Examining DAPI Spectra: Insights and Applications
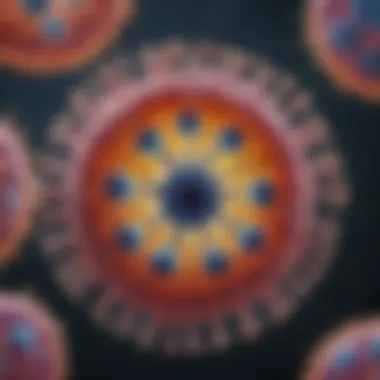
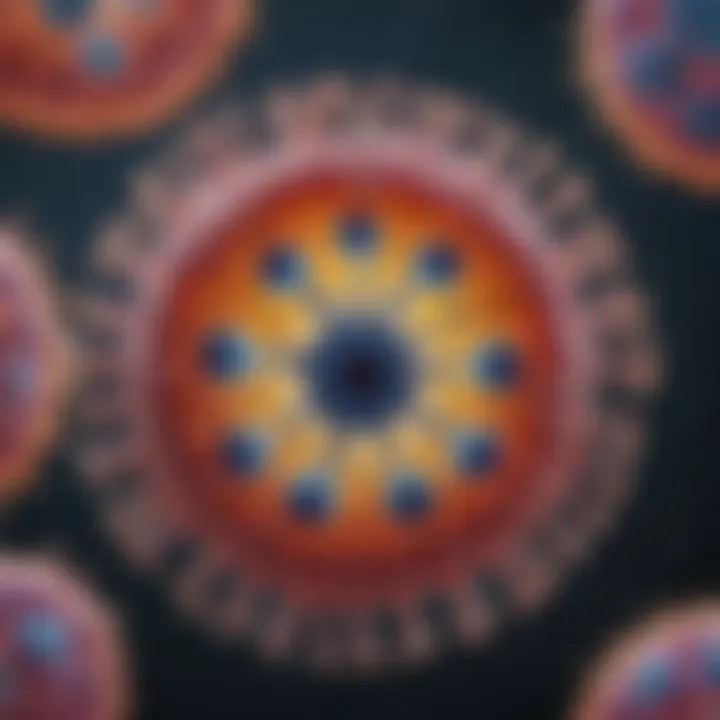
Intro
In the world of biological and chemical research, visualizing nucleic acids is no small feat. Researchers often find themselves wrestling with the complexities of traditional staining techniques. One prominent solution that stands out is DAPI, a fluorescent stain that binds specifically to DNA. Its spectral properties make it a key player in cell imaging and molecular biology. Understanding DAPI spectra goes beyond simply knowing how to apply the stain; it necessitates a grasp of the underlying principles, characteristics, and a variety of its applications.
Over the years, the mounting need for reliable and precise methods to visualize nucleic acids has engendered a deeper investigation into DAPI. What began as a practical innovation has blossomed into an essential component of numerous research projects that seek to unearth the very fundamentals of life at the cellular level.
DAPI, with its unique spectral qualities, facilitates the detection of nucleic acids in various samples. From plant cells to cultured human tissues, the applications for DAPI staining are broad, highlighting its integral role within scientific exploration. In this article, we engage with the nuances of DAPI spectra, focusing on its significance, applications, and the research methodologies underpinning its use.
Research Background
Overview of the scientific problem addressed
At the heart of molecular biology is the quest to understand genetic material. Nucleic acids serve as the blueprint of life, dictating cell behavior and functionality. However, visualizing these macromolecules accurately poses challenges, particularly when it comes to distinguishing between DNA and RNA in complex samples. Conventional techniques often yield mixed results, leading to inconsistencies in data interpretation and analysis. DAPI stands as a contender to tackle these hurdles through its selective binding to DNA.
Historical context and previous studies
The journey of DAPI traces back to the mid-20th century, when it was first synthesized as a part of synthetic dyes. Initially, its applications were limited, enticing researchers to explore its potentiality deeper. Pioneering studies laid the groundwork for its eventual adoption in fluorescence microscopy. The subsequent decades ushered in a slew of research demonstrating its effectiveness and reliability in nucleic acid detection. Notably, advancements in imaging technology and the quest for higher resolution have only served to amplify its relevance. Recent investigations continue to refine DAPI's use, offering insights into how spectral characteristics can influence results in varied research contexts.
