Exploring the Role of sgRNA Expression Vectors in CRISPR
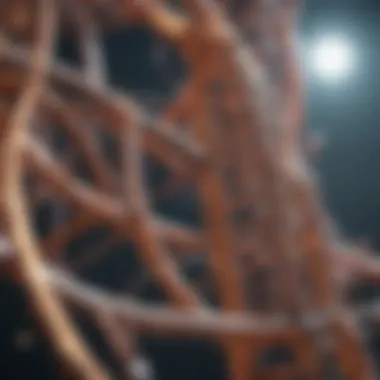
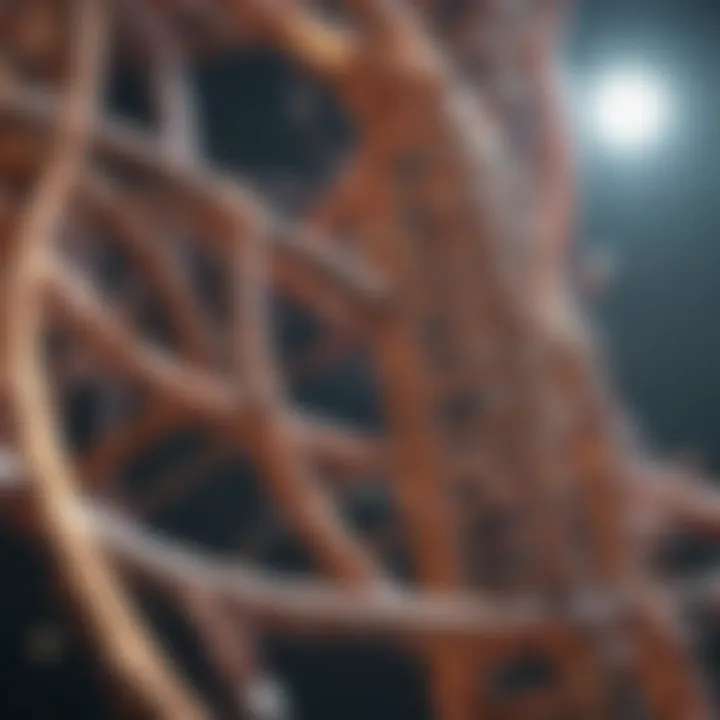
Intro
The introduction of CRISPR-Cas9 technology fundamentally changed the landscape of genetic engineering. At the heart of this advancement lies the significance of single guide RNA (sgRNA) expression vectors, which enable targeted genetic modifications with high precision. These vectors serve as essential tools in delivering synthetic sgRNAs into target cells, facilitating effective genome editing. Understanding their structure and function is crucial for both current research and future therapeutic applications.
Research Background
Overview of the scientific problem addressed
Genetic engineering has long been challenged by the difficulties in achieving specificity and efficiency in modifying genetic material. Traditional methods often relied on less precise techniques that could lead to unintended alterations in the genome. With the CRISPR technology, the focus shifted to a more refined approach, where sgRNAs guide the Cas9 nuclease to exact genomic locations. This advances the capability of precision genetics, requiring robust sgRNA expression vectors for successful implementation.
Historical context and previous studies
The development of sgRNA expression vectors can be traced back to the initial discoveries surrounding RNA-guided genome editing. Early studies, such as those conducted by Jinek et al. in 2012, laid the groundwork for understanding how sgRNAs interact with Cas9 to create double-strand breaks at specific sites in the DNA. Over time, various studies have explored the optimization of these vectors, including their design and delivery mechanisms. Recent advancements continue to expand our knowledge, addressing limitations in vector stability and efficiency in different host systems.
Findings and Discussion
Key results of the research
Several critical advancements have emerged in the field of sgRNA expression vectors. Research has indicated that different vector designs, such as those using plasmids or viral systems, can significantly influence the success of sgRNA delivery and retention in target cells. Studies emphasize the importance of selecting appropriate promoter elements that enhance expression levels.
Interpretation of the findings
The findings suggest that a thorough understanding of vector components is imperative for optimizing sgRNA delivery. Precision in genetic editing hinges on selecting suitable vector designs tailored to specific applications, whether for laboratory research or therapeutic interventions. As the field evolves, ongoing research efforts are directed toward refining these vectors, ensuring they meet the demands of complex experiments and clinical applications alike.
The End
The exploration of sgRNA expression vectors is vital in the quest for efficient and specific genetic engineering. By dissecting their structure, function, and the challenges within vector design and delivery, researchers can pave the way for innovative applications in both research settings and clinical environments.
Prelude to sgRNA Expression Vectors
The role of sgRNA expression vectors is essential in the landscape of genetic engineering, particularly within CRISPR technology. Understanding these vectors is paramount for anyone invested in genetics, biotechnology, and molecular biology. This section aims to provide a foundational overview of what sgRNA expression vectors are, their structure, and their significance in the context of genome editing. In the following paragraphs, key characteristics of sgRNA expression vectors, their applications, and their necessity in research and therapeutic settings will be discussed.
Definition and Importance
sgRNA expression vectors are plasmid DNA constructs that facilitate the production of synthetic guide RNAs (sgRNAs) needed for CRISPR-based genome editing. They contain gene sequences that encode sgRNAs, which direct the CRISPR-associated protein Cas9 to specific genomic targets. The ability to customize these vectors allows researchers to tailor the guide RNA to various genomic targets, thus enhancing the precision of genome manipulation.
This capability is critical for a range of applications, from basic biological research to therapeutic interventions. In the past, traditional genetic engineering methods could be time-consuming and less efficient. However, sgRNA expression vectors provide a more streamlined approach that significantly improves targeting efficiency. Their importance can be highlighted through various applications, such as gene knockouts, gene activation, or the correction of genetic defects, which may lead to potential cures for genetic disorders.
The Role of sgRNA in CRISPR Technology
The sgRNA serves as a crucial component of the CRISPR system. It is responsible for guiding the Cas9 protein to its target site within the genome. The interaction between the sgRNA, Cas9, and the target DNA is fundamental; the sgRNA must be complementary to the target sequence for successful editing. By introducing a precisely designed sgRNA into a cell, researchers enable the CRISPR machinery to locate and induce double-strand breaks in the DNA, allowing for various editing strategies.
It is also essential to note the versatility of sgRNA. The same sgRNA-based system can be adapted to different organisms, making it a powerful tool across various species and fields. This adaptability paves the way for advancements in functional genomics, where researchers investigate gene function and interactions on a broad scale.
"The advancement of sgRNA expression vectors represents a leap forward in genetic engineering, enabling unprecedented levels of precision in genome editing."
Components of sgRNA Expression Vectors
The components of sgRNA expression vectors are essential for their effectiveness in the CRISPR technology landscape. Each element plays a specific role, contributing to the overall functionality and efficiency of the sgRNA system. Understanding these components allows researchers to design vectors that optimize gene editing outcomes. Furthermore, the careful selection of these components impacts factors like transfection efficiency and specificity of the gene editing process.
Promoters and Regulatory Elements
Promoters are sequences of DNA that initiate the transcription of a particular gene. In the context of sgRNA expression vectors, they determine the rate and timing at which sgRNA is produced. The choice of promoter can significantly affect the expression levels of the sgRNA, thereby influencing the efficiency of genome editing.
Several types of promoters are commonly used, including constitutive promoters that drive constant expression, and inducible promoters that require specific conditions for activation. This option provides researchers with flexibility in modulating expression levels based on experimental needs.
Regulatory elements, such as enhancers or repressors, also play a critical role. They can interact with promoters to increase or decrease transcriptional activity. The right combination of promoters and regulatory sequences can enhance sgRNA production, making the vector more effective in targeting desired genes.
Selectable Markers
Selectable markers are genes introduced into sgRNA expression vectors that help identify cells that have successfully incorporated the vector. Their role is crucial in experiments where only a small percentage of cells may take up the sgRNA vector. Common selectable markers include antibiotic resistance genes, which allow for the selective survival of transformed cells when exposed to corresponding antibiotics.
Using these markers greatly streamlines the screening process. Researchers can easily differentiate cells with successful vector integration based on their ability to survive under selective conditions. This selection not only saves time but also increases the probability of obtaining high-quality, modified cell populations for further analysis.
Additionally, marker genes can contribute to the functionality of the vector. For example, fluorescent markers allow for real-time monitoring of transfected cells, providing insights into the effectiveness of the sgRNA in facilitating genome editing.
Cloning Sites
Cloning sites within sgRNA expression vectors are crucial for the insertion of the sgRNA sequence itself. These sites are often designed with specific restriction enzyme sites, which facilitate the straightforward integration of sgRNA sequences. The availability of multiple cloning sites allows for flexibility in vector design, making it easier to adapt the vector for specific research needs.
The efficiency of cloning also relates to how well the sgRNA sequence can be incorporated into the vector without disrupting essential vector functions. For this reason, attention must be given to the compatibility of the cloning method with the vector's intended use.
Researchers often employ methods such as restriction enzyme digestion followed by ligation or employ Gateway cloning, which utilizes recombination-based techniques for seamless integration of sgRNA sequences. These methods ensure that the integrity and function of the vector are preserved while achieving efficient cloning of the desired sgRNA.
Designing sgRNA Expression Vectors
Designing sgRNA expression vectors is a crucial aspect of genetic engineering and research, particularly in the CRISPR domain. These vectors serve as the vehicles for introducing synthetic guide RNAs into target cells, thus enabling precise modifications in the genome. The importance of this process cannot be underestimated, as it directly affects the efficiency and specificity of the genome editing. A well-designed vector will not only deliver the sgRNA effectively but also minimize potential side effects such as off-target effects.
The design process encompasses several elements, including target sequence selection and vector construction techniques. Each element contributes to creating an effective sgRNA expression vector capable of achieving the desired modifications with minimal complications. Key considerations involve the choice of vector backbone, regulatory elements, and the inclusion of selectable markers that can help in identifying successfully modified cells.
Target Sequence Selection
Target sequence selection is foundational for the effectiveness of sgRNA expression vectors. The identification of the correct genomic target is imperative, as the sgRNA must base-pair with the complementary DNA sequence to initiate the CRISPR/Cas9-mediated editing. Failure to select the appropriate target can lead to inefficiencies or unintended edits. Key factors to consider include the uniqueness of the target sequence within the genome and the presence of nearby protospacer adjacent motifs (PAMs), which are essential for Cas9 binding.
Furthermore, bioinformatics tools can assist in predicting off-target sites and assessing the potential impact of the chosen sequence. An optimal target sequence will balance specificity and effectiveness, ensuring that modifications are made efficiently and accurately.
Vector Construction Techniques
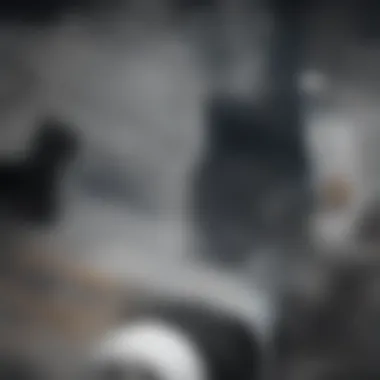
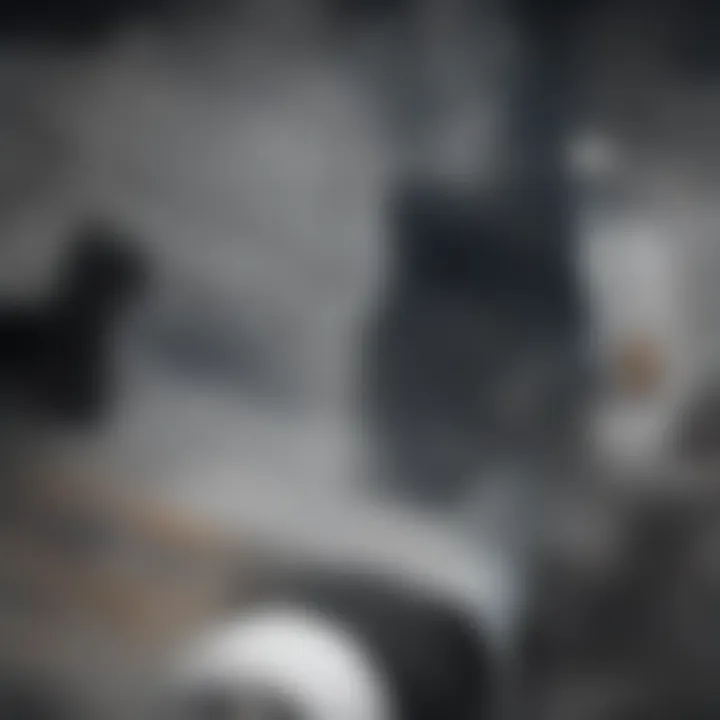
Vector construction is a fundamental step in developing sgRNA expression vectors. Several methods are commonly employed. Understanding these techniques can enhance the ability to create vectors tailored for specific applications.
Restriction Enzyme Digestion
Restriction enzyme digestion involves using specific enzymes to cut DNA at defined sequences. This method is crucial in the cloning process for introducing the sgRNA sequence into the vector backbone. One key characteristic of this technique is its precision; restriction enzymes enable the generation of cohesive ends that facilitate the insertion of the sgRNA into the vector.
The use of restriction enzyme digestion is popular because of its simplicity and efficiency. It allows for the selection of specific sites for insertion, which minimizes the risk of unwanted mutations. However, this method also has some limitations. Each restriction enzyme has a specific recognition site, which may not be present in the desired DNA fragment or vector. This can necessitate additional steps in the design process.
Ligation Techniques
Ligation techniques are employed to join DNA fragments together, usually following digestion. This process is vital for the seamless insertion of the sgRNA into the vector. The key characteristic of ligation is its reliance on the enzyme DNA ligase, which facilitates the formation of phosphodiester bonds between adjacent nucleotides.
Ligation is beneficial because it can work with various fragment sizes and does not require specific overhangs like some other methods. This technique allows for flexibility in vector design. That said, achieving a high efficiency of ligation can be challenging and may require optimization of conditions. The probability of forming undesired products also raises concerns during vector construction.
Gateway Cloning
Gateway cloning provides an advanced method for constructing sgRNA expression vectors, enabling the transfer of DNA sequences between different plasmids without the need for restriction enzymes or ligases. This method relies on site-specific recombination, where DNA fragments are exchanged between donor and destination vectors. A notable characteristic of Gateway cloning is its highly efficient and versatile nature, which streamlines the cloning process significantly.
This technique is preferred in many applications due to its rapidity and reliability. It allows for straightforward modular assembly of multiple sequences. However, one of the challenges includes the potential dependency on specific recombination sites which may limit flexibility. Additionally, the initial setup may require more extensive planning compared to traditional cloning methods.
The design of sgRNA expression vectors plays a significant role in the overall success of CRISPR applications, influencing precision and efficiency.
Through careful target sequence selection and appropriate vector construction techniques, effective sgRNA expression vectors can be developed. This groundwork leads to advancements in genetic research and potential therapeutic applications.
Methodologies for Transfection
Transfection is a crucial process in genetic engineering, particularly for the successful application of sgRNA expression vectors within CRISPR technology. By introducing the sgRNA into target cells, researchers can achieve specific modifications in the genome. Essentially, transfection methods dictate the efficiency and effectiveness with which sgRNAs are delivered into cells. Understanding the methodologies for transfection allows scientists to make informed decisions about which techniques provide the best outcomes for their specific experiments, based on factors such as target cells, required efficiency, and the desired duration of expression.
Chemical and Lipid-Based Methods
Chemical and lipid-based methods involve the use of reagents that facilitate the uptake of genetic material by cells. These methods tend to be simple and can be used on various cell types, making them highly versatile. They are particularly attractive for their ease of use and adaptability to high-throughput screening.
Lipid formulations, such as Lipofectamine or FuGENE, encapsulate nucleic acids, forming lipid-nucleic acid complexes that easily merge with the cell membrane, allowing for effective uptake. The choice of lipid can influence the efficiency of transfection significantly, and optimization is often necessary.
Some key benefits include:
- Non-viral delivery: Reduces potential safety concerns linked with viral vectors.
- Wide applicability: Can be used with different cell lines easily.
However, considerations such as cytotoxicity of certain compounds and variations in transfection efficiency across cell types can present challenges that researchers must navigate.
Physical Methods
Physical methods of transfection generally involve techniques that directly manipulate the target cells to facilitate nucleic acid uptake.
Electroporation
Electroporation involves applying an electrical field to cells, creating temporary pores in cell membranes. This method allows for the rapid delivery of sgRNA and other nucleic acids. A significant characteristic of electroporation is its efficiency with a variety of cell types, including those that are often difficult to transfect via traditional methods.
One unique feature of electroporation is the ability to control pulse duration and intensity, allowing researchers to optimize conditions according to the cell type. Its key advantage is the high transfection efficiency. However, one must consider:
- Cell viability: Not all cells survive the process, and optimizing conditions is critical.
- Equipment: Specialized equipment is required, which may not be readily available for all labs.
Microinjection
Microinjection involves physically injecting sgRNA directly into individual cells using a fine glass needle. This method allows for a very precise delivery. Its primary characteristic is the level of accuracy it enables when targeting specific cells, making it a beneficial choice when working with high-value or delicate cell systems.
A distinctive aspect of microinjection is that it can achieve high transfection efficiency, especially in embryos or cells requiring targeted delivery. Despite its advantages, microinjection has certain drawbacks:
- Labor intensity: It requires skilled personnel and is more time-consuming.
- Resolution for large cell populations: It is not suitable for large-scale applications due to its manual nature.
"Choosing the right transfection method is essential not only for the success of sgRNA delivery but also for the integrity of the host cells."
In summary, both chemical methods and physical methods like electroporation and microinjection present viable pathways for transfection, each carrying its benefits and limitations. Understanding these methodologies assists researchers in selecting the most appropriate techniques tailored for their specific experimental needs.
Systems for Vector Delivery
In the realm of sgRNA expression vectors, the choice of delivery system is crucial for the success of CRISPR technology. Efficient vector delivery ensures that synthetic guide RNAs are accurately introduced into target cells. It greatly enhances the efficacy of genetic modifications. The selection of either viral or non-viral methods has significant implications for research and therapeutic applications. Each system comes with its own set of benefits and considerations which researchers must carefully evaluate.
Viral Vectors
Viral vectors are often seen as efficient tools for delivering sgRNA because of their natural ability to infect host cells. These vectors can be engineered from various types of viruses, including lentiviruses, adenoviruses, and adeno-associated viruses (AAV).
Advantages of Viral Vectors:
- High Delivery Efficiency: Viral vectors have evolved to infect host cells with high efficiency. This makes them ideal for applications where high transduction rates are desired.
- Stable Expression: Many viral vectors, especially lentiviruses, can integrate into the host genome. This allows for long-term expression of sgRNA.
- Versatility: They can carry relatively large genetic payloads, allowing for the inclusion of multiple genes or long sgRNA sequences.
Despite these advantages, there are challenges associated with using viral vectors:
- Safety Concerns: There can be risks of uncontrolled expression or insertional mutagenesis. Proper safety measures are essential.
- Immune Response: The host immune system may recognize viral proteins, leading to an immune response that can hinder the effectiveness of the treatment.
"The choice of delivery system can significantly influence the outcome of CRISPR applications, making a thorough evaluation essential."
Non-Viral Delivery Systems
Non-viral delivery systems are an alternative to viral methods. These approaches do not rely on viruses to transport sgRNA into cells. Instead, they employ chemical, physical, or biological means. Some common non-viral methods include lipid-mediated transfection, electroporation, and microinjection.
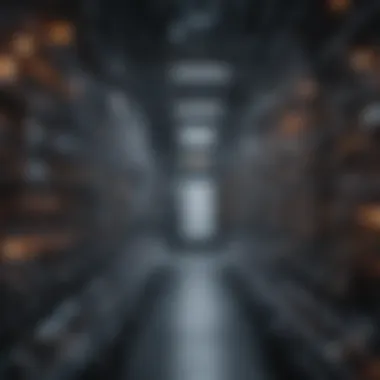
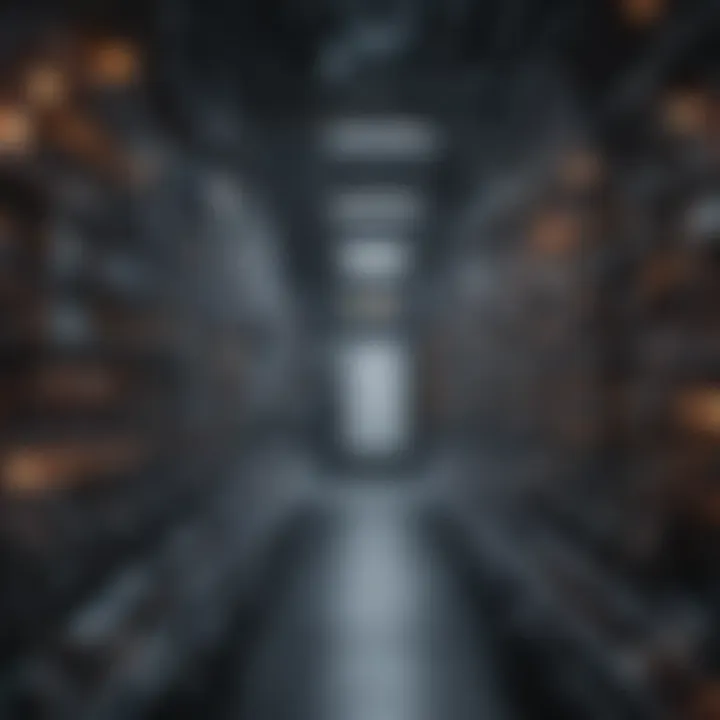
Key Benefits of Non-Viral Delivery Systems:
- Lower Safety Risks: Non-viral methods generally have a better safety profile, reducing the risk of an immune response or insertional mutations.
- Easier Manipulation: Non-viral systems allow for modifications to the delivery process. This flexibility can lead to the optimization of experimental conditions for specific targets.
- Cost-Effectiveness: Many non-viral methods are less expensive than viral vector production, making them an attractive option for many labs.
However, non-viral methods can present challenges as well:
- Lower Efficiency: Many non-viral techniques often have lower delivery efficiencies compared to viral methods.
- Transient Expression: Non-viral systems typically result in temporary expression of sgRNA, which may not be sufficient for all applications.
Evaluating Vector Efficiency
Evaluating vector efficiency is a crucial step in the development and application of sgRNA expression vectors. The efficiency with which these vectors operate directly impacts the success of CRISPR-based genome editing. When assessing vector efficiency, it is important to consider a variety of factors including the extent of gene editing, the specificity of the vector, and potential off-target effects.
Understanding these elements ensures that researchers can fine-tune their vectors for optimal performance. Techniques used for evaluation help in determining not only how well a vector delivers its payload but also how accurately it targets the desired genes without affecting other unrelated genes.
Assessment Techniques
Fluorescence Microscopy
Fluorescence microscopy allows researchers to visualize the location and expression of sgRNA within cells. This technique relies on the luminescence emitted by fluorophores linked to the sgRNA. One significant aspect of fluorescence microscopy is its ability to provide real-time observations of vector performance in living cells. This makes it a very beneficial choice for the evaluation process.
The key characteristic of fluorescence microscopy is its high sensitivity, which makes it possible to detect even low levels of sgRNA. Its unique feature is the ability to distinguish between different types of RNA within the same cell, providing a comprehensive overview of sgRNA distribution and activity. However, one disadvantage is that it can require complex imaging setups and expertise to interpret the results correctly.
Flow Cytometry
Flow cytometry is another powerful technique for assessing vector efficiency, particularly for quantifying the expression of sgRNA in a large population of cells. This method separates cells as they pass through a laser, allowing for precise measurements of multiple parameters simultaneously. The key characteristic of flow cytometry is its ability to analyze thousands of cells per second, providing statistical power to the results of the evaluation.
The unique feature of flow cytometry includes the capability to sort cells according to specific markers, such as fluorescent tags on the sgRNA. This is advantageous for isolating successfully edited cells for further study. However, flow cytometry can be expensive due to the required technology and may involve complex preparation of cell samples.
Analysis of Editing Outcomes
Analyzing editing outcomes is vital for confirming the functionality of sgRNA vectors. This part of evaluation involves ensuring that the intended genetic modifications have occurred while minimizing unintended changes. Various methods can be employed to assess editing outcomes.
Surveying Methods
Surveying methods encompass a range of techniques designed to analyze genomic alterations post-editing. One specific aspect of these methods is their quantitative nature, allowing researchers to measure the frequency of successful edits within a sample.
The key characteristic of surveying methods is their ability to provide insights into both on-target and off-target effects of the genetic modification. They are beneficial because they can help researchers understand the comprehensive impact of their edits on the genome. However, they can be time-consuming and may require additional validation through sequencing technologies.
NGS Techniques
Next-Generation Sequencing (NGS) techniques provide a high-throughput approach to analyze gene editing outcomes. NGS allows for the complete sequencing of regions where edits are intended, thus making it easier to detect any modifications that have occurred. This approach is particularly useful for assessing large-scale editing projects.
The key characteristic of NGS techniques is their ability to deliver a substantial amount of data in a relatively short period. They are popular due to their precision and depth of analysis. However, the high cost associated with NGS can be a barrier for some researchers, particularly in smaller labs with limited budgets.
Overall, a thorough evaluation of vector efficiency is essential, ensuring that CRISPR applications achieve their intended goals while maintaining the integrity of the organism's genome.
Challenges in Vector Development
The advancement of sgRNA expression vectors has opened numerous avenues in genetic engineering. However, several significant challenges exist in their development. These challenges directly impact the effectiveness and reliability of CRISPR technology. Understanding these hurdles is crucial for improving sgRNA vector designs, which ultimately leads to more accurate genome editing.
Off-Target Effects
One prominent challenge is the issue of off-target effects. This term refers to unintended changes in the genome that can occur when sgRNA mistakenly binds to areas other than the intended target. Such effects can lead to unpredictable consequences in cells, making it a critical concern in both research and therapeutic settings. Minimizing off-target activity is essential for ensuring that genome editing is precise and safe. Researchers are actively exploring various ways to enhance sgRNA specificity. This includes modifications to the sgRNA sequence and utilizing high-fidelity Cas proteins.
Off-target effects not only complicate the interpretation of experimental results but also raise ethical questions regarding safety. For instance, in therapeutic applications, any unintended edits could result in adverse outcomes, potentially compromising patient safety. Thus, rigorous validation processes are necessary to assess and mitigate these risks effectively.
Delivery Efficiency
Another significant challenge in sgRNA vector development is achieving high delivery efficiency into target cells. The effectiveness of CRISPR technology hinges on the successful introduction of the sgRNA and Cas protein into the desired cell type. Various factors influence delivery efficiency, including the choice of delivery systems, physical barriers within tissues, and cell types’ inherent characteristics.
Different delivery methods, such as viral vectors, electroporation, and lipid-based formulations, come with their trade-offs. For example, while viral vectors can achieve high transfection rates, they may trigger immune responses, which could limit their use in clinical applications. Conversely, lipid-based methods are generally less immunogenic but might not be effective for all cell types.
Moreover, optimizing vector design plays a vital role as well. Here, researchers must strike a balance between vector stability and expression levels, ensuring that sgRNA remains functional once delivered to the target cell. This complexity adds another layer to the development process.
Addressing these challenges requires a multi-faceted approach. Collaboration across disciplines, thorough testing, and continual innovation in vector technology are necessary to enhance the effectiveness of sgRNA expression vectors.
"It is imperative for the scientific community to focus on overcoming these challenges to fully realize the potential of CRISPR technology in genetic engineering."
By addressing off-target effects and improving delivery efficiency, researchers can pave the way for safer and more effective applications of sgRNA expression vectors in gene therapy and beyond.
Advancements in sgRNA Vector Technology
The evolution of sgRNA vector technology represents a significant leap in the field of genetic engineering. This advancement is pivotal, as it enhances the efficiency and specificity of CRISPR-based editing systems. The development of more refined sgRNA expression vectors can lead to improved results in gene editing applications.
Innovative Vector Designs
Recent innovations in vector design have focused on increasing both the versatility and efficiency of sgRNA delivery. For instance, the incorporation of synthetic promoters can provide tighter control over sgRNA expression. This allows for temporal regulation, enabling scientists to activate or repress gene editing when desired. Additionally, using self-cleaving ribozymes can reduce by-products from sgRNA production, increasing the yield of the functional guide RNAs.
Moreover, advancements in nanoparticle technology have introduced non-viral delivery methods that present fewer risks than traditional viral vectors. These novel vectors can encapsulate sgRNA and deliver it directly to target cells, improving cellular uptake and minimizing degradation. Enhanced delivery tools result in better editing outcomes and reduced off-target effects, a prime concern in CRISPR usage.
Next-Generation CRISPR Components
The integration of next-generation components into sgRNA vector technology aims to expand the scope of applications. For example, variations of Cas proteins, such as Cas9 and Cas12 (Cpf1), offer different cutting mechanisms and target specificities. By designing sgRNA vectors that can express various Cas proteins, researchers have access to a broader toolkit for genome editing.
Incorporating elements such as enhanced specificity modules also plays a crucial role in minimizing off-target effects. These modules can be engineered into the sgRNA sequence, guiding the Cas proteins more accurately to the intended sites in the genome. The advancements provide prospects for safer and more effective gene editing solutions.
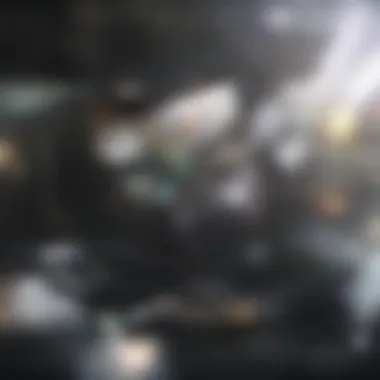
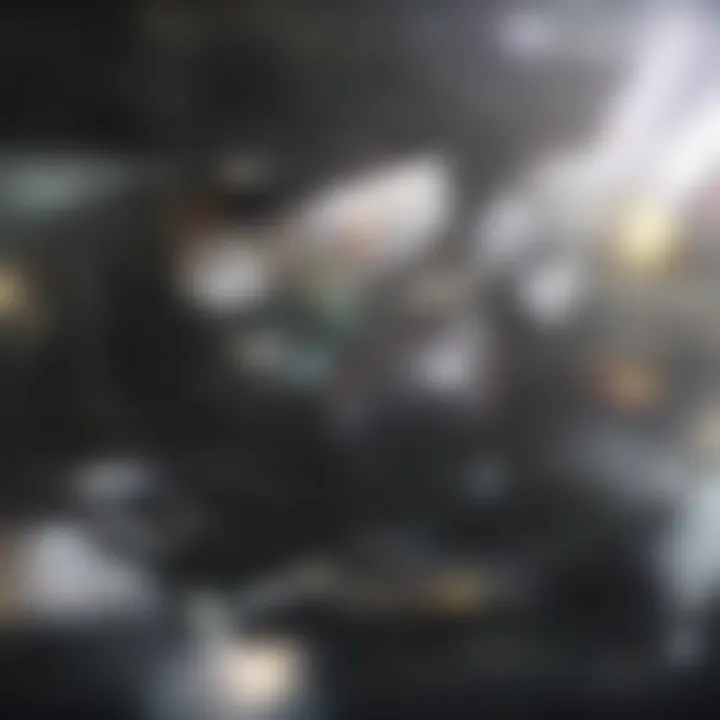
"The continual refinement of sgRNA expression vectors is crucial for the future of genetic research and therapy."
Overall, the advancements in sgRNA vector technology not only open new avenues for research but also hold significant promise for therapeutic applications. The focus on innovative designs and next-generation components will likely influence various fields, such as functional genomics and personalized medicine.
Applications in Research
Research utilizing sgRNA expression vectors has spurred significant advancements in the fields of genetics and molecular biology. These vectors enable scientists to manipulate genetic material with a level of precision that was previously unattainable. Primarily used in CRISPR technology, sgRNA expression vectors facilitate targeted genome editing, allowing researchers to probe the functions of genes in various cellular contexts.
Importance of Applications in Research
The importance of sgRNA expression vectors in research cannot be overstated. They serve as fundamental tools for functional genomics, enabling scientists to explore gene functions and regulatory networks effectively. By directing the CRISPR-associated protein Cas9 to specific genomic sites, researchers can induce targeted mutations, deletions, or insertions. This ability opens doors to understanding gene functions, validating gene targets, and dissecting complex genetic interactions.
Benefits and Considerations
- Precision: The specificity of sgRNA prevents off-target effects compared to older techniques. This reliability enhances the validity of experimental results.
- Efficiency: sgRNA expression vectors allow for multiple genes to be targeted simultaneously, accelerating discovery.
- Versatility: They are applicable in a variety of model organisms, from bacteria to mammals, broadening the scope of research.
- Cost-Effectiveness: As techniques mature, the reduction in required resources for gene editing increases accessibility for laboratories.
Despite these benefits, challenges remain. The potential for off-target modifications, even if reduced, requires thorough validation strategies. Furthermore, ensuring efficient delivery to target cells remains an ongoing endeavor in the field. Understanding these dynamics is crucial for researchers aiming to harness the full potential of sgRNA expression vectors.
Functional Genomics
Functional genomics is one of the most prominent applications of sgRNA expression vectors. This field aims to reveal the functions of genes and their interactions within the genome. sgRNA vectors enable high-throughput screening approaches, where researchers can systematically knock out or modify genes and observe resulting phenotypic changes.
- Genome-Wide Screening: By creating libraries of sgRNAs targeting various genes, scientists can assess gene function on a large scale. This is particularly useful in identifying essential genes in specific biological pathways.
- Pathway Analysis: sgRNA vectors help unravel complex biological pathways by allowing researchers to manipulate components systematically.
- Disease Association: Functional genomics using sgRNAs can help link genetic variations to disease phenotypes, leading to a deeper understanding of disease mechanisms.
Disease Modeling
Disease modeling represents a crucial area where sgRNA expression vectors have made a profound impact. By inducing specific genetic alterations in model organisms or cell lines, researchers can mimic human diseases more closely. This modeling is particularly beneficial for studying genetic disorders, cancers, and other conditions where specific mutations play a significant role.
- Model Organisms: sgRNA vectors are employed in organisms such as mice, zebrafish, and yeast to create models that carry human disease-associated mutations.
- Cellular Models: Human cell lines can be engineered to exhibit disease-specific mutations, facilitating in-depth study of cellular responses to treatments.
- Drug Development: Understanding disease mechanisms through these models paves the way for the development of novel therapeutic strategies, including personalized medicine approaches.
Utilizing sgRNA expression vectors for disease modeling not only accelerates the understanding of genetic diseases but also enhances the development of targeted therapies, truly bridging the gap between bench and bedside.
In summary, the applications of sgRNA expression vectors in research are vast and varied. They contribute significantly to functional genomics and disease modeling, providing invaluable insights and tools for researchers dedicated to deciphering complex genetic landscapes.
Therapeutic Implications
The exploration of sgRNA expression vectors reveals a vast landscape in the context of therapeutic implications. These vectors serve as critical tools in advancing medical science, particularly in precision medicine and genomic therapies. The integration of sgRNA expression vectors into therapeutic strategies opens doors to novel applications, including gene replacement therapies and cancer research. By understanding the nuances of these implications, researchers and clinicians can better assess their potential to address complex genetic disorders and malignancies.
Gene Replacement Therapies
Gene replacement therapy is a pivotal application of sgRNA expression vectors. This method aims to replace defective or missing genes to treat genetic disorders. The efficiency with which sgRNA vectors can deliver corrective sequences into target cells underlies their therapeutic promise.
In many genetic diseases, such as cystic fibrosis or muscular dystrophy, specific genes are mutated or absent. Utilizing sgRNA vectors allows the insertion of healthy gene copies directly into the genome of affected cells. This approach can restore normal function, potentially leading to significant improvements in health outcomes.
The advantages of this therapy are numerous:
- Specificity: sgRNA vectors can be designed to target exact genetic locations, minimizing off-target effects.
- Efficiency: Advanced delivery systems enhance the uptake of sgRNA vectors into the target cells, increasing the success rate.
- Versatility: These therapies can be adapted for various genetic conditions, broadening their applications.
However, there are important considerations. Safety remains a primary concern, particularly regarding the long-term effects of genome editing. There are also regulatory hurdles that must be navigated before these therapies can be applied in clinical settings. Furthermore, the potential for immune responses against the delivered vectors poses additional challenges that require careful assessment.
Cancer Research Applications
In cancer research, sgRNA expression vectors are proving to be invaluable. They provide insights into the genetic underpinnings of cancer and facilitate the development of targeted therapies. By using these vectors, researchers can edit genes in cancer cells to study their roles in tumor development and progression.
The therapeutic potential in this context includes:
- Targeting Oncogenes: sgRNA expression vectors can be utilized to knock out specific oncogenes, which are responsible for driving tumorigenesis. This loss of function can reduce the growth of tumors and make them less aggressive.
- Enhancing Immune Response: By editing genes related to immune evasion, sgRNA vectors can help re-establish effective immune responses against tumors. This approach can enhance the efficacy of immunotherapies.
- Modeling Cancer: sgRNA vectors allow for the creation of patient-specific models of cancer. This facilitates better understanding and testing of therapeutic strategies in a controlled environment.
The challenges in cancer research using sgRNA expression vectors also exist. For instance, achieving precise delivery to target tissues remains difficult. Moreover, heterogeneity within tumor populations can complicate outcomes, as not all cells may respond uniformly to treatment.
Understanding the therapeutic implications of sgRNA expression vectors is crucial for shaping future research and clinical applications. Their potential to revolutionize treatments hinges on addressing the challenges and maximizing their effectiveness.
Ethical Considerations
Ethics serves as a vital framework within the realm of genetic engineering, particularly in the context of CRISPR technology and sgRNA expression vectors. Understanding the ethical implications related to these tools is essential for responsible research and application. The conversation surrounding ethics often encompasses biological safety and societal implications, which significantly impact the future trajectories of genetic technologies.
Biological Safety
Biological safety addresses the containment and control of biological materials. When working with sgRNA expression vectors, the risk of unintended effects or outcomes must be carefully evaluated. This involves assessing potential off-target effects that could result in undesirable genetic modifications. The use of CRISPR technology has raised alarms regarding its safety in human subjects, especially when considering gene editing at the embryonic level. Establishing stringent safety protocols in research and practice ensures that applications of sgRNA vectors minimize risks and safeguard both researchers and the broader community.
Key considerations in biological safety include:
- Rigorous testing of sgRNA designs to evaluate specificity.
- Development of innovative delivery systems that reduce exposure risks.
- Mandatory pathogen control protocols in laboratories.
"It’s critical that scientific advancements do not outpace our ethical considerations; mishaps could set back the field of genetics significantly."
Societal Implications
The societal implications of using sgRNA expression vectors extend beyond the laboratory. There are profound moral, legal, and social dimensions to consider. Public perception shapes the acceptance of genetic technologies. As gene editing moves into therapeutic applications, we must engage with communities to educate them about the benefits and risks involved. Transparency in communication can foster trust and collaborative efforts among scientists, ethicists, and the general public.
Some of the societal implications include:
- Access to technology: Ensuring that cutting-edge gene therapies are accessible to a broad segment of the population.
- Equity in treatment: Addressing potential disparities that may arise in healthcare, particularly among marginalized groups.
- Regulatory frameworks: The need for robust policies to govern the use and application of CRISPR technology to mitigate misuse.
Ending
The conclusion of our exploration into sgRNA expression vectors highlights the critical role these vectors play in advancing CRISPR technology and genetic research. Understanding the design, function, and applications of sgRNA vectors impacts not only the efficiency of genome editing but also the safety and specificity of genetic modifications.
Future Directions in Vector Research
The future of vector research is deeply intertwined with the evolution of genetic engineering techniques. Some key areas for future exploration include:
- Improved Delivery Mechanisms: Developing more efficient and less invasive methods for delivering sgRNA to target cells remains a priority. Enhanced viral and non-viral delivery systems could reduce off-target effects and improve precision.
- Therapeutic Applications: As therapeutic applications of CRISPR technology expand, new vectors tailored for specific diseases or cell types will be necessary. Research into vector designs that optimize therapeutic outcomes is essential.
- Multi-Target Approaches: Future vectors may incorporate multiple sgRNAs to edit several genes simultaneously. This could revolutionize research into complex traits and diseases that involve multiple genetic factors.
- Synthetic Biology: Integrating synthetic biology with sgRNA expression vectors presents exciting opportunities for engineering new biological functions or systems.