exploring the mechanics of resolution reveals key components that directly influence performance. Among the factors affecting resolution are instrument design, ion optics, and the nature of the sample being analyzed. By refining these parameters, researchers can significantly improve the ability to resolve complex mixtures.
Key Results of the Research
Recent studies have shown significant strides in improving mass spectrometer resolution through enhanced detector technology and better ionization techniques. For instance, newer instruments can achieve resolutions exceeding 100,000 FWHM (full width at half maximum), allowing for precise differentiation between isobaric species (compounds with the same nominal mass).
Interpretation of the Findings
Understanding and interpreting these findings involves recognizing the practical implications they pose for research. High-resolution capabilities facilitate deeper insights into biological processes, environmental pollutant identification, and quantification of pharmaceutical agents. A robust resolution means confident results, paving the way for more reliable applications and discoveries across diverse domains.
"Indeed, the resolution of a mass spectrometer can be the difference between a breakthrough discovery and an unproductive detour in scientific research."
In the face of evolving challenges in mass spectrometry, attention to resolution matters more than ever. The push towards innovative methodologies reflects the broader trend towards precision in scientific analysis, ensuring that the tools of today can meet the demands of tomorrow's inquiries.
Prelude to Mass Spectrometry
Mass spectrometry has become a cornerstone in various scientific fields, underpinned by its ability to analyze and identify molecules with precision. Understanding the basic elements of mass spectrometry sets the stage for exploring the resolution of mass spectrometers, a critical attribute that greatly influences the quality and reliability of the results produced.
The resonance of mass spectrometry extends across disciplines such as biology, chemistry, and environmental science. It serves numerous purposes ranging from characterizing complex mixtures to establishing chemical structures and quantifying analytes. The importance of grasping how mass spectrometry functions lies not just in its application but also in appreciating the sophisticated mechanisms that ensure its effectiveness. All this ultimately culminates in the pursuit of knowledge and innovation in a range of scientific endeavors.
Definition and Purpose
Mass spectrometry is defined as an analytical technique that measures the mass-to-charge ratio of ions. In simpler terms, it helps scientists determine the composition of a sample by converting it into ions and measuring how these ions behave in electric and magnetic fields. The primary purpose of mass spectrometry is to identify compounds within a sample and quantify them based on their mass, which translates into extensive applications, including drug development, environmental testing, and proteomic studies.
Essentially, mass spectrometry matches the physical properties of molecules against a known standard, effectively allowing for the detailed elucidation of molecular structures. Whether one is studying metabolites in a biological study or tracing pollutants in environmental samples, mass spectrometry has become an indispensable tool.
Historical Development
The roots of mass spectrometry can be traced back to the early 20th century. The journey began with J.J. Thomson's discovery of the electron in 1897, laying the groundwork for modern mass spectrometry. By 1918, F.W. Aston had developed the first mass spectrograph, which enabled precise measurements of atomic weights.
Over the decades, mass spectrometry has seen substantial technological advancements. During the mid-20th century, major strides were made with the advent of atmospheric pressure ionization techniques and the development of various mass analyzers such as the quadrupole and time-of-flight (TOF). Such innovations have paved the way for increasingly complex applications, making mass spectrometry a versatile and highly sought-after analytical tool in laboratories worldwide.
As science has evolved, so too has the need for more refined techniques. The historical context reveals that each technological leap brought new capabilities, thus shaping the trajectory of mass spectrometry into the sophisticated and broadly applicable instrument we rely on today. Understanding its history allows researchers to appreciate the depth of knowledge accumulated over the years and signals the continual evolution of this powerful analytical technique.
Fundamentals of Mass Spectrometer Resolution
The resolution of a mass spectrometer serves as a crucial element that ultimately determines the instrumentās performance and its applicability in various scientific disciplines. When we delve into the fundamentals of this resolution, we must recognize that a higher resolution facilitates more accurate and reliable results in analyses, making it an indispensable feature for researchers and analysts alike.
What is Resolution?
In the realm of mass spectrometry, resolution is defined as the ability of the instrument to distinguish between two closely spaced mass peaks. It defines how much detail can be discerned in an analysis. Essentially, resolution can be thought of as the sharpness or intimacy with which we can perceive the mass-to-charge (m/z) ratios of ions. A well-tuned mass spectrometer may achieve high resolution, allowing the differentiation of ions that differ by merely a few millidaltons.
Put simply, higher resolution means that two ions that are very similar in mass can still be detected and measured distinctly as separate entities rather than appearing as a single peak on a mass spectrum.
"Resolution differentiates the twins of the ion world, ensuring that their identities are intact in the chaotic dance of molecular analysis."
Importance of Resolution in Mass Spectrometry
The significance of resolution in mass spectrometry unfolds through several pivotal considerations:
- Precision in Identification: High resolution enables the separation of isomers or compounds with nearly identical m/z values, which is crucial for accurate identification in complex mixtures. For example, in proteomics, resolving peptides that differ by mere atomic mass units could be the difference between correct annotation and misidentification.
- Quantitative Analysis: Accurate quantification often depends on resolving peaks clearly. In metabolomics, for instance, the quantification of metabolites hinges upon distinguishing between overlapping signals; thus, inadequate resolution can skew results and lead to faulty biological interpretations.
- Improved Sensitivity: Higher resolution often correlates with improved sensitivity, allowing scientists to detect low-abundance compounds within a sample. When sensitivity and resolution go hand-in-hand, the effective limits of detection are significantly reduced.
- Advanced Applications: For tasks such as studying environmental samples or pharmaceutical products where complex matrices are common, high resolution is not just preferable, it is a potential game-changer. The ability to parse through a sample with a fine-tooth comb can reveal insights that less sophisticated techniques might miss.
Ultimately, the fundamentals of mass spectrometer resolution shape the quality of research outcomes and contribute deeply to the advancement of various scientific fields. Whether one aims to unravel the complexities of biological systems, dive into environmental analysis, or design novel materials, understanding resolution is foundational. In the coming sections, we will explore the array of factors that influence resolution and the tools to enhance it, thereby broadening our understanding even further.
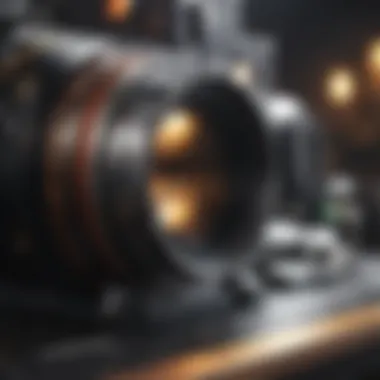
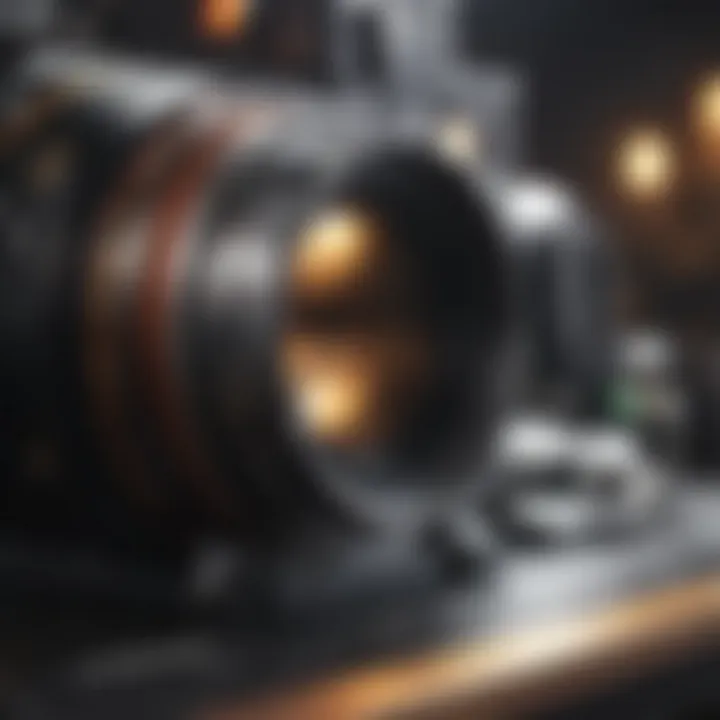
Factors Affecting Resolution
The resolution of a mass spectrometer is not just a simple figure; itās the very heart of the device's analytical power. Understanding the factors that influence resolution is crucial. Many researchers focus on accuracy and sensitivity, but overlooking resolution can lead to incomplete or inaccurate interpretations of mass spectral data. When discussing mass spectrometry, the term "resolution" generally refers to the ability of the instrument to distinguish between closely spaced mass-to-charge (m/z) ratios. Achieving enhanced resolution opens doors to identifying complex mixtures and enables more precise quantitation. It's an intricate dance of technology and methodology, where each component, from design to sample nature, plays its part.
Instruments and Design
The design of the mass spectrometer significantly influences its resolution. Different types of mass analyzers, such as quadrupole, time-of-flight, and orbitrap, come with varied abilities to separate ions based on their m/z ratios.
- Quadrupole analyzers offer a resolution that is more than adequate for many applications but can struggle with overlapping peaks, especially in complex samples. However, their faster scan speeds make them beneficial for targeted analyses.
- Time-of-Flight (TOF) analyzers shine when it comes to analyzing large biomolecules or complex mixtures. They can achieve high resolution by allowing ions to drift through a field-free region, providing a broader range for separation.
- Orbitrap analyzers, known for their exceptional resolution, employ electrostatic fields to trap ions and measure their m/z ratios with remarkable precision. The advanced design means they can achieve resolutions exceeding 1,000,000, a game-changer for many specialized applications.
The instrument layout, including the quality of vacuum systems and ion sources, also plays a pivotal role in maintaining resolution. A poor vacuum can lead to collisions and loss of ion integrity, thereby reducing resolution and impacting overall results.
Operating Conditions
Operating conditions essentially frame the environment in which your samples are analyzed. Factors such as pressure, temperature, and the type of chemical ionization method can alter ion behavior and affect how well the mass spectrometer retains resolution.
For instance, atmospheric pressure chemical ionization (APCI) might improve ionization for certain compounds but could also introduce variability in resolution if conditions arenāt well controlled. In contrast, electron impact ionization generally leads to stable conditions, but it may not be suitable for all analytes; thus, compromise can arise.
Moreover, settings such as voltage and timing of the ion pulses can steeply modulate the resolution. Even something as simple as the source temperature can dramatically affect how well the spectrometer functions and thereby impacts the clarity of the resulting data.
Sample Characteristics
The physical and chemical characteristics of the sample itself can make or break the resolution achieved by mass spectrometers. For example, sample purity can heavily influence results. Contaminants can lead to what we call spectral interference, where one compound masks another, especially if both have similar m/z ratios.
- Matrix effects are a considerable hurdle. When analyzing complex biological samples, the sample matrix can suppress or enhance signals of the analyte of interest, which can skew resolution.
- Volatility and polarity are two factors often overlooked. Samples that do not readily form ions or that fragment too easily can give a muddled picture in mass spectral analysis.
- Concentration levels also matter; too high or too low can lead to distorted outcomes, affecting resolution. A high concentration may cause ion suppression from too many competing signals, while a low concentration may not generate a strong enough signal to be distinguishable.
In sum, the resolution achieved in mass spectrometry is a rich tapestry woven from instruments and designs, operating conditions, and sample characteristics. Each thread contributes to the overall picture, illustrating the intricacies of mass spectrometry as a powerful analytical tool that continues to evolve.
Techniques to Enhance Resolution
Optimizing the resolution of a mass spectrometer is crucial for achieving accurate and reliable results in analytical chemistry. Techniques that enhance resolution not only improve measurement precision but also broaden the range of applications for mass spectrometry. By employing these techniques, researchers can discern subtle differences in compound structures and concentrations, enabling deeper insights into complex samples. In this section, we explore several key methodologies that bolster the performance of mass spectrometers and their significance in various fields.
Mass Analyzers
Quadrupole
Within the realm of mass spectrometry, the quadrupole mass analyzer stands out for its effectiveness. It works by using oscillating electric fields to filter ions according to their mass-to-charge ratio. A notable characteristic of the quadrupole is its ability to perform fast scans, making it a popular choice in various applications such as targeted analysis and multiple reaction monitoring. One unique feature here is the ease of integration with other instruments, such as liquid chromatography, which enhances overall system performance.
However, while quadrupoles are known for their rapid analyses and robust designs, they might have limitations in resolution when compared to high-end alternatives. They can resolve ions efficiently, but for complex mixtures or isobaric compounds, their power can wane. So, while they have undeniable advantages, considering their limitations is important for selecting the right tool for the job.
Time-of-Flight
The time-of-flight (TOF) mass analyzer is another key player in enhancing mass spectrometer resolution. This technology measures the time it takes for ions to traverse a fixed distance, allowing for high-resolution measurements. A standout feature of TOF is its exceptional ability to provide significantly high-resolution results for a wide range of mass ranges in rapid succession. This is particularly useful in proteomics and metabolomics, where understanding complex mixtures is essential.
But, that exhilaration comes with a catch. TOF instruments tend to be more sensitive to fluctuations in ionization conditions, which can sometimes lead to skewed data if not properly controlled. Despite that, its broad applicability shines through, making it a go-to option in many advanced analytical settings.
Orbitrap
The Orbitrap mass spectrometer rises above others for its unmatched resolution capabilities. Operating on the principle of trapping ions in an electrostatic field, it analyzes their oscillatory motion to retrieve mass data with extraordinary precision. An alluring aspect of Orbitrap technology is its capability to achieve extremely high mass resolution, often exceeding 1 million, making it a sought-after tool in high-end applications like metabolomics and environmental studies.
Yet, along with its strengths, the Orbitrap also presents a few challenges. Its complexity and cost can be prohibitive, particularly for smaller labs or institutions. Moreover, the required vacuum conditions and maintenance can contribute to higher overall operational costs. Nevertheless, for researchers who need to push the boundaries of analysis, investing in an Orbitrap can yield significant returns.
Ion Manipulation
In addition to mass analyzers, ion manipulation techniques can significantly enhance resolution. These methods encompass a range of strategies for controlling ion behaviors post-ionization. Techniques such as ion cooling, focusing, and trapping can lead to improved stability of ion signals. By refining these parameters, researchers can enhance the clarity of their mass spectra, thereby facilitating more accurate analysis.
Sample Preparation
Finally, sample preparation plays a pivotal role in achieving high resolution in mass spectrometry. Preparing samples meticulously ensures that the data collected reflects the true composition of the analyte. The purity, concentration, and form of the sample all influence ionization efficiency and detector response. Techniques such as solid-phase extraction or liquid-liquid extraction can concentrate analytes and remove potential interferents. This careful preparatory work lays the groundwork for obtaining cleaner and higher quality spectra, ultimately guiding to better interpretation of results.
"Achieving optimal resolution in mass spectrometry requires a fine-tuned balance of technological and preparatory techniques."
Applications of High Resolution in Mass Spectrometry
High resolution in mass spectrometry isnāt just a fancy term tossed around in labs; itās a fundamental component that can make or break an experiment. Essentially, it dictates how well one can differentiate between two closely related compounds. This differentiation is crucial, particularly in intricate fields such as proteomics, metabolomics, and environmental studies.
Having a high-resolution mass spectrometer (HRMS) improves the ability to accurately identify and quantify a broad array of substances, from protein fragments to complex biodiesel mixtures. The implications are enormous, extending from basic research to clinical diagnostics and environmental monitoring.
When an instruments resolution is high, it captures even the faintest distinctions. This means, for example, that minute variations in molecular weight or structure are not lost in the mix.
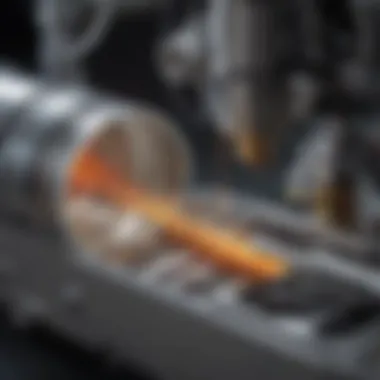
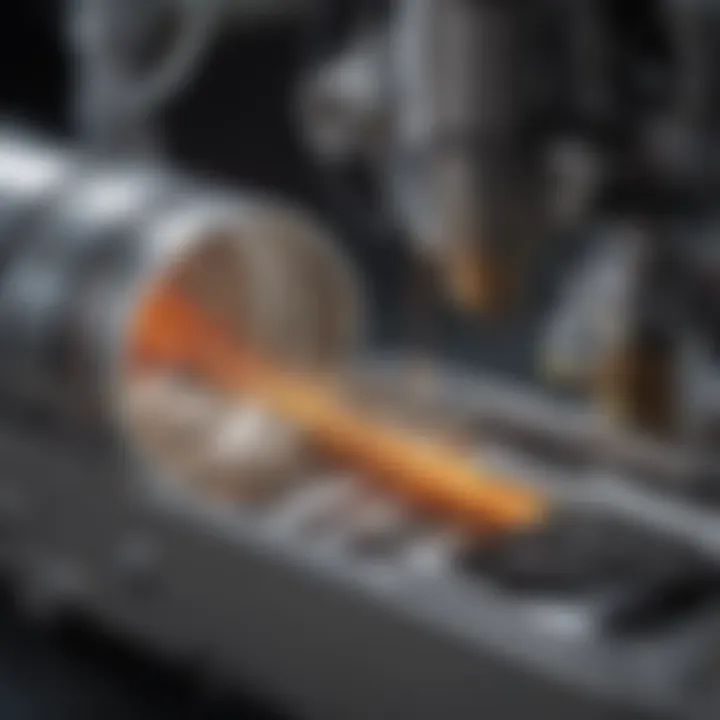
High resolution can allow for clear identification of compounds that are structurally similar, which might otherwise confuse results in traditional mass spectrometers.
This clarity is what enables researchers and professionals alike to glean meaningful insights from their analyses, paving the way for advancements in their respective fields.
Proteomics
In proteomics, the study of proteins and their functions, high resolution is vital due to the complex and highly variable nature of protein structures. Each protein can possess numerous isoforms, which may only differ by a single proton or minor modifications. Therefore, being able to distinguish between these subtle differences is crucial for accurate identification and quantification.
Moreover, with high-resolution techniques, researchers can map entire protein interactions and networks, providing insights that inform drug discovery and treatment strategies in medicine.
- Key Benefits:
- Distinguishes between closely related protein variants
- Enables accurate quantification of proteins
- Facilitates the identification of post-translational modifications.
Metabolomics
Metabolomics focuses on the comprehensive study of metabolites within an organism. Again, the nature of metabolites is often intricate. Many metabolites can have very similar masses, making resolution imperative to their analysis.
By employing high-resolution mass spectrometry, scientists can uncover biomarkers related to various diseases, evaluate metabolic pathways, and even examine the effects of drugs on metabolism. The precision involved in this analysis can steer clinical applications to new heights.
- Considerations:
- Detection of low-abundance metabolites
- Comprehensive data set generation from biological samples
- Insight into dynamic metabolic processes.
Environmental Studies
In environmental research, especially regarding pollution and complex chemical mixtures, high resolution plays a crucial role. Pollutants often comprise numerous substances that vary even slightly in composition. The resolution of the mass spectrometer determines how effectively these compounds can be separated and measured, informing environmental impact assessments and regulatory compliance.
Using high-resolution techniques, researchers can achieve precise quantification of pollutants in water, soil, and air samples.
- Benefits in Environmental Contexts:
- Detailed profiling of contaminants
- Enhanced understanding of chemical interactions
- Better risk assessment models for public health.
In summary, high resolution is much more than a technical specification; itās a decisive factor that drastically enhances the functional capabilities of mass spectrometry. With ongoing developments, these applications in proteomics, metabolomics, and environmental studies are just the tip of the iceberg.
Comparative Analysis of Mass Spectrometry Techniques
In the realm of mass spectrometry, comparative analysis serves as the bedrock upon which researchers assess the efficacy and precision of various methodologies. Each technique brings a unique set of capabilities that can influence the reliability of results across different applications. Understanding these differences is crucial not only for optimizing analytical methods but also for tailored solutions to specific research queries. The resolution capabilities that each methodology offers could very well be the difference between capturing a subtle signal amid a cacophony of background noise or overlooking important analytical insights altogether.
Resolution Capabilities of Different Methodologies
A key aspect that distinguishes mass spectrometry techniques is their resolution capabilities. The resolution of a mass spectrometer dictates how well it can differentiate between ions of slightly different masses. Here are some of the notable methodologies:
- Quadrupole Mass Spectrometry: This technique typically offers moderate resolution. Its three-dimensional configuration allows for selective filtering of ions based on their mass-to-charge ratio. Itās widely used in routine analysis but can struggle with resolving isobaric compounds effectively.
- Time-of-Flight (ToF) Mass Spectrometry: ToF technology can achieve high resolution, operating on the principle that ions of different masses will travel at different speeds through a vacuum. This makes it particularly effective for analyzing complex mixtures where precise mass measurements matter.
- Orbitrap Mass Spectrometry: Known for its exceptional resolution, the Orbitrap can differentiate ions separated by mere fractions of a mass unit. This high level of resolution facilitates detailed analysis of biomolecules, making it a favorite in proteomics.
The choice of methodology hinges not only on resolution capabilities but also the specific context of the analysis.
Pros and Cons of Each Technique
Understanding the advantages and disadvantages of each method can inform best practices in mass spectrometry. Hereās a breakdown:
Quadrupole Mass Spectrometry
- Pros:
- Cons:
- Cost-effective and widely available.
- Fast analysis times, ideal for high-throughput applications.
- Limited resolution for closely related compounds.
- Less effective in complex matrix analysis compared to other techniques.
Time-of-Flight (ToF) Mass Spectrometry
- Pros:
- Cons:
- High speed and exceptional resolution capabilities.
- Versatile, useful for both qualitative and quantitative analyses.
- More expensive and complex instrumentation.
- Requires careful calibration for precise measurements.
Orbitrap Mass Spectrometry
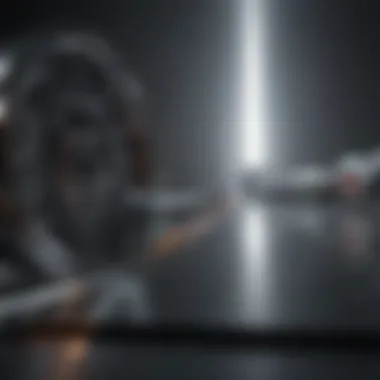
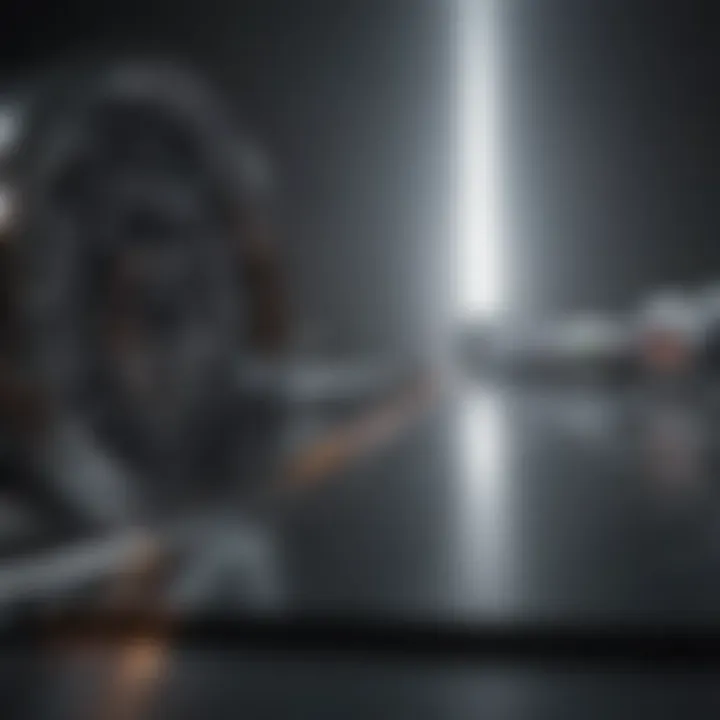
- Pros:
- Cons:
- Unmatched resolution ideal for detailed molecular characterization.
- Capability to analyze complex biological samples with minimal interference.
- High operational costs and maintenance requirements.
- Typically slower than other methods due to extensive data processing.
As a result, shifting between techniques depending on research stages or specific goals can ensure the robustness of analytical outcomes. Depending on the context, researchers might find themselves toggling between methods to optimize resolution without sacrificing time or budget.
"The choice of mass spectrometry technique is not merely an option; it's a strategic decision that can define the quality and reliability of scientific outcomes."
In summary, a thorough comparative analysis of mass spectrometry techniques enables the discerning researcher to navigate the complexities of analytical chemistry intelligently. With a clear understanding of the strengths and weaknesses present in each approach, scholars can better equip themselves for challenges inherent in the pursuit of knowledge.
Challenges in Achieving Optimal Resolution
When we talk about mass spectrometry, resolution is a core concept that directly influences the quality of data obtained. However, the journey to achieve optimal resolution is fraught with challenges that can hinder precise analysis. Addressing these hurdles is not just a matter of enhancing performance; itās about ensuring reliability and trust in the results. This part of the article sheds light on the key challenges in reaching peak resolution.
Technical Limitations
The landscape of mass spectrometry technology includes an array of sophisticated instruments, yet, despite their complexity, there are inherent technical limitations. One critical element is the design of the mass analyzers themselves. For instance, different types like Qudrupole, Time-of-Flight, or Orbitrap have distinct designs that govern their resolution. Some analyzers are inherently limited by their mass range, while others may face constraints with their ability to differentiate ions with very close mass-to-charge ratios.
Another trouble spot lies in the instrumental parameters, like voltage settings, pressure conditions, and ion source characteristics. An ideal scenario would pair optimal settings tailored to specific analytical tasks, but often itās a balancing act. In reality, finding this sweet spot where all parameters align for peak performance is easier said than done.
Moreover, the influence of noise and interference canāt be overlooked. Devices may pick up stray signals that not only cloud results but can skew data interpretation as well. So, even in the most high-tech setups, precision tends to be a moving target. The technological challenges of mass spectrometry require continuous innovation and rigourous testing to push the boundaries of resolution.
Practical Challenges in the Laboratory
While the technical factors are certainly significant, practical challenges in laboratory settings add another layer of complexity. A key aspect is the sample preparation process. The way a sample is prepared can drastically influence the signal quality. If not done meticulously, it can lead to contamination or loss of target analytes, both of which directly impact resolution.
Furthermore, maintaining consistent operating conditions is crucial. Variability in temperature, humidity, or pressure can lead to fluctuating results, making it difficult to achieve the desired resolution consistently. Laboratory personnel must adhere to strict protocols to ensure that these conditions remain stable.
Additionally, there is the unavoidable human element. The experts operating the mass spectrometers might sometimes face challenges, whether it's due to an often-too-tight schedule or simply the inherent difficulty of the analysis itself.
"The road to achieving optimal resolution in mass spectrometry is paved with both technical and practical hurdles, each requiring careful consideration and creative solutions to overcome."
Future Directions in Resolution Enhancement
Enhancing the resolution of mass spectrometers is a continually evolving endeavor in scientific research. This topic, therefore, is crucial to understand, as improvements in resolution can lead to breakthroughs in various applications such as proteomics, metabolomics, and environmental studies. The future holds promising prospects that directly correlate with enhanced resolution capabilities. By refining the technology that underpins mass spectrometry, researchers can glean richer, more accurate data from their analyses.
Emerging Technologies
The landscape of mass spectrometry is witnessing an influx of emerging technologies that promise to redefine the resolution capabilities of these instruments. One notable area of innovation is the development of next-gen mass analyzers like high-resolution quadrupoles and advanced time-of-flight systems. These tools are engineered to enhance the detection limit and sharpen resolution without sacrificing speed.
- Ultra-High-Resolution Mass Spectrometry (UHRMS): UHRMS takes conventional mass spectrometry up a notch. By minimizing the size of the ions during analysis, it increases the peak capacity. This allows for better separation of molecules that would otherwise be indistinguishable in traditional systems.
- Miniaturization: Another exciting development is the miniaturization of mass spectrometers. Smaller instruments can more easily adapt to fieldwork or be integrated into portable devices for on-site analyses. Compact designs can also reduce costs, making high-resolution analysis more accessible to a wider range of researchers.
- Hyphenation Techniques: Coupling mass spectrometry with other analytical methodsālike liquid chromatography or nuclear magnetic resonance (NMR)āimproves both capacity and resolution. Such hybrid techniques capitalize on the strengths of each method, yielding more comprehensive profiles of complex samples.
Predictive Modelling and Data Analysis
In the realm of mass spectrometry, the quantity of data produced can be overwhelming. This is where predictive modeling and advanced data analytics come into play. By harnessing machine learning algorithms, scientists can sift through large datasets to identify patterns that might not be immediately apparent. This approach can logically enhance the precision of resolution.
- Pattern Recognition: Machine learning models can be trained to recognize specific signatures of compounds. This capability aids researchers in distinguishing between ions that have similar mass-to-charge ratios, essentially enhancing resolution by facilitating a more nuanced analysis of complex mixtures.
- Prioritization of Analytes: Through predictive modeling, analysts can more effectively prioritize which analytes to target in an experiment. This not only streamlines research but also allows scientists to focus on compounds that matter most for their specific inquiries, reducing time and enhancing data relevance.
- Real-Time Processing: Implementing real-time data analysis using predictive algorithms means that researchers can adjust conditions as data comes in, improving the overall experiment outcomes and ensuring higher fidelity results.
"The integration of predictive modeling with mass spectrometry provides a powerful platform that enhances resolution capabilities, offering unprecedented insights in diverse fields of research."
The ability to foresee trends and behaviors in experimental outcomes can lead to new methods of analysis that were not previously possible. As both emerging technologies and data analytics proliferate, the future of resolution enhancement in mass spectrometry appears vibrant, with countless possibilities for advancing research and scientific understanding.
Ending
In wrapping up this exploration of mass spectrometer resolution, it's crucial to underscore how this intricately woven tapestry impacts scientific inquiry and discovery. The resolution of a mass spectrometer directly influences its ability to discern between molecules that might otherwise seem indistinguishable. In practical terms, this capability translates to insights not just in basic research, but also in applied science disciplines like pharmaceuticals, environmental science, and forensic analysis.
Summary of Key Points
To recap, several key themes have emerged throughout this article:
- Definition and Importance of Resolution: Resolution is fundamental in distinguishing ions of differing mass-to-charge ratios, a critical function in mass spectrometry.
- Factors Influencing Resolution: Instrument design, operating conditions, and sample characteristics all play pivotal roles in determining how well a mass spectrometer performs.
- Methods for Enhancing Resolution: The choice of mass analyzer and ion manipulation techniques can significantly improve resolution, which is vital for detailed qualitative and quantitative analysis.
- Real-World Applications: Areas such as proteomics and metabolomics demonstrate how improved resolution affects the understanding of biological processes and environmental monitoring.
- Future Directions: New technologies and improved data analysis methods will likely change the landscape of mass spectrometry.
In essence, the resolution of mass spectrometers forms the backbone of high-quality, reliable analytical data that researchers rely on. The more clarity these instruments can provide, the more insightful and transformative the research outcomes can be.
The Future of Mass Spectrometer Resolution
Looking ahead, the future of mass spectrometer resolution appears interwoven with advancements in technology. Emerging technologies promise to enhance resolution capabilities even further, facilitating the detection of lower concentrations of analytes.
For instance, developments in predictive modeling and machine learning are set to revolutionize data interpretation. By utilizing vast datasets, these technologies could potentially predict outcomes with unprecedented accuracy, leading to quicker and more reliable analyses. Alongside this, improvements in ionization techniques and materials may also reshape how samples are processed and analyzed.
The implications for diverse fieldsāranging from clinical diagnostics to environmental surveyingāare staggering. As methods evolve, the capacity for scientists to glean finer details from their analyses will expand, propelling innovations in research and application.
In summary, while the challenges in achieving optimal resolution persist, the trajectory of advancements indicates a future rich with possibilitiesāone where mass spectrometers will continue to be an invaluable tool in unlocking the secrets of matter.