As we delve deeper into the intricacies of DAPI spectra, understanding its historical evolution informs our appreciation of its current applications. It allows us to recognize the ingenuity behind fluorescence microscopy and its impact on our comprehension of life at a molecular level.
"Understanding the properties of DAPI not only illuminates the path for researchers but also connects layers of molecular biology that once seemed opaque."
This narrative serves as a guide to unravel not just the spectral analysis of DAPI, but also its significance in advancing methodologies within diverse scientific fields.
Understanding DAPI
Understanding DAPI is a cornerstone in the realm of molecular biology and chemical analysis. The compound, 4',6-diamidino-2-phenylindole, is particularly celebrated for its ability to bind to nucleic acids in a manner that facilitates visualization under specific lighting conditions. This property has opened doors in a variety of applications, from basic research to clinical settings.
The significance of grasping the nuances of DAPI cannot be understated. For researchers, the importance lies in its practical utility—imagine a technique that allows one to pinpoint genetic material with precision. With DAPI, it’s as if the complexities of DNA are illuminated, enabling clearer interpretations and further exploration. Moreover, it offers a relatively straightforward approach to analyzing cellular structures, allowing for quick assessments in various biological investigations.
However, navigating the waters of DAPI requires a solid understanding of its chemical structure and properties, as well as its mechanism of action. This information plays a vital role in ensuring the accuracy and reliability of experiments. The following outlines emphasize the core elements associated with DAPI:
- Chemical Properties: Understanding how DAPI interacts with different compounds in a sample is crucial. Its chemical makeup influences how it fluoresces under UV light, giving insights into cell viability and nucleic acid content.
- Mechanism of Binding: Comprehending the binding process can aid in optimizing experimental conditions, thus yielding more consistent and reproducible results.
In light of these considerations, one can appreciate that the knowledge surrounding DAPI stretches far beyond mere usage. It's about leveraging its capabilities to enhance scientific insight.
"Fluorescence microscopy with DAPI doesn't just reveal structures; it brings to life the narratives hidden within cellular interactions."
Delving deeper into the intricacies of DAPI will unveil the mysteries of its Chemical Structure and Properties, as well as its Mechanism of Action. This understanding equips scientists with the tools they need to unlock the full potential of their research.
DAPI Spectra Overview
DAPI spectra play a significant role in various branches of scientific research, particularly in the fields of cell biology, genetics, and clinical diagnostics. Understanding the nuances of DAPI spectra is essential for researchers looking to leverage its capabilities to visualize and quantify nucleic acids effectively. The importance of this topic lies in its ability to bridge the gap between theoretical knowledge and practical application. By mastering DAPI spectra, scientists can significantly enhance their research methodologies, leading to clearer insights and more reliable results.
Definition and Importance
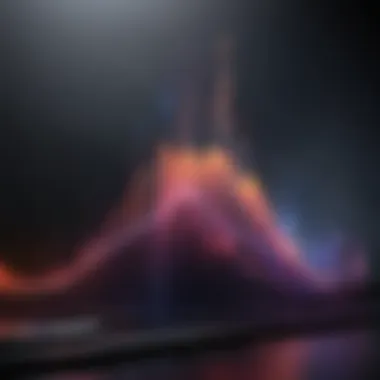
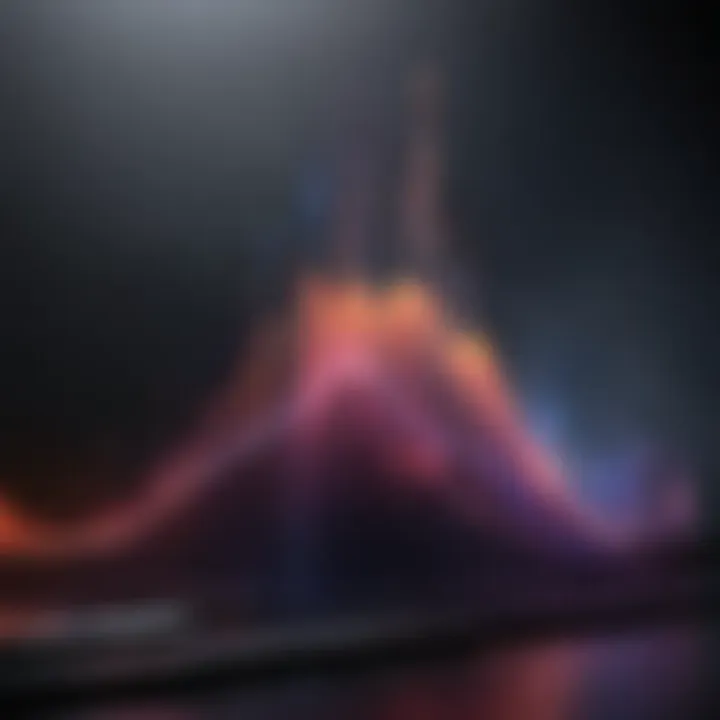
DAPI, or 4',6-diamidino-2-phenylindole, is a fluorescent stain that specifically binds to A-T rich regions in DNA. The importance of DAPI spectra stems from its ability to provide high-contrast images of cellular components, making it a critical tool in the visual analysis of nucleic acids. In layman's terms, DAPI acts like a spotlight on the DNA within a cell, illuminating it in a way that researchers can visualize and count. Its specificity allows for quantitative assessments of DNA within cellular samples, making it indispensable in various studies, including those assessing cell viability and apoptosis.
When discussing the definition of DAPI spectra, it is crucial to note how spectra reflect the absorption and emission characteristics of the dye. Since DAPI emits a bright blue fluorescence when excited by specific wavelengths, researchers can use spectral data to determine the concentration of DNA in a sample and assess other essential metrics.
Spectral Range and Properties
The spectral range of DAPI is particularly noteworthy, as it exists in the ultraviolet region of the electromagnetic spectrum. DAPI has two primary excitation peaks—one around 358 nm and another at 460 nm—which makes it suitable for use with multiple fluorescence microscopy systems. This dual excitation capability can be invaluable for a myriad of applications, enabling researchers to choose the optimal wavelengths that align with their experimental design.
Some properties of DAPI that further enhance its utility include:
- Stability: DAPI remains stable under typical laboratory conditions, allowing for reproducible experimental results.
- Cell Permeability: The dye can readily penetrate live cells, enabling real-time imaging and analysis without necessitating cell fixation.
- Low Background Fluorescence: This minimizes signal interference, improving the clarity of images captured during experiments.
However, despite its myriad benefits, DAPI is not without limitations, such as phototoxicity and signal interference, which will be further elaborated upon in later sections. Nonetheless, appreciating its spectral characteristics and properties is paramount for effective application in both academic and clinical settings.
"The unique aspects of DAPI spectra make it a staple in the toolkit of modern biologists, opening up numerous pathways for exploring cellular processes and disease mechanisms."
In summary, the exploration of DAPI spectra underscores a blend of definition, importance, and nuanced understanding of its spectral range and properties, making it a vital consideration in ongoing research.
Spectral Analysis Techniques
Understanding the methods used in spectral analysis is crucial for any researcher utilizing DAPI in their studies. Both fluorescence microscopy and flow cytometry serve distinct yet complementary roles in analyzing DAPI spectra. Their effectiveness lies not just in capturing data, but also in how they illuminate the finer details of nucleic acid interactions.
Fluorescence Microscopy
Fluorescence microscopy is one of the cornerstones of DAPI analysis. It leverages the unique properties of fluorescence to visualize and quantify nucleic acids within cells. The technique operates on the principle that certain dyes, such as DAPI, emit light upon excitation with a specific wavelength. When used in microscopy, this allows researchers to pinpoint areas of interest at a cellular level, unveiling the intricate dynamics of nucleic acids.
- Advantages: The precision of fluorescence microscopy is unmatched when it comes to spatial resolution. It enables researchers to examine the relationships between nucleic acids and cellular structures in real-time. This is particularly useful in cell biology, where observing live interactions can yield insights that static images cannot.
- Considerations: However, several factors can affect the efficacy of this technique. Light exposure can lead to phototoxicity, especially when prolonged, which can alter cell behavior. Additionally, proper filter sets must be used to ensure accurate spectral measurement, as using the wrong filter can muddle the data.
"Fluorescence microscopy offers a window into the world of cells where DAPI acts as a guide, illuminating the pathways of nucleic acids."
Flow Cytometry
Flow cytometry provides another layer of analysis for DAPI spectra. Unlike fluorescence microscopy, this technique handles large populations of cells in a short time, quantifying and characterizing cells based on the fluorescence emitted by DAPI. The rapid throughput makes it invaluable in applications such as clinical diagnostics and environmental monitoring.
- Advantages: Flow cytometry excels in yielding quantitative data. By analyzing thousands of cells per second, it provides robust statistics on nucleic acid content and cell cycle phases. Furthermore, it can detect subtle differences in fluorescence intensity, translating to precise measurements of nucleic acid concentrations.
- Considerations: Flow cytometry does require careful sample preparation. The cells must be in a single-cell suspension, free from aggregations that could skew results. Additionally, the complexity of analysis can necessitate sophisticated software to interpret the vast amounts of data generated.
Interpreting DAPI Spectra Data
Interpreting DAPI spectra data is crucial for advancing research in various fields such as cell biology, clinical diagnostics, and environmental monitoring. Understanding the fluorescence emitted from DAPI-stained samples can provide significant insights into nucleic acid presence and organization. Inaccurate interpretation can lead to flawed conclusions, which is why attention to detail and methodology is paramount.
Fluorescence Intensity Measurements
Fluorescence intensity measurements are the backbone of DAPI spectral analysis. This technique involves quantifying the amount of light emitted from a sample when exposed to specific wavelengths. The intensity of fluorescence is directly related to the quantity of DNA or RNA present in the sample. Thus, accurate measurement provides a reliable indicator of nucleic acid content.
To perform these measurements, several factors must be taken into account:
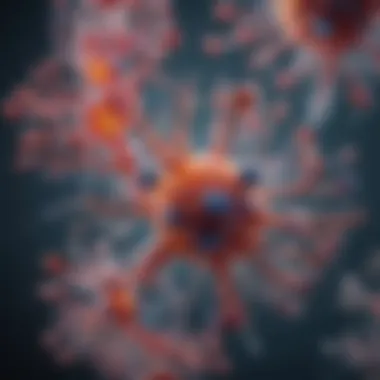
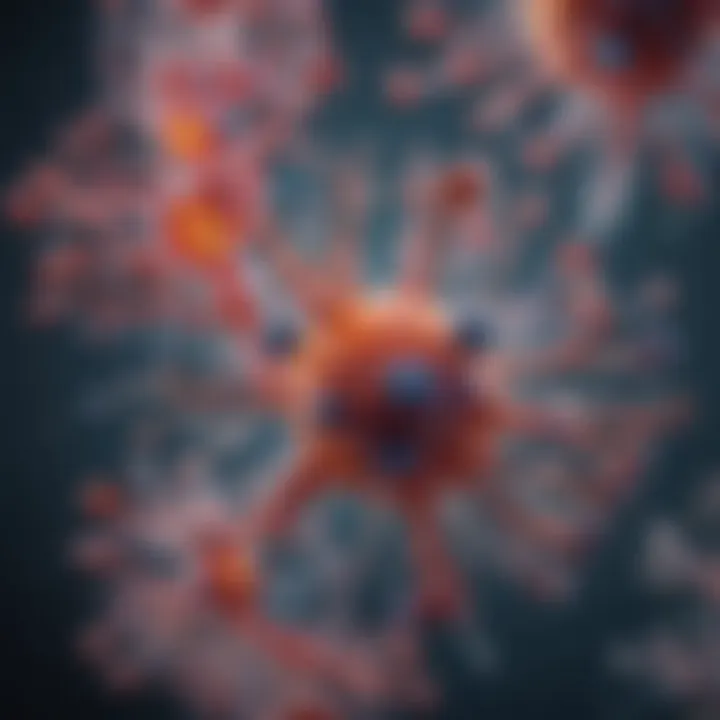
- Calibration: Prior to any measurement, one should ensure that the fluorescence microscope is calibrated. Calibration ensures that the intensity readings are consistent and comparable across different samples.
- Sample Preparation: Properly preparing samples can significantly affect readings. For instance, overly thick samples may lead to light scattering that diminishes intensity readings. Performing a dilution or optimizing the thickness can yield clearer results.
- Environmental Conditions: Ambient light and temperature can skew fluorescence readings. Conducting experiments in a controlled environment is advisable to eliminate variability.
Furthermore, to quantify fluorescence, researchers often utilize software tools that analyze the intensity data. The programs help in transforming raw data into usable formats.
Quantitative Analysis
Quantitative analysis of DAPI spectra data delves deeper, allowing researchers to derive statistically significant conclusions from the fluorescence intensity measurements. Here, the goal is to not just measure the intensity but to interpret it in the context of biological questions.
One reliable method of quantitative analysis involves creating a standard curve. This curve is developed by plotting known concentrations of nucleic acids against their corresponding fluorescence intensities. Interpolating unknown sample readings against this curve allows for accurate quantification of nucleic acid concentration in those samples.
Key considerations in this aspect include:
- Replicates: Running multiple replicates of each sample not only enhances reliability but also allows for averaging out anomalies.
- Controls: Using negative and positive controls is necessary. Controls ensure that the assay conditions are ideal and help in detecting any possible interferences.
- Statistical Analysis: Employing statistical methods such as ANOVA or t-tests can determine the significance of the results, offering a more profound understanding of the data.
Overall, robust interpretation of DAPI spectra data involves careful measurement and quantitative analysis, providing indispensable insights into nucleic acid studies and beyond. By dissecting the fluorescence characteristics, researchers can discover patterns that may point to significant biological processes or anomalies. In the long run, mastering this process will bolster the reliability of experimental results, enhancing the credibility of scientific work.
Applications of DAPI Spectra
DAPI spectra serve as more than just a method of visualizing nucleic acids; they form the backbone of various essential applications across scientific disciplines. Understanding these applications not only illuminates the utility of DAPI but also reveals its role in expanding the horizons of current research methodologies. The capability of DAPI to bind to DNA and fluoresce under specific wavelengths places it firmly in the toolkit of biologists, clinicians, and environmental scientists alike. This section discusses the prominent applications of DAPI spectra and considers the crucial aspects of each domain that benefit from its use.
Cell Biology and Nucleic Acid Quantification
In the realm of cell biology, DAPI has become synonymous with DNA visualization. Its ability to stain nuclear material with striking clarity allows researchers to assess cellular structures easily. By utilizing fluorescence microscopy, scientists can not only observe cell morphology but also evaluate processes like cell division and apoptosis. The significance of this is heightened when one considers that accurate quantification of nucleic acids can affect experimental outcomes.
- Benefits of DAPI in Cell Biology:
- Precise Imaging: The sharp fluorescence intensity enables researchers to pinpoint and distinguish between various phases of cell activity.
- Quantitative Insights: Applying DAPI for quantifying nucleic acids facilitates an understanding of gene expression, cell cycle progression, and other cellular dynamics.
- Multiplexing Capabilities: DAPI can be used alongside other fluorescent markers, providing a multifaceted view of cellular functions.
"DAPI is a cornerstone in the toolkit of molecular biologists, allowing for the dissection of cellular functions and dynamics with remarkable precision."
Clinical Diagnostics
In clinical settings, DAPI fluorescence proves invaluable for diagnosing diseases by enabling the visualization of genetic material directly from patient samples. When combined with modern techniques such as fluorescence in situ hybridization (FISH), DAPI aids in identifying chromosomal abnormalities which could indicate malignancies. The dual benefits of identifying cells and simultaneously quantifying nucleic acids enhance the robustness of diagnostics.
- Key Considerations for Clinical Diagnostics:
- Rapid Identification: DAPI allows for quick processing of samples, expediting diagnosis timelines.
- Sensitivity: DAPI's high affinity for DNA permits the detection of small quantities of nucleic acids, crucial in identifying early-stage cancers or other genetic diseases.
- Standardization: DAPI protocols are proliferating in laboratories, providing a reliable method to normalize results across various cases.
Environmental Monitoring
DAPI's applications extend beyond the biology lab and clinic, finding its place in environmental monitoring. The ability to assess the quality of water by examining microbial communities hinges on techniques like flow cytometry, where DAPI is employed to quantify DNA from environmental samples. With increasing concerns about pollution and its impacts on ecosystems, DAPI facilitates assessments of biodiversity and environmental health.
- Environmental Applications of DAPI:
- Microbial Analysis: DAPI can stain live and dead microorganisms, offering vital information about microbial population dynamics.
- Pollution Assessment: By monitoring organisms in contaminated sites, DAPI can help gauge the impact of pollutants on community structure.
- Research on Ecosystem Health: Understanding the abundance and diversity of nucleic acids in various ecosystems provides insight into overall ecological balance.
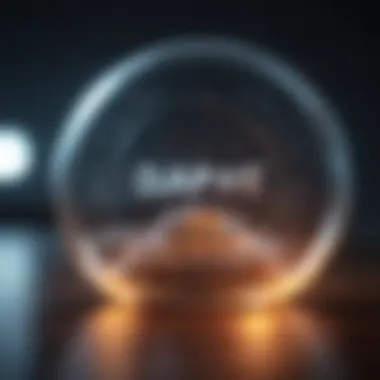
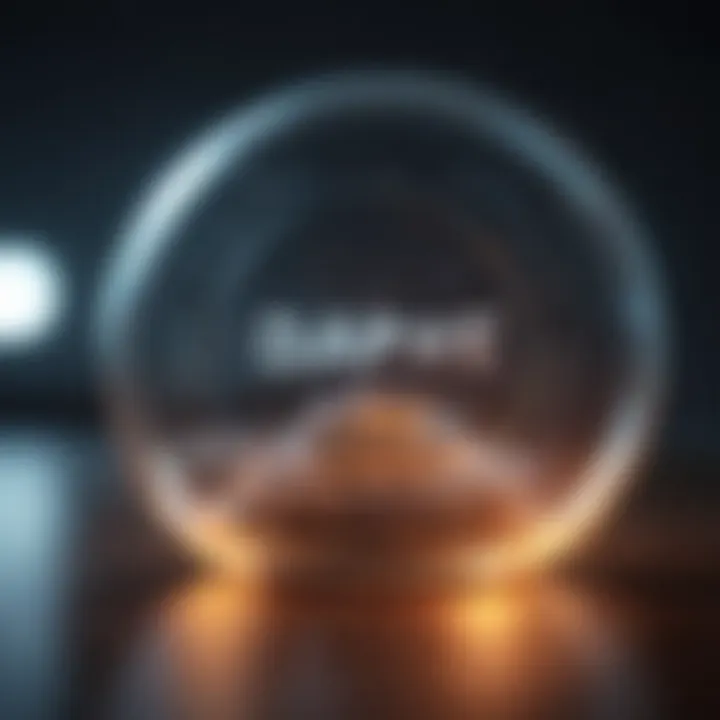
In sum, the applications of DAPI spectra stretch across various fields, shedding light on complex biological systems, improving clinical outcomes, and driving ecological research. With a finger on the pulse of scientific advancement, DAPI continues to be a vital tool for researchers aiming to bridge the gap between observation and understanding.
Limitations of DAPI Spectra
DAPI spectra, while invaluable in the realm of nucleic acid research, do come with their set of limitations. Understanding these constraints is paramount for researchers and practitioners who rely on DAPI for their investigations. This section will highlight some critical issues surrounding the use of DAPI spectra, particularly focusing on phototoxicity, interference, and specificity concerns. When grappling with experimental data, it’s vital to weigh these limitations against the benefits DAPI has to offer.
Phototoxicity and Interference
Phototoxicity refers to damage caused to cells when exposed to light during fluorescence microscopy. DAPI spectra, which are excited under UV light, are particularly susceptible to inducing this phenomenon. Overexposure can lead to cellular damage, prompting unintended cell death or alterations in nucleic acid integrity. This is especially crucial in live-cell imaging where the viability of the cells must remain intact for valid observations.
Moreover, the interference from other fluorescent agents can complicate the analysis. DAPI is known to exhibit spectral overlap with several other fluorophores, which can lead to misleading interpretations of fluorescence. For instance, in multi-channel imaging studies, if a researcher is not cautious, they may inadvertently attribute fluorescence signals to the wrong source.
Thus, it is essential to use controlled experimental conditions. Reducing exposure time and applying proper filters can help mitigate phototoxic effects as well as create a clearer distinction between signals from DAPI and those from other fluorophores.
Specificity Concerns
Specificity is another pressing issue with DAPI spectra. While DAPI exhibits a high affinity for DNA, particularly binding to A-T rich regions, it’s not entirely foolproof. The binding can sometimes lead to non-specific interactions with RNA and other cellular components. This lack of specificity can skew results, particularly in applications involving mixed nucleic acid populations.
Moreover, researchers need to be cognizant of the potential background fluorescence that can arise from cellular debris or autofluorescence from the samples themselves. Such background noise can impair the accurate interpretation of DAPI spectra, leading to challenges in quantifying nucleic acid content accurately.
Ultimately, it’s crucial for researchers to not only recognize DAPI’s strengths but also account for its limitations. Ensuring that experimental designs control for these factors can lead to more reliable data and more nuanced interpretations in various fields, from clinical diagnostics to environmental biology. This consideration of limitations can guide the use of DAPI spectra in a more informed manner, aligning methodologies with the specific research questions at hand.
Future Prospects in DAPI Research
The domain of DAPI research is ripe with potential, particularly as advancements in technology pave the way for new insights and applications. In today’s scientific landscape, where the visualization of nucleic acids is of paramount importance, understanding the future directions in DAPI research is crucial. It encompasses not only cutting-edge imaging methods but also innovative integrations with complementary techniques that promise enhanced accuracy and efficiency.
Advancements in Imaging Technologies
The field of imaging technologies is evolving faster than a rabbit on a hot stove. New methodologies are coming to light that significantly enhance the resolutions and capabilities of DAPI staining. For instance, super-resolution microscopy, like STED (Stimulated Emission Depletion) and SIM (Structured Illumination Microscopy), is pushing the boundaries of how we visualize nucleic acids. These techniques can detect structures at the nanoscale, empowering researchers to discern unique features within cellular components that were previously unresolvable.
Moreover, platforms that combine DAPI with multi-photon excitation offer deeper tissue penetration, making it easier to visualize samples that are thick or poorly accessible. As these imaging technologies become more refined, they promise not only sharper images but also more reliable quantification of nucleic acids in various contexts, from cellular studies to environmental monitoring.
Advancements in software for image processing are also noteworthy. Tools that leverage artificial intelligence for image analysis can sift through mountains of data, identifying patterns or anomalies that might be missed by the human eye.
- Enhanced imaging offers:
- High-resolution imaging for detailed nucleic acid structure study.
- Deep tissue imaging to analyze complex samples.
- AI-based analysis for efficient data interpretation.
"The sophistication of emerging imaging technologies is a game-changer in biological research, especially when paired with dyes like DAPI to visualize nucleic acids in unprecedented detail."
Integration with Other Techniques
Integrating DAPI with other analytical methods can dramatically broaden its usefulness in various scientific domains. For example, when DAPI is utilized alongside techniques such as CRISPR or fluorescent in situ hybridization (FISH), the insights gained can be multifaceted. DAPI labels the nuclei, providing context, while CRISPR offers precise genetic modifications, shining a light on functional outcomes in specific cellular environments.
Additionally, the coupling of DAPI with RNA sequencing techniques provides a comprehensive understanding of nucleic acid dynamics during cellular processes. This combined approach can elucidate how different RNA populations interact within cells and how these interactions influence overall cellular function.
- Potential integrations include:
- CRISPR for genetic editing studies.
- FISH for simultaneous visualization of nucleic acids.
- RNA sequencing for understanding transcriptomics.
Integration of DAPI with proteomic studies also stands to enrich research by allowing a more holistic view of cellular processes, interpreting how nucleic acids interact with proteins and other cellular structures.
As the field matures, collaborating across disciplines and leveraging the strengths of various techniques will catalyze significant breakthroughs. As these integrations deepen, we can expect DAPI—already a reliable stalwart in the scientific toolkit—to gain newfound versatility and relevance